19
How to Use the Radiofrequency Hot Balloon for Ablation of Atrial Fibrillation
David E. Haines, MD
Introduction
Catheter ablation of atrial fibrillation (AF) has proved to be a valuable modality in the management of symptomatic patients. The mainstay of AF ablation remains pulmonary vein (PV) antral isolation.1 It has been the repeated observation that triggers for initiation of AF most often arise from the muscular sleeve that extends up the PVs.2,3 Since the focal origin of AF from PVs was initially described, these veins have been the primary target for ablation. Initial attempts at direct ablation of the triggering foci were limited by the intermittent nature of spontaneous firing and the substantial anisotropy of conduction within the PV wall, making conventional activation timing extremely challenging. The focal ablation approach was soon supplanted by segmental PV ablation, in which a quadrant of fibers extending from the ostium into the vein were ablated, thus rendering inactive the communicating tissues distal to the point of ablation.4 Procedural success continued to improve as ablation stepped out to the PV ostia. Eventually, 4-vein ostial PV isolation became a standard of care.5,6 A significant limitation of ostial PV ablation has been PV stenosis, reported in up to 7% of cases in the literature of that area.7 It was also observed that triggering foci sometimes arose from the transition tissue bordering the PV ostium and PV antrum. For these reasons, PV antral ablation or wide-area circumferential ablation is now the standard approach.8–10 This avoids the potential for PV stenosis and incorporates a larger region of potentially arrhythmogenic tissue.
Despite the appeal of this approach, point-by-point ablation of the PV antra can be laborious and time consuming. In addition, a long ablation line is required to complete the extraostial circle. Any recovery of function anywhere along the entire line can result in breakout potentials from PV triggers and lead to recurrence of the clinical arrhythmia.11 Therefore, there was a strong impetus to develop effective tools to rapidly and reliably isolate the PVs. Balloon ablation technology has an obvious appeal. When collapsed, the balloon can be inserted into a relatively small introducer sheath to gain access to the left atrium (LA). Once in position, the balloon can be inflated to a size large enough to cover the entire extraostial antral zone. If ablation of contiguous tissue can be reliably achieved, PV isolation may be achievable with a single catheter placement. The challenge, then, was how to best achieve reliable and safe transmural ablation circumferentially along the perimeter where the balloon opposed the target tissue.
Radiofrequency Hot Balloon Design
The design characteristics of an ideal balloon ablation catheter include both a very low profile for insertion as well as the capability for a very large inflated dimension to allow ablation of larger veins and vein antra. In order to allow one balloon to be sized to veins of varying diameters, the balloon material should be pliable, so that it may be inflated to different sizes. The balloon should also be compliant so it can conform to variable anatomies, including oval, flat, or funnel-shaped vein ostia. Although a highly compliant balloon is ideal, this characteristic of balloon material must be balanced with tear resistance and overall durability so that a single catheter can be repositioned, removed, and reinserted multiple times throughout the case without developing leaks.
The radiofrequency hot balloon (TSB, Toray Industries, Inc., Tokyo, Japan) is fashioned from a very elastic polyurethane that is 20 µm in thickness. The central shaft of the catheter has a guidewire lumen for vein anchoring and distal infusion and can be advanced through the outer catheter body in order to stretch the balloon longitudinally to reduce its profile and facilitate passage into the introducer sheath. When the balloon is deployed in the heart, the central shaft may be retracted, allowing for a rounder balloon profile after inflation. There is a second lumen with a large cross-sectional area communicating between the balloon inflation port on the catheter handle and the balloon lumen. This lumen allows relatively unrestricted flow from the agitator pump to the balloon lumen for mixing during energy delivery (Figure 19.1).
Figure 19.1 Schematic representation of the radiofrequency hot balloon catheter.
The balloon is inflated with a solution of normal saline and ionized contrast medium (usually 1:1) so the viscosity is low but the balloon can still be easily visualized on fluoroscopy. Since radiofrequency (RF) energy is delivered within the balloon, it is important that the liquid in the balloon has reasonable electrical conductivity. The RF element, an electrical coil, is mounted to the inner shaft within the balloon along with a thermocouple for temperature monitoring. Energy is delivered between the RF element and a pair of dispersive ground pads placed on the patient’s skin. At least 2 ground pads are needed because high powers are delivered to the RF hot balloon catheter, and use of a single dispersive electrode could cause skin burns. Maximum delivered power is 150 W at a frequency of 1.8 GHz. Even though the balloon material is electrically insulating, there is capacitive coupling of charges inside and outside of the balloon, which allows the transmission of the electrical energy through the balloon into the surrounding medium. The RF element causes resistive heating of the solution within the balloon, and temperature feedback power control modulates power delivery to maintain the preset temperature chosen by the operator. One of the major challenges to overcome with a RF hot balloon ablation system was the tendency for the heated saline to rise superiorly in the balloon, leaving cooler saline in the inferior hemisphere. In order to realize uniform circumferential heat delivery to the balloon surface, mixing of the saline in the balloon lumen is required. The TSB ablation system design accomplished this by using an external agitator pump. A tube is connected from the balloon inflation port through a 3-way stopcock to a modified circular roller pump on the RF generator. When engaged, the roller pump agitates the solution within the balloon with oscillating pressure waves transmitted through the balloon inflation lumen of the catheter. This results in mixing of the solution within the balloon and uniform temperature distribution (Figure 19.2).
Figure 19.2 Schematic diagram of hot balloon catheter with superimposed finite element analysis of temperature distribution within the balloon. The saline/contrast mixture in the inflated balloon has a relatively uniform temperature due to the mixing action of the oscillating pump. The balloon surface that contacts the tissue conducts heat passively transmurally through the atrial wall. (Reproduced with permission from Evonich RF et al.15)
Mechanism of Radiofrequency Hot Balloon Ablation
Like many forms of catheter ablation, the mechanism of tissue injury from RF hot balloon ablation is thermal. The electrode within the balloon heats the saline/contrast mixture in the balloon which, in turn, heats the outer surface of the balloon. Wherever the balloon surfaces come in contact with tissue, that heat is conducted into that tissue. If the tissue is heated above the temperature of irreversible injury (approximately 50°C),12 then there will be tissue necrosis. With all ablation technologies, the challenge is how to produce transmural lesions reliably without causing significant injury to collateral structures. In particular, esophageal injury with risk of atrial esophageal fistula is one of the greatest concerns from this procedure. Although the incidence is very low, the case fatality rate exceeds 50%.13 With all other hyperthermic technologies, there are varying degrees of direct tissue heating (volume heating). For example, with RF catheter ablation, there is direct electrical heating below the endocardial surface. When combined with convective cooling at the tissue surface, highest temperature may be reached 2 to 4 mm below the endocardial surface.14 The temperature measured on the endocardium by the catheter temperature sensor markedly underestimates the peak tissue temperature. As a consequence, it is very difficult to know how deep the lesion is that is being created. Thus, the operator may have minimal heating at the tissue surface on the posterior LA wall during RF catheter ablation, but may be getting substantial heating of the underlying esophagus. In contrast, ablation from the RF hot balloon ablation catheter is purely thermal. The only measurable volume heating occurs entirely within the confines of the balloon. All heating that occurs in tissues in contact with the balloon surface is strictly conductive heating. Thus, the maximum tissue temperature during any given ablation is that which is measured on the balloon and endocardial surfaces. All temperatures below the endocardial surface are lower than those on the surface; tissue temperature falls in a predictable fashion inversely proportional to the distance from the thermal source. It is impossible to get excess heating to deeper structures if balloon temperature is limited.
Preclinical studies demonstrated relatively uniform temperature of the saline/contrast mixture within the balloon during heating using the oscillating mixing pump; however, the surface temperature relative to the peak temperature near the electrode is predictably lower. The temperature of the tissue is predicted by the temperature selected during catheter ablation as demonstrated in a preclinical study (Table 19.1).15 In no case was the measured epicardial temperature higher than the endocardial temperature. Larger balloon diameters result in a greater temperature differential between measured central temperature and balloon surface temperature. Thus, when the balloon is inflated to a larger size to match the size of a larger antrum, higher powers and higher temperature presets must be used. Lesion formation was achieved reliably when high preset temperatures (90°C) were used, and lesion formation was more reliably achieved with a 180-second duration of power delivery. When the RF hot balloon was tested in vivo in a porcine model, PV isolation was achieved in 83% of the veins attempted. The veins in which electrical isolation was achieved showed macroscopic evidence of complete circumferential ablation at necropsy. Histological examination showed findings consistent with transmural thermal injury of the PV and antral myocardium (Figure 19.3).16
Central Balloon Temperature | 60°C | 70°C | 80°C |
Endocardial temperature | 46 ± 6°C | 53 ± 8°C | 57 ± 11°C |
Epicardial temperature | 43 ± 5°C | 50 ± 5°C | 57 ± 11°C |
Power | 50 ± 13 W | 75 ± 24 W | 92 ± 15 W |
Figure 19.3 Panel A: Low-power longitudinal section of right inferior PV showing transmural thermal injury, characterized by fibro-collagenous changes (staining blue by Masson’s stain). Panel B: Higher magnification of the boxed area in Panel A, showing focal calcification of giant cells and macrophages (eosinophilic staining by hematoxylin & eosin). Panel C: Low-power longitudinal section of left inferior PV showing transmural thermal injury, characterized by fibro-collagenous changes (staining blue by Masson), and focal area of organizing granulation tissue with mild chronic inflammation consisting of macrophages and few giant cells, indicated by the small arrow in Panel D.
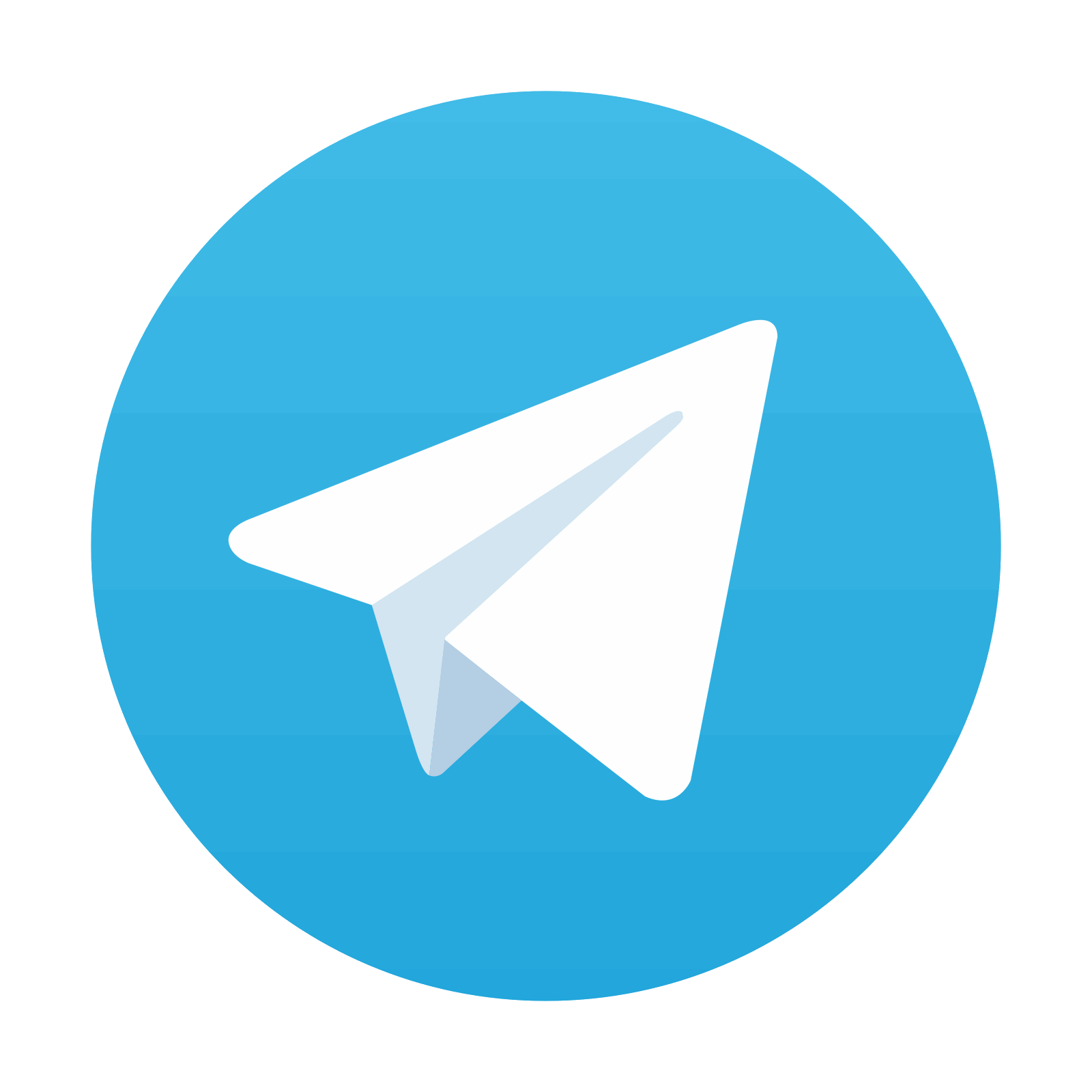
Stay updated, free articles. Join our Telegram channel

Full access? Get Clinical Tree
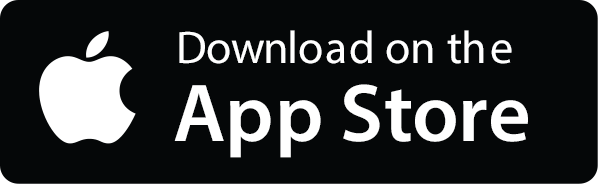
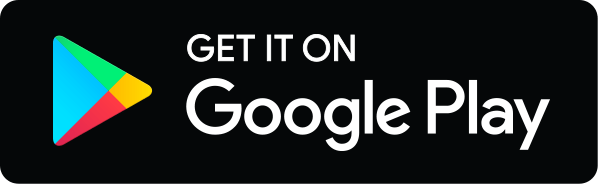