Gene symbol
Protein
Extracardiac phenotypes
Reference
Sarcomeric protein
ACTC1
Alpha-cardiac actin
No
[15]
MYH6
Alpha-cardiac myosin heavy chain
No
[16]
MYH7
Beta-myosin heavy chain
No
[17]
Signaling pathway
BRAF
v-raf murine sarcoma viral oncogene homolog B
Noonan syndrome
DHCR7
7-dehydrocholesterol reductase
Smith-Lemli-Opitz syndrome
JAG1
Jagged 1
Alagille syndrome
[23]
KRAS
Kirsten rat sarcoma viral oncogene homolog
Noonan syndrome
NOTCH2
Alagille syndrome
[23]
PTPN11
Protein-tyrosine phosphatase, nonreceptor-type 11
Noonan syndrome
[24]
RIT1
Ras-like without CAAX 1
Noonan syndrome
[25]
SOS1
Son of sevenless homolog 1
Noonan syndrome
[26]
STRA6
Stimulated by retinoic acid 6
Brain, eyes, diaphragm, lung
[27]
Transcription factor
CITED2
Cbp/p300-interacting transactivator, with Glu/Asp-rich carboxy-terminal domain, 2
None reported in human
[28]
FOXC1
Forkhead box C1
Axenfeld-Rieger syndrome
[29]
GATA4
GATA binding protein 4
Diaphragm, gonad, pancreas
[30]
GATA6
GATA binding protein 6
Pancreas
[31]
NKX2–5
NK2 homeobox 5
Pylorus, spleen
[8]
PITX2
Paired-like homeodomain 2
Axenfeld-Rieger syndrome
[32]
SALL1
Spalt-like transcription factor 1
Townes-Brocks syndrome
[33]
SALL4
Spalt-like transcription factor 4
Okihiro/Duane-radial ray syndrome
TBX1
T-box 1
DiGeorge syndrome
[36]
TBX5
T-box 5
Holt-Oram syndrome
TBX20
T-box 20
No
[37]
ZIC3
Zic family member 3
Heterotaxy
[38]
Other gene regulatory mechanisms
BCOR
BCL6 corepressor
Oculofaciocardiodental and Lenz microphthalmia syndrome
CHD7
Chromodomain helicase DNA-binding protein 7
CHARGE syndrome
[41]
CREBBP
CREB binding protein
Rubinstein-Taybi syndrome
EHMT1
Euchromatic histone methyltransferase 1
Brain, craniofacial, urogenital
[44]
KDM6A
Lysine (K)-specific demethylase 6A
Kabuki syndrome
[45]
KMT2D
Lysine (K)-specific methyltransferase 2D (commonly referred to as MLL2, myeloid/lymphoid or mixed-lineage leukemia 2)
Kabuki syndrome
[46]
NSD1
Nuclear receptor binding SET domain protein 1
Sotos syndrome
RBM10
RNA binding motif protein 10
Brain, craniofacial, palate, lung, limb
[49]
STK4
Serine/threonine kinase 4
Immunodeficiency
[50]
Other or unknown function
CYR61
Cysteine-rich, angiogenic inducer, 61
[51]
KIAA0196
Strumpellin
Ritscher-Schinzel/cranio-cerebello-cardiac syndrome
Table 20.2
Chromosomal abnormalities that have been associated with ASD. Candidate genes are suggested by experimental evidence or human mutations that cause congenital heart disease
Syndrome | Candidate genes | Reference |
---|---|---|
2q31.1 deletion | [54] | |
4p16.3 deletion (Wolf-Hirschhorn) | WHSC1, FGFRL1 | [55] |
4q32.2-34.3 deletion | HAND2, TLL1 | [56] |
5p deletion (Cri du chat) | [57] | |
9q subtelomeric deletion | EHMT1 | [44] |
Distal 11q deletion (Jacobsen) | ETS1 | [58] |
Trisomy 13 (Patau) | [59] | |
15q11.2 deletion | [60] | |
16p13.3 deletion (Rubinstein-Taybi) | CREBBP | |
17q23.1-q23.2 deletion | TBX2, TBX4 | [61] |
Trisomy 18 (Edward) | [59] | |
Trisomy 21 (Down) | [62] | |
22q11 deletion (DiGeorge) | TBX1, CRKL, MAPK1 |
Two emerging classes of genetic variation – copy number variations (CNVs) and common single nucleotide polymorphisms (SNPs) – have been associated with ASDs but are not listed in Tables 20.1 and 20.2. CNVs are chromosomal segments that are duplicated or deleted such that there will be more or fewer than two copies of the genes contained within the interval. In general the genes in CNVs that are relevant to their association with congenital heart disease are poorly defined, although a significant fraction contains known or suspected cardiac developmental genes [60, 65]. Two genome-wide association studies (GWAS) of ASD in European and Chinese populations have reported common SNPs associated with ASD risk. The GWAS of European Caucasians detected three SNPs near MSX1 and STX18 on chromosome 4p16 [66]. The 4p13 SNPs were also associated with risk in two different Chinese populations in studies that genotyped just those SNPs [67, 68]. The original GWAS on one of the two Chinese populations did not detect the 4p16 locus; instead, two SNPs near TBX15 at 1p12 and MAML3 at 4q31.1 were reported [69]. None of the genes near the SNPs has a known function in atrial septation, although there are some studies of the role of MSX1 in cardiac development. Much more investigation is necessary to determine the biological meaning of statistically significant CNVs and SNPs.
As a rule, no gene is associated with just one type of defect. Possible exceptions to the rule may offer clues into the mechanisms that lead specifically to ASD. The functional classification of genes in Table 20.1 helps to explain why the rule is generally true. Genes in three of the five categories – signaling pathway, transcription factor, and other mechanisms of gene regulation such as chromatin modification or RNA splicing – affect the expression of many downstream genes, which in turn act in diverse developmental pathways. The specific phenotype expressed depends not only on the causal mutation but also genetic or environmental factors that modify the susceptibility of particular developmental pathways to the mutation [70].
20.2 Secundum ASD Genes
Mutations of some ASD genes are preferentially associated with other classes of defects. For example, mutations of TBX1 are associated with outflow tract defects like truncus arteriosus, tetralogy of Fallot, and interrupted aortic arch, all of which commonly are observed in the 22q11.2 deletion or DiGeorge syndrome [36]. The disease phenotype is consistent with the well-established role of TBX1 in regulating second heart field development at the anterior or arterial pole [71]. Atrial septal development receives contributions from the posterior but not the anterior second heart field [72, 73]. TBX1 is expressed in the entire second heart field [74, 75], so the few cases of ASD may represent a lesser dependence in the posterior second heart field on its activity [76].
Mutations of cardiac transcription factors may be associated preferentially with ASD, but a large fraction of cases involve other simple and complex heart defects. In the cases of NKX2–5 and GATA4, the associations with ASD may be overestimated because of ascertainment bias. Mutations were first described in families in whom more than half and sometimes all the affected members had an ASD, although a few relatives had other types of defects [8, 30]. The incidence of ASD among unselected NKX2–5 or GATA binding protein 4 (GATA4) mutation carriers has not been assessed systematically, but it is probably not as high as in the families who came to attention because of their striking presentations. NKX2–5 mutations are found in only up to 4 % of unselected ASD cases [12, 77, 78]. GATA4 mutations are found in only 1–2 % of unselected ASD cases [78–82]. The incidences of NKX2–5 or GATA4 mutations in other types of defects such as tetralogy of Fallot are similar, which suggests no predilection for ASD [12, 82]. Likewise, pleiotropic heart defects are found in mice that are haploinsufficient for either gene [70, 83, 84].
Mutations of the transcription factor TBX5 cause pleiotropic heart defects as well [13, 85], but a predilection for ASD is well documented. The incidence of ASD ranges from 35 to 60 % in case series of Holt-Oram syndrome patients, which exceeds the incidences of other defects [86–88]. TBX5 genetically interacts with the spalt-like transcription factor 4 (SALL4), the gene involved in Okimoto or Duane-radial ray syndrome [89, 90]. Mutations of SALL4 and SALL1 cause heart and radial limb defects that resemble the Holt-Oram phenotype but without the predilection for ASD [33–35]. Thus, the pathogenesis of ASD in TBX5 mutation may involve a SALL1–SALL4 independent pathway, or SALL1, SALL4, or another gene may serve redundant functions for each other in atrial septal development.
Most genes that cause congenital heart disease sit atop regulatory or signaling hierarchies. Thus, the roles of cardiac alpha-actin (ACTC1 [15, 91, 92]) and alpha- and beta-myosin heavy chains (MYH6 [16, 93, 94] and MYH7 [17], respectively) came as a surprise. Sarcomere mutations have long been known to cause cardiomyopathies that are usually not associated with heart defects. Conversely, the first families discovered to have ASD caused by mutations of ACTC1 or MYH6 showed no evidence for cardiomyopathy [15, 16, 91]. Affected members in subsequent families have hypertrophic cardiomyopathy or ventricular noncompaction that may or may not be associated with a heart defect [17, 92]. No genotype-phenotype correlation or general molecular mechanism explains why a mutation would cause a heart defect, cardiomyopathy, or both. For example, the p.Glu101Lys mutation of ACTC1 can cause hypertrophic, dilated, restrictive, or noncompaction cardiomyopathy or ASD [92].
Unlike most other genes, mutations of ACTC1 and MYH6 seem to be preferentially or exclusively associated with ASD as the phenotypic manifestation of congenital heart disease. No congenital heart defect beside ASD has been diagnosed in large families who have ACTC1 mutations, including the descendants of the family originally described by Zetterqvist [15]. Selection bias seems unlikely in the setting of diagnostic evaluations that span multiple generations up to the modern era [15, 91]. Among the sarcomeric genes, MYH6 mutations appear to be the most common cause of familial ASD [94]. In three studies that identified 54 MYH6 mutation carriers from 12 families, ASD was most common defect (19/54). Certain defects, however, occurred more frequently than might be expected, including tricuspid atresia (2/54), atrioventricular septal defect (2/54), and left-sided obstructive lesions, i.e., aortic or subaortic stenosis or coarctation (7/54) [93–95]. This suggests that the defects share an unknown developmental basis.
Mutations of MYH7 cause ASD, but Ebstein anomaly and left ventricular noncompaction are more prominent developmental phenotypes [17, 96, 97]. Ebstein malformation of the tricuspid valve frequently coexists with an ASD, which is presumed to be secondary to the right-to-left shunt induced by regurgitation from the defective tricuspid valve. This may not always be true, however, as one MYH7 mutation carrier had an ASD without an Ebstein malformation [17]. Independent of the cause, left ventricular noncompaction in children commonly is associated with a wide variety of congenital heart defects [98]. The mechanical function of sarcomeric proteins likely plays dual roles in the formation of the compact myocardium and cardiac morphogenesis [99].
20.3 Sinus Venosus Defect Genes
Only three human genetic mutations have been reported with isolated sinus venosus defects and none with coronary sinus defects. One patient who had a sinus venosus defect with partial anomalous pulmonary venous return had a mutation of CITED2 (Cbp/P300-interacting transactivator, with Glu/Asp-rich carboxy-terminal domain 2) [28]. Partial anomalous pulmonary venous return is a feature of sinus venosus defects. Typically, the right upper pulmonary vein communicates with the superior vena cava but still connects to the left atrium [100]. The interatrial shunt is across the abnormal venovenous communication and not a defect in the atrial septum per se. Genetic mutations that cause total anomalous pulmonary venous return or anomalous connections to form between the systemic and pulmonary venous circulations could explain some cases of sinus venosus defects [101], but none has been described yet.
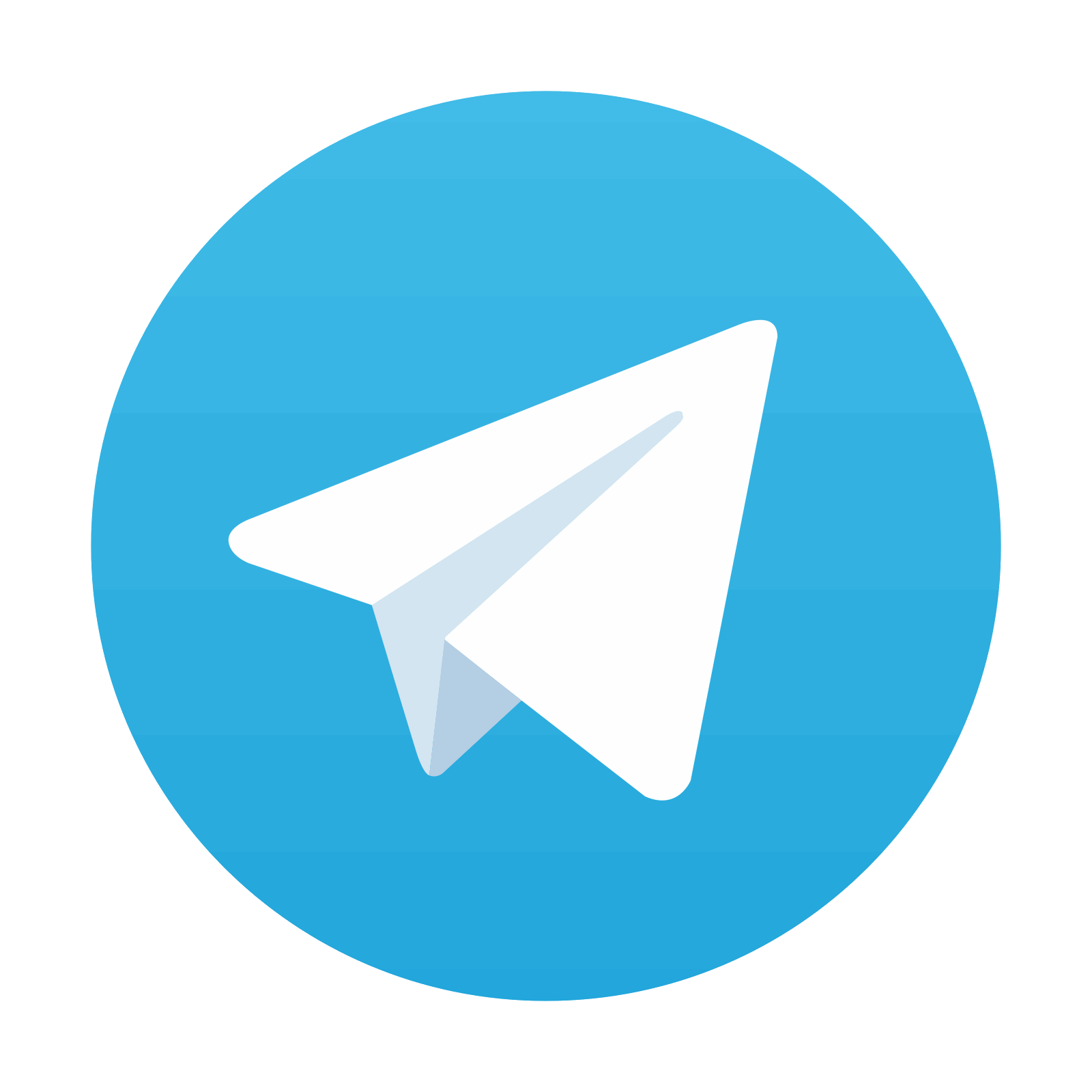
Stay updated, free articles. Join our Telegram channel

Full access? Get Clinical Tree
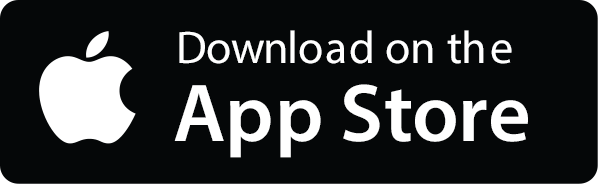
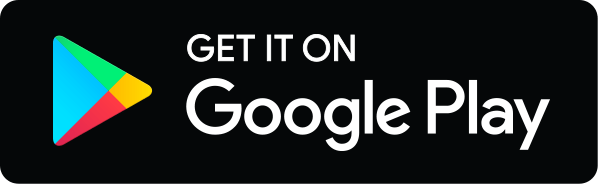