26 Atrial fibrillation (AF) is the most common cardiac arrhythmia in clinical practice. It is well known that AF depends on the interaction between the triggers and substrate.1 It is also well known that the elimination or isolation of the pulmonary veins (PVs) and non-PV triggers can cure AF.1–3 Some patients with paroxysmal AF and nearly all patients with nonparoxysmal AF may require additional substrate modification to improve the clinical outcome. In recent years, growing knowledge of atrial substrate mapping has helped us to learn more about the maintenance of AF and to identify the critical atrial substrate for catheter ablation in spite of the elimination of all triggers initiating AF. However, how to identify the critical arrhythmogenic atrial substrate remained unclear. It is well known that AF is characterized by a spatiotemporal organized activation with a variable frequency and spectral morphology throughout the atria. Therefore, mapping of the atrial substrate would be difficult during a complex rhythm such as AF. The complex activation patterns of AF can result in fractionated signals separated by short and variable intervals that prohibit a reliable identification of the precise local activation rate or CL. Furthermore, it is difficult to represent sustained episodes of AF by only a small sample of fibrillatory EGMs within a limited period of time, and it is technically difficult to link and compare the regional distribution of the AF from time-domain signals alone.4,5 One reasonable method for a transformation is to transform the time-domain EGM signal into a frequency-domain EGM signal, that is, to elucidate the data beyond the time-domain EGM. Frequency-domain signals provide a feasible method to analyze the temporal and spatial distribution of fibrillatory waves during AF.4,5 The regional frequency analysis can identify local sources of AF with rapid repetitive activation and reveal their mechanism. From animal studies to the clinical setting, dominant-frequency (DF) mapping is emerging as a technique for guiding EGM-based approaches to substrate modification. A Fourier transform is based on the concept that the signals can be approximated by the sum of the sinusoidal waveforms with different frequencies. Therefore, the resultant power spectrum is a graph of the DFs that are present within the period of the duration they were collected. The DF of the EGM represents the frequency of the highest power in the spectrum. Assuming an appropriate pretreatment of filtering is applied, the DF is related to the inverse of the average CL. Therefore, the purpose of a frequency analysis is to map the mean activation rate of seemingly chaotic signals during AF. The sites with the highest DFs were considered to be the drivers of AF by analyzing the intracardiac fibrillatory signals throughout the right atrium (RA) and left atrium (LA). The DF calculation is not a flawless process due to the nature of the fibrillatory activities during AF. Various factors, such as preprocessing filtering, QRS-T far field, and the duration of the recording may affect the DF analysis.6 For example, the identification of DF values within the spectra of the physiologically relevant range from 3 to 15 Hz. However, the harmonic peaks of the low frequency range due to the QRS-T complexes may interfere with the atrial DF peaks of interest. The determination of the atrial DF peaks may be inaccurate. This is especially frequently observed with unipolar recording (Figure 26.1).6 Our laboratory routinely applied QRS-T subtraction as signal preprocessing before spectral analysis (Figure 26.2). In our experience, QRS subtraction is required for frequency analysis of fibrillation signals if the recording sites show low atrial voltage or prominent far-field ventricular potentials. The QRS subtraction techniques improve the reliability of the frequency spectra obtained from intracardiac AF signals. Figure 26.1 Schematic presentation of frequency analysis from time-domain signals. Figure 26.2 An example of frequency analysis before and after QRS subtraction. The frequency peaks (right upper) obtained from frequency analysis without QRS subtraction are difficult to analyze because of multiple harmonic peaks of QRS-T wave signals. On the other hand, the frequency analysis showed single and dominant peak if the signals were processed after QRS subtraction. These results indicate that QRS subtraction before frequency analysis is mandatory in this ventricular far-field dominant atrial signals. Reliability of the DF sites, spatially and temporally, is an important issue for the application of clinical DF mapping. The reproducibility of DF values over time remains a controversial issue. Recently, Habel et al used a stationary 64-electrode basket catheter in the LA to record the atrial activity over 5 minutes. They reported that inaccuracy occurred during repeated measurements of the DF value. These data raised more questions about the accuracy of DF mapping by the sequential site-by-site approach. However, the investigation from other laboratories showed the opposite results.7,8 Sander et al showed the temporal stability of the DF values in patients with AF presenting for ablation, and the stability of the DF improved after a recording duration of > 5 seconds.7,8 Verma et al examined the stability of fractionated EGMs in 24 patients with AF. There was no significance of a mean interval of < 120 ms (> 8.3 Hz), as compared to sites with a mean interval of > 120 ms.9 Lin et al further demonstrated that only the sites with the shortest fractionated interval harbored the least temporal variation.10 These data suggest the temporal stability in the highest DF region, and a temporal variation of the DF may be observed in the rest of the atrium. Thus, DF mapping with simultaneous multiple electrode catheters or Ensite-array DF mapping may be alternatives to improve the accuracy of the DF (Figures 26.3 and 26.4; Figure 26.3 A schematic example demonstrating the global frequency analysis in the atrial chamber, the overall power spectrum, and the 2-dimensional (2D) display of the DF value distribution. The 256 noncontact unipolar EGMs of each chamber were exported for a dominant frequency analysis (6.8 seconds, 1200 Hz, using surface ECG lead V1 as the template to subtract the QRS-T far-field signal, and the 2D DF map was performed using SPSS software version 11.0). Figure 26.4 Activation map, frequency map of the LA, and the unipolar EGMs in a patient with paroxysmal AF from RSPV. The high-DF site was near the RSPV ostium, where repetitive rS unipolar EGMs were observed near the origin of RSPV triggers. In most of the laboratories, DF value was calculated during AF. An alternative approach is to use the spectral analysis to characterize the sinus EGMs based on the consistency of the sinus EGMs over time. Previous studies showed that abnormal atrial sites were characterized by fractionated EGMs as shown in the time-domain EGMs and high-frequency peaks in the frequency domain analysis during sinus rhythm.11–15 According to Pachon’s study,11 the high-DF sites (or AF nest) were identified by visual inspection of the frequency spectra, especially in the high-frequency range of > 80 Hz. This laboratory12 applied a 30-Hz high-pass filtering to exclude the fundamental peaks to highlight the corresponding high DF sites of the abnormal atrial substrate in Pachon’s study. Technically, the spectral analysis of sinus EGMs was based on bipolar EGMs (Figure 26.5). A low-frequency resolution (> 0.5 Hz) owing to a short recording duration of the sinus EGMs makes a sophisticated quantitative analysis of the DF value of a single site less likely. The advantage of AF nest analysis is the reliability and consistency of the EGM during sinus rhythm. Figure 26.5 Illustration of the frequency analysis during AF and spectral analysis during sinus rhythm (AF nest). (The data were reproduced from reference 12, with the permission of the publisher.) The application of DF mapping in the substrate mapping and ablation is summarized in Table 26.1. Current evidence has demonstrated that DF mapping could be used to (1) classify the atrial substrate, (2) locate the abnormal arrhythmogenic regions, and (3) as an endpoint for substrate modification. Prospective DF-guided ablation was mostly performed in paroxysmal AF patients.11,13,14 The application of a DF-guided ablation in nonparoxysmal AF patients remains limited in the literature. In the majority of patients with paroxysmal AF, there is an LA-to-RA DF gradient; this is compatible with the animal model of acute AF, in which AF waves emanating from the high-frequency sources of the LAPV resulted in an LA-to-RA DF gradient, suggesting that the LAPV area is important for maintaining sustained AF. However, in patients with AF originating from SVC,15 the highest-DF sites were located inside the arrhythmogenic SVC to the SVC-ostium, and no LA-to-RA DF gradient was observed (Figures 26.6 and 26.7). Recently, Suenari et al used 3D high-density DF mapping for paroxysmal AF prior to the PV isolation. High DFs (> 8 Hz) were identified in 1.5 ± 0.9 regions per patient and were mostly located within 15 mm of the PV ostia. Of those, 75% of the high DF regions were related to arrhythmogenic veins. There was a significant steep DF gradient from the arrhythmogenic PV ostium to the left atrium. Therefore, the DF valve could be used to identify the potential thoracic vein triggers during AF. Figure 26.6 Multisite bipolar recordings and frequency analysis in the patients with paroxysmal AF originating from the SVC. A DF of 10 Hz was found inside the SVC. The DFs of the RA sites were lower, ranging from 5.67 to 6.1 Hz. There was a frequency gradient from the arrhythmogenic SVC to the rest of the atria and other thoracic veins. The frequency gradient of LA to RA was not evident in this patient. (The data were reproduced from reference 15, with the permission of the publisher.) Figure 26.7 Panel A: In the patients with SVC-AF, the DF was the highest inside the SVC or SVC ostium, with a significant frequency gradient to the RA, LA, PV, and CS. Panel B: In the PV-AF patients, the DF of the PV ostium was higher than the DF of the LA (near the PV ostium), and the DF of the LA was significantly higher than that of the RA. The lowest DFs were located in the CS, RA, and SVC, which were far away from the highest DF and site of the ectopy initiating AF. (The data were reproduced from reference 15, with the permission of the publisher.)
How to Utilize Frequency Analysis to Aid in Atrial Fibrillation Ablation
Yenn-Jiang Lin, MD, PhD; Men-Tzung Lo, PhD; Li-Wei Lo, MD; Shih-Ann Chen, MD
Introduction
Preprocedural Planning: Technological Considerations
Requirement of QRS-T Subtractions
Reliability and Stability of DF Value
Video 26.1). However, these limitations do not preclude the application of sequential DF mapping, because only the high-DF regions are of interest during AF in the electrophysiologic mapping and ablation procedure.
Spectral Analysis During Sinus Rhythm: AF Nest Identification
Procedure
DF Mapping in Paroxysmal AF Patients
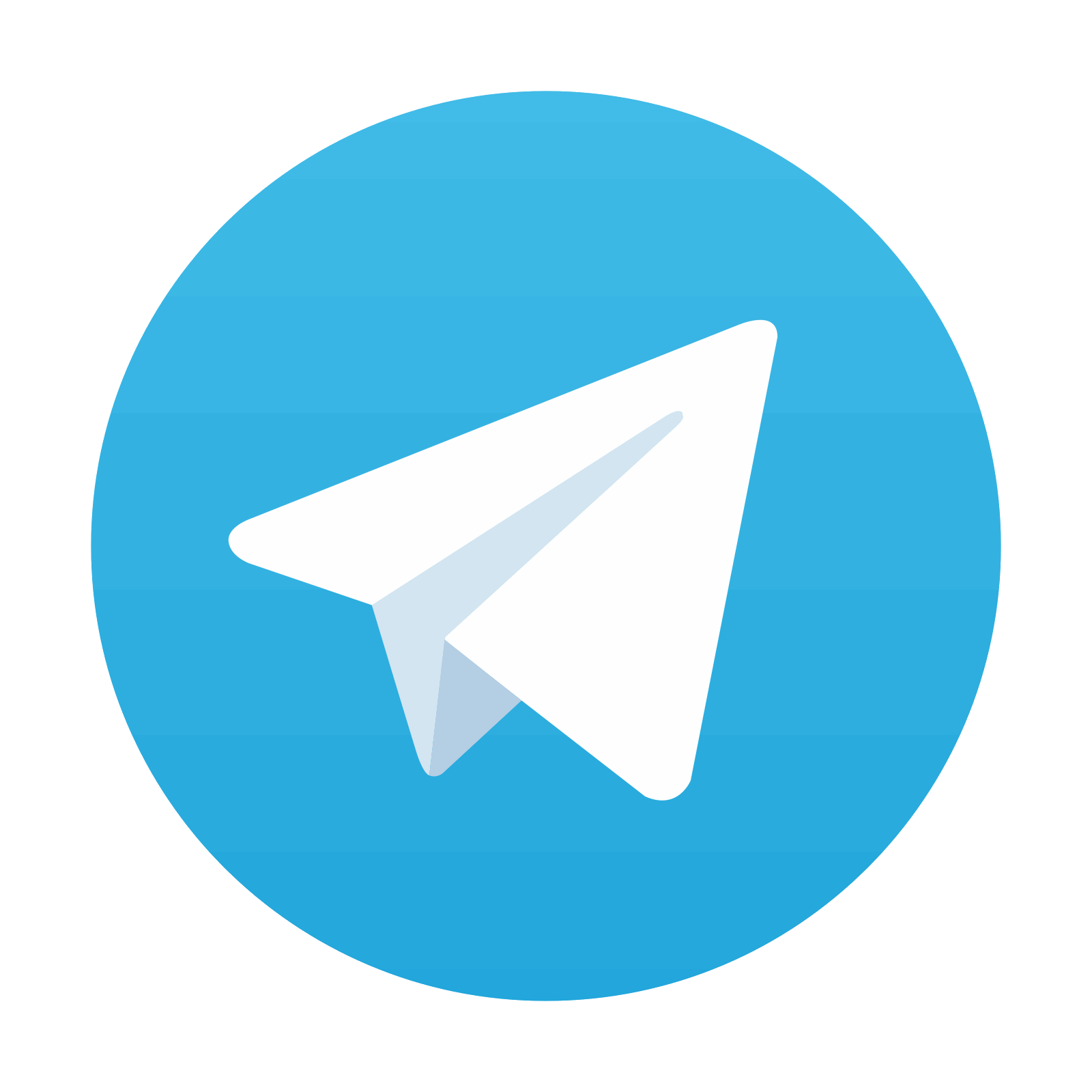
Stay updated, free articles. Join our Telegram channel

Full access? Get Clinical Tree
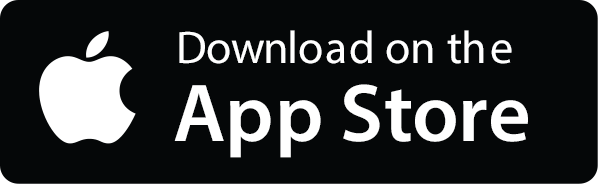
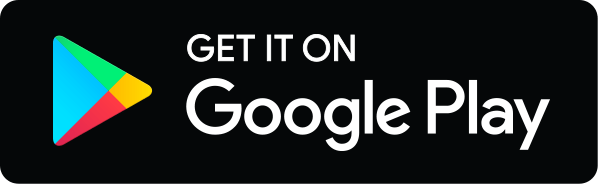