30 Catheter ablation for cardiac arrhythmias, including AF, has been facilitated in recent years by the development of new technologies allowing precise localization of mapping and ablation catheters in relation to the relevant cardiac anatomy. Electroanatomical mapping, in which 3-dimensional (3D) anatomical information is combined with local electrical information during real-time catheter mapping, can create 3D representations of cardiac structures and associated electrical function. However, even with extensive and high-density mapping, the anatomic information provided by this technique remains inferior to that provided by imaging modalities such as cardiac MRI and CT. This is due in part to the complex anatomy of the PV and the LA. There are thin ridges separating the PVs from each other and from structures such as the LAA. As a result, navigating the catheter in the LA can sometimes be challenging and the availability of a detailed representation of the LA anatomy at the time of the procedure is important to ensure a safe and effective outcome. Image integration has been developed with the aim of incorporating the detailed anatomy of the LA into the operative field. By allowing the fusion of CT and MRI acquired prior to the procedure with a catheter localization tool, this technology provides the operator with a 3D view of the heart with the catheter position superimposed in an anatomically accurate manner.1 Currently, the available image integration algorithms can be used with magnetic and impedance-based electroanatomic mapping systems. In addition to CT and MRI, anatomy of the LA can also be acquired using 3D rotational angiography, which in turn can be integrated with a catheter-localization tool. Image integration allows better visualization of the location of the ablation catheter in relation to critical LA structures such as the PV ostia and the LAA ridge. Observational and prospective randomized studies have demonstrated that image integration improves the procedural outcome by reducing fluoroscopy time and improving the success rate.2–6 However, in order to fulfill these promises, image integration must be accurate. One major limitation of this approach is that is that the CT and MRI are not obtained in real time coincident with the catheter mapping, and any error in integration will translate into error in identification of catheter position in relation to the superimposed images. Accurate integration is therefore necessary for precise catheter localization relative to the imported MRI and CT images in order to ensure maximum safety and efficacy during ablation. In this chapter, some techniques that may improve the accuracy of image integration are discussed. Before performing imaging studies, the patient’s medical history should be investigated in order to rule out the presence of contrast medium allergies or devices which are not compatible with MRI (i.e., older generation cardiac devices or metallic implants). It is -dvisable to test blood hCG levels in all premenopausal women undergoing CT scan. Renal function must be assessed because of the risk of iodine contrast medium-induced nephropathy or gadolinium-induced nephrogenic systemic fibrosis. Claustrophobia may also be an issue, especially in patients undergoing MRI study. Both cardiac CT and MRI are used to obtain images to be integrated with electroanatomical maps, and they seem to provide comparable image quality and information, thus making the choice largely determined by an institution’s expertise and availability. MRI avoids ionizing radiation exposure and the use of iodine-based contrast media, which may carry harmful effects for the patients. However, it has been suggested that serious clinical consequences in patients with significantly impaired renal function may be caused by gadolinium as well, even though evidence in this matter is not conclusive. Furthermore, MRI equipment is not as widely available as CT equipment is. Finally, patients’ tolerance to MRI is poor, primarily due to the duration and confinement associated with the exam. Changes in hemodynamic status between the time of imaging and catheter ablation have the potential to influence registration accuracy. In order to minimize differences between the geometry at the time of image acquisition and the ablation procedure, the intervening period should be reduced as much as possible. The helical scanning of multidetector tomographs enables acquisition of images within a breath hold. In more evolved tomographs, more series of detectors are present, which further reduces procedural time. This allows acquiring simultaneously data relative to large volumes. For cardiac studies, equipment must be furnished with at least 16 series of detectors. Recently, the introduction of wide-area detectors or dynamic volume scanners, such as the 320-row multidetector CT scanner (Aquilion One, Toshiba, Otawara, Japan) provides full cardiac coverage enabling whole-heart imaging without helical scanning. This new technology may reduce not only radiation dose to less than one-fourth of that for a typical multidetector CT scan but also the total amount of contrast material needed for ECG-gated scanning. The “ECG-pulsing technique,” in which the tube current is modulated between 40% and 80% of the R-to-R interval, has the potential to reduce by 80% the effective radiation dose. A bolus of iodinated contrast is injected intravenously, followed by a saline chaser. The LA and PVs anatomy can also be studied using gadolinium-enhanced MRI. Such studies are performed on 1.5- to 3-Tesla MRI systems during a breath-hold in the postabsorptive state. Angio-MRI is usually obtained with a 3D fast-field Spoiled Gradient Echo imaging sequence. Slices with a thickness of about 1.5 mm are acquired. The acquisition is performed after the injection of paramagnetic contrast medium. Revelation systems which can synchronize the acquisition of the image at the exact time when the bolus passes the LA are used in order to reduce the exposure time. Intraprocedural acquisition of LA volumes using rotational angiography has recently been introduced.7,8 After contrast medium injection in the right heart chambers, the fluoroscopy c-arm is rapidly rotated around the patient, and images are acquired throughout the rotation to generate 3D volumetric anatomical rendering of the LA-PVs. Such images can be superimposed to the fluoroscopic projections of the heart or integrated into an electroanatomical mapping system. This innovative technique might overcome the limitation of acquiring the images in a different time with respect to the ablation procedure, but the consistent iodinated contrast agent load and radiation dose are important limiting factors. Once the image is acquired, it is stored in a proprietary format. The data can then be exported from the scanner. A medical image standard known as DICOM (Digital Information and Communications in Medicine) has been devised and is widely used. This allows data to be exchanged between different scanners and viewing consoles. After performing the CT/MRI studies, the images are imported into the electroanatomic mapping system using customized softwares (CARTOMERGE, Biosense Webster, Diamond Bar, CA; NavX Fusion/Verismo, St. Jude Medical, St. Paul, MN; Rhythmia, Boston Scientific, Cambridge, MA). The process of isolating the structures of interest from the remainder of the radiological image is called segmentation. First, the intensity threshold is set by selecting a transverse slice at the level of the LA, which is the chamber of interest where contrast density should be maximized. Pixels with intensities below a threshold value are assigned one class and the remaining pixels a different class. Regions are then formed by connecting adjacent pixels of the same class. After that, labels or “seeds” are placed in the center of each anatomical structure to optimize separation of the chamber of interest. When any structures are not adequately separated by the semi-automatic software, the image can be manually edited using the “slice and punch” tools to differentiate the structures. The PVs are often cut at a more proximal level, where an excessive branching does not create problems in distinguishing vascular structures from each other. Since ablation deep inside the PVs must be avoided, excluding their distal portion does not negatively affect the ablation procedure. Once the 3D reconstruction of the LA-PVs block has been separated from the surrounding structures, it can be exported into the real-time mapping system for registration. Before performing image integration itself, the 3D anatomic shell of the LA must be created using an electroanatomical system. Two such systems were the only ones currently available until recently: the magnetic-based CARTO System (Biosense Webster), and the impedance-based EnSite NavX system (St. Jude Medical). The latest versions of both systems use contact mapping for chamber geometry reconstruction by sweeping the catheter tip along the endocardial surface of the chamber of interest, which allows for quicker acquisition of a large number of points and a better definition of anatomy, especially when using multielectrode catheters. Adequate catheter contact while acquiring surface points is determined by fluoroscopic visualization of catheter mobility in relation to cardiac motion, a discrete atrial EGM, the catheter icon on the 3D navigation system, and, when available, contact force sensing technologies. Uniform point sampling is essential to avoid geometry distortion. Regions of the LA susceptible to deformation (roof, anterior wall, appendage) should be approached carefully (Figure 30.1). Figure 30.1 Accurate image registration. Posterior view of the LA showing an integrated MRI image. The ablation catheter (black arrow) is advanced into a small branch of the right inferior pulmonary vein without protruding outside the MRI shell. The white points along the roof (white arrowhead) protrude outside the shell which is the result of distention of the roof during map acquisition.
How to Perform Accurate Image Registration with Electroanatomic Mapping Systems
Francesco Perna, MD, PhD; Moussa Mansour, MD
Introduction
Preprocedural Planning
Patient Preparation
Imaging
CT Scan
MRI
Rotational Angiography
Procedure
Image Segmentation
3D Geometry Creation
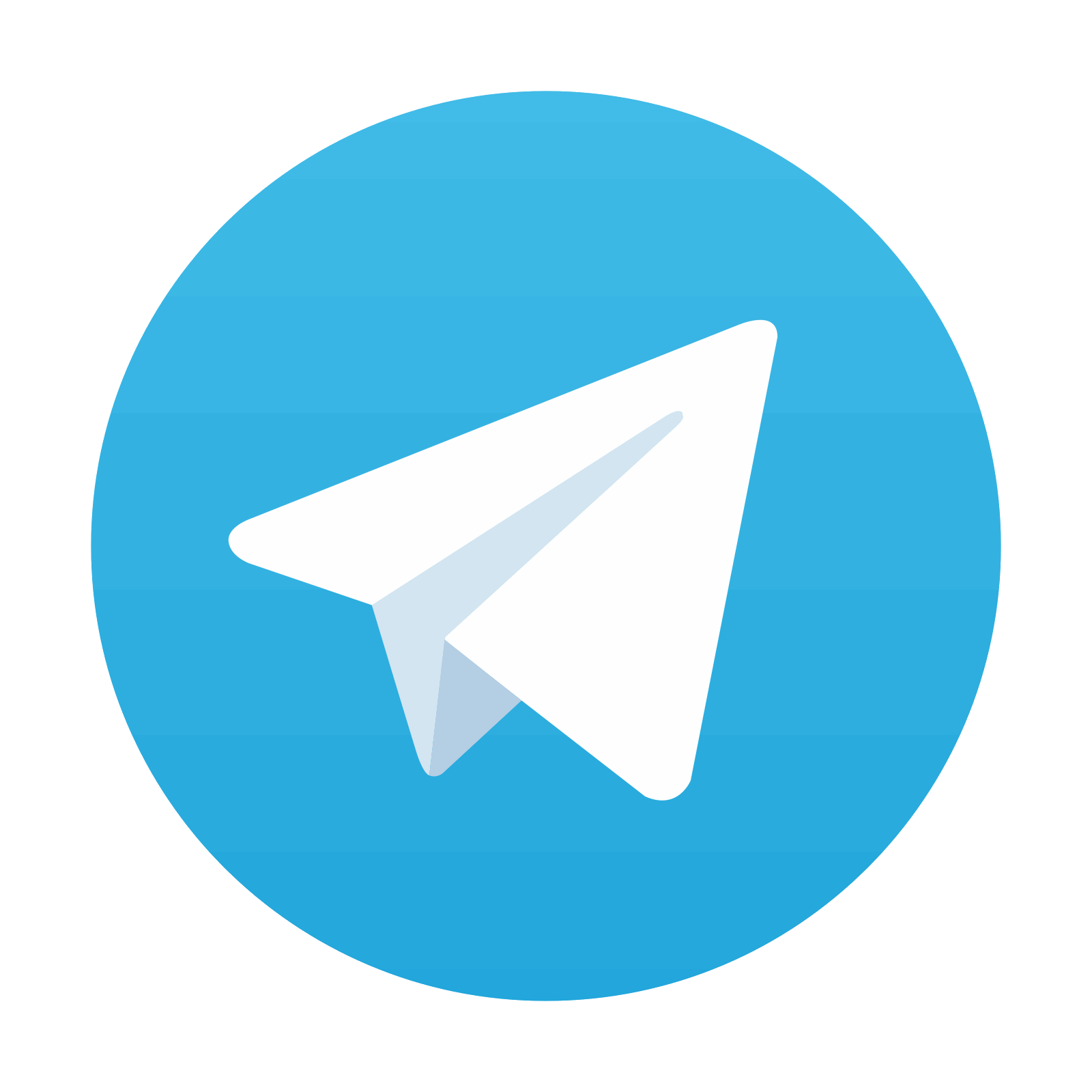
Stay updated, free articles. Join our Telegram channel

Full access? Get Clinical Tree
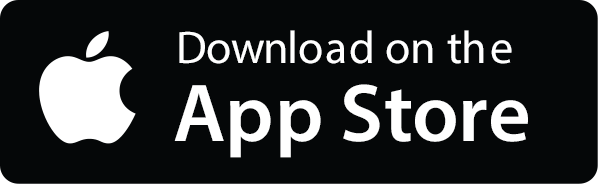
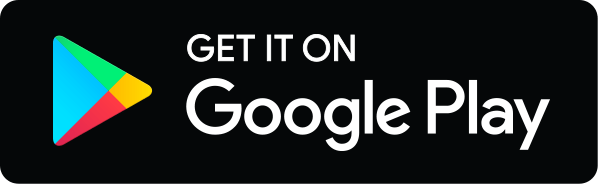