39 Sustained ventricular tachycardia (VT) is an important cause of morbidity and mortality in patients with structural heart disease. Although implantable cardioverter defibrillators (ICDs) are effective in terminating VTs and reduce the risk of arrhythmic death,1,2 ICD shocks, particularly when recurrent, have been associated with an increased in mortality3–5 as well as causing significant decreases in patients’ quality of life.6,7 Additional intervention is needed when VT becomes recurrent or incessant. Antiarrhythmic drugs are sometimes useful but are associated with cardiac and noncardiac toxicities, and their long-term efficacy is limited.8 Catheter ablation offers an alternative therapy for controlling ventricular arrhythmia recurrences, especially in patients with hemodynamically tolerated VTs.9–11 Patients for VT ablation should undergo a detailed cardiovascular evaluation prior to the procedure. Tachycardia mechanisms and origins usually depend on the patient’s underlying heart disease, which should be characterized. Much of what we know was based on ventricular arrhythmias associated with ischemic heart disease. In patients with ischemic heart disease, the reentrant VT circuits are often located at the subendocardial surface near the infarct region, usually confined to a specific coronary vascular territory.12,13 The abnormal subendocardial scar gives rise to slow, nonuniform, anisotropic conduction and low-amplitude, fractionated electrograms (EGMs) recorded at the infarct border zone, as well as constituting the substrate for scar-based reentry.13–15 In contrast to patients with postinfarction ventricular arrhythmias, the majority of patients with nonischemic cardiomyopathy (NICM) had only a modest area (< 25%) of endocardial low-voltage abnormality that was located near the ventricular base in the perivalvular region.16 Recent studies in patients with NICM demonstrated 2 predominant scar patterns—basal anteroseptal versus inferolateral—of nearly equal distribution.17,18 Delayed-enhancement magnetic resonance imaging (MRI) and electroanatomic mapping (EAM) have demonstrated a predominance of mid-myocardial and epicardial scar, with larger confluent epicardial low-voltage areas compared to the endocardial surface.19–23 Consistent with pathologic findings, less dense scar (< 0.5 mV) was observed in patients with nonischemic compared to ischemic cardiomyopathies, and low amplitude late potentials reflecting conduction delay is significantly less prevalent. A late potential-guided ablation strategy was associated with less favorable outcomes in patients with NICM compared to postinfarct patients.24 In patients with arrhythmogenic right ventricular cardiomyopathy/dysplasia (ARVC/D), sizable low-voltage areas may involve the infundibulum, free wall, and basal perivalvular regions that constitute the endocardial VT substrate in this disease.25–27 Progressive fibro-fatty replacement and the presence of extensive epicardial scars have recently identified,28 with the reentrant VT circuits predominantly located at the epicardial surface. In patients with congenital heart disease with prior surgical correction, the reentrant circuits are often related to the procedural incisions and surgical reconstructions.29 A prior history of open-chest coronary bypass grafting or valvular surgery is important if a percutaneous epicardial approach is being considered,30 whereas the presence of significant peripheral vascular disease, intracavitary thrombus, or prior mechanical aortic or mitral valvular prostheses precludes a retrograde aortic or transseptal approach for endocardial intervention. In patients with structural heart disease, the presence of a monomorphic VT usually denotes a relatively “fixed” arrhythmia substrate, commonly related to scar-based reentry, although it is important to qualify that patients with structural heart disease occasionally have idiopathic VTs. Although myocardial ischemia and acute infarction alone are rare causes of monomorphic VTs, the potential of ischemia should always be assessed, especially in patients with coronary artery disease and prior myocardial infarction. The severity of cardiac dysfunction and the locations of wall-motion abnormalities should be evaluated by a transthoracic echocardiogram before VT ablation. The presence of wall motion abnormalities or thinning suggests areas of scar and potential locations for the arrhythmogenic substrate. The presence of a mobile intracavitary thrombus is a contraindication for endocardial mapping procedures, particularly in patients with severe ventricular dysfunction or LV aneurysm. MRI with delayed gadolinium enhancement (DE-MRI) is increasingly recognized as an important imaging adjunct for identification of myocardial scar and potential arrhythmia origin in patients with structural heart disease.20,23 Infarct tissue heterogeneity on MRI is a strong predictor of spontaneous ventricular arrhythmia occurrences and have been correlated to the critical sites of ischemic VT.31,32 Furthermore, VT-related conducting channels, detected by EAM, can also be identified by contrast-enhanced MRI.33–35 Alternatively, regional myocardial wall thinning detected on multislice-computed tomography (MSCT) images correlate to low-voltage scars and distribution of local delayed potentials in postinfarction VTs.36 The scar locations (endocardial, epicardial, or intramural) on preprocedural imaging facilitates procedural planning and workflow and may reduce procedural time. The integration of MRI or CT images with 3D EAMs helps to focus mapping and ablation on the culprit regions. Careful review of the ECG is a critical step of the preprocedural planning. Focusing on VT initiation, termination, and cycle length oscillations, insights into the arrhythmia mechanisms (macroreentry or focal) and sites of origin of VT can be obtained. A “warm-up” phenomenon with significant cycle length oscillation (≥ 20 to 30 ms) suggests a focal, automatic mechanism, whereas an abrupt initiation or termination with PVC implies reentry. The presence of Q-waves during sinus rhythm may reveal the locations of myocardial scars and the potential arrhythmia substrates. The presence of a bundle branch block suggests the presence of a diseased conduction system that may participate in a reentrant arrhythmia using the Purkinje fibers or bundle branch reentry. If the 12-lead ECG during VT is available for review, the QRS morphology of VT or PVC reflects the earliest site of ventricular activation, and the VT site of origin (reentry exit site or focal early activation) can be regionalized with a reasonable accuracy.37 Some general rules can be applied to the ECG analysis.37 We focus on (1) the bundle branch block configurations in lead V1, (2) the frontal QRS axis in lead I, (3) the inferior lead (II, III, aVF) QRS axis, and (4) patterns of precordial R-wave transition. VT with a left bundle branch block (LBBB with lead V1 being predominately negative) configuration and a positive R wave in lead I (leftward axis) suggests the ventricular arrhythmia originates at the interventricular septum or the right ventricle (RV). Right bundle branch block (RBBB, with lead V1 being predominately positive) configuration and a negative QRS in lead I (rightward axis) suggests an arrhythmia site of origin located near the free wall of left ventricle (LV). Positive in the inferior leads (inferior axis) indicates an exit on the anterior/superior walls, whereas a negative QRS in the inferior leads (superior axis) indicates an exit on the inferior/posterior walls. An early R-wave transition in precordial leads (positive by lead V3) suggests a basal site of origin and a poor/late R-wave transition with dominant Q wave in V4 to V6 suggests an exit near the ventricular apex. Other ECG characteristics help to distinguish endocardial versus epicardial site of origin. In general, ventricular arrhythmia originating near the epicardium tends to have a wider QRS duration with a slurred initial QRS upstroke, compared to arrhythmias arising from the endocardium. The presence of (1) a pseudo-delta wave of more than 34 ms in duration, (2) a delayed intrinsicoid deflection to the peak R wave in V2 of more than 85 ms, or (3) the shortest RS complex, measured from the earliest ventricular activation to the nadir of the first S wave in any precordial lead, of greater than 121 ms, favor an epicardial arrhythmia site of origin.38 In addition, a precordial maximum deflection index (MDI) of more than 0.55 ms also suggests arrhythmias of epicardial origin.39 MDI is calculated by dividing the earliest time to maximum deflection (maximum deflection time: MDT) in any of the precordial leads by the total QRS duration. Since most patients have multiple induced VTs during the procedure, it is useful to identify the “clinical” VT in patients. Because the 12-lead ECGs of the spontaneous arrhythmias are often not available, the stored EGM recordings from the ICD interrogation can provide useful data. By comparing the real-time ICD EGM morphologies and cycle lengths during intraoperative VT inductions with the stored EGM data from the spontaneous VT episodes (Figure 39.1), the clinical VT can often be identified.40 In addition, analysis of the EGM recording during sinus rhythm is important. If the intracardiac EGMs during the spontaneous tachycardia recorded by ICD are similar to that recorded during the sinus rhythm, the ventricular activation pattern during the tachycardia is thus similar to that during the sinus rhythm. This suggests the VT may utilize the native conduction system (Figure 39.2). Bundle branch reentry or a fascicular VT should be considered. Figure 39.1 Utility of ICD EGM recordings in VT ablation. Two different morphologies of VTs were induced during the procedure. The 12-lead ECG and intracardiac EGMs of a LBBB, right superior QRS (LBRS) VT are shown (left panel). The ICD EGMs during the tachycardia matched the stored ICD EGM during the spontaneous VT episodes (inset). A RBBB, left inferior (RBLI) VT was also induced with the ICD EGM during the tachycardia did not match that recorded during spontaneous VT episode (right panel). The LBRS VT was considered to be the “clinical” arrhythmia. It is important to note that the timing of the ICD EGM has a different “off-set” from the rest of the intracardiac recordings due to delays between the ICD programmer outputs and the EP recording system. Since the 12-lead ECG analysis is essential to regionalize the VT exit as well as for pace mapping, correct surface ECG electrode placement is critical. Incorrect ECG electrodes placement will result in misinterpretation, procedural delay, and failed outcome. Our protocol recommends the use of the electroanatomic system for VT mapping and ablation (CARTO, Biosense Webster, Diamond Bar, CA). Care must be taken for placement of the reference patch to compensate for the dilated LVs that are often leftward rotated. The reference patch should be placed in the center of the ventricular silhouette in the anterior-posterior fluoroscopic projection to assure proper registration of the navigational data. For the real-time ICD EGM recordings, a vendor-specific junction box may be obtained from the company that connects the programmer to the EP recording system in the analog channels for display. The arterial access is obtained through the right femoral artery with an 8- or 8.5-Fr sheath for the retrograde aortic approach. A “long” sheath (≥ 30 cm) may be helpful, particularly in those with significant peripheral vascular disease or tortuosity of the aorto-iliac system. For careful intraoperative hemodynamic monitoring, a separate arterial line may be necessary. In patients who have severe peripheral artery diseases or aortic valve stenosis/prosthesis, a transseptal approach should be considered. Two RV catheters are routinely placed at the RV apex and at the His bundle (His). The RVA catheter marks the ventricular apex while the His catheter marks the ventricular base and the level of the aortic valve. In addition, the His bundle recordings during VT is essential to assess arrhythmias involving the His-Purkinje system (Figure 39.2). Figure 39.2 Utility of ICD EGM recordings in VT ablation. (Panel A) Comparison of the ICD intracardiac EGM recordings during VT and during an atrial paced rhythm. Ventriculoatrial dissociation was evident during VT which was terminated by anti-tachycardia pacing (ATP). The ventricular EGMs on both the pace-sense (near-field) and the shocking electrode (far-field) recordings demonstrated identical morphologies, suggesting identical activation during VT and during native conducted beats. (Panel B) The 12-lead ECG during VT showed an RBBB-left anterior fascicular block pattern that was identical to the ECG during atrial pacing, suggesting conduction down the left posterior fascicle (LPF) during both rhythms. A LPF potential (arrow) was recorded during VT that occurred after the His bundle recording during atrial pacing. For the patient in whom an epicardial approach is anticipated, or in those who may have VTs originate near the mitral annulus, an additional coronary sinus catheter is used to outline the basal LV silhouette and mapping. For VTs originate from near the left ventricular outflow tract (LVOT), a small diameter catheter (such as the 3.3-Fr multi-electrode diagnostic micro-catheter (Map-iTTM, Access Point Technologies, Rogers, MN) is useful to record epicardial EGMs from the “summit” of the LV near the anterior interventricular vein or great cardiac vein. Intracardiac echocardiographic (ICE) imaging (CARTOSOUND, Biosense Webster) is routinely used for creating the 3D anatomical shell of the ventricular chamber and for identifying the scar with wall motion abnormalities.41 Moreover, the real-time monitoring using ICE to ensure contact between the catheter tip and tissue is useful, especially for VT originating near complex anatomical structures such as the LV outflow tract and papillary muscles. ICE is also helpful for early detection of the intra-cavitary thrombus or cardiac tamponade. The right femoral vein is usually accessed for the placement of an 8- or 10-Fr phased-array ICE catheter. Our protocol recommends the use of the open irrigated radiofrequency ablation catheter (THERMOCOOL SMARTTOUCH®, Biosense Webster, Diamond Bar, CA). The use of a contact force-sensing catheter is also standard in our laboratory to assure adequate substrate characterization and ablation lesion formation.42 The CARTOUNIVU module integrates fluoroscopic images and cine loops into the electroanatomical mapping system. Such new image integration tools have been shown to be effective in minimizing fluoroscopic exposure for operators and patients undergoing catheter ablation of complex arrhythmias. Patients with ischemic VT and endocardial ablation are most likely to benefit from this image integration tool.43 Most of patients who undergo catheter VT ablation in our laboratory are under general anesthesia. Multiple episodes of poorly tolerated arrhythmias are often induced during the procedure that required shock terminations. General anesthesia helps to control patient’s discomfort, minimizes movement and improves mapping accuracy. The disadvantages of general anesthesia include (1) VT induction may be more difficult, and (2) lower blood pressure during VTs due to the abolition of sympathetic tone for compensatory vasoconstriction. Close communication and collaboration between the anesthesiologists and the electrophysiologists are mandatory, particularly if mapping is performed during sustained VTs. Placement of intravascular catheters, creation of ablation lesions, activation of coagulation factors, and potential disruption of atherosclerotic plaques all contribute to a risk of thromboembolism during and after the ablation procedures. The risk of stroke or thromboembolism for LV catheter VT ablation is at least as high as any left heart catheterization at ~1%. We recommend meticulous monitoring of the activated clotting time (ACT) every 20 to 30 minutes. The target ACT is maintained at ~300 to 350 seconds. A lower level anticoagulation for prevention of venous thromboembolic complications is also recommended during right heart catheterization/ablation procedures as most patients have prolonged placement of multiple intravascular catheters. If epicardial mapping/ablation is being considered, pericardial access should be obtained before anticoagulation is initiated. Otherwise, complete reversal of anticoagulation must be achieved before attempting the percutaneous subxyphoid pericardial puncture.30,44 A systematic approach of VT ablation should be followed (Figure 39.3). A “hybrid” approach combining both conventional and substrate-based mapping techniques is utilized to optimize the procedural workflow and to improve outcome. Figure 39.3 A flow diagram for the approach of catheter mapping and ablation of VT. A “hybrid” protocol with a combination of both “conventional” and “substrate-based” mapping techniques are utilized. Induction of VT should be performed to confirm the diagnosis, to evaluate for bundle branch reentry and to ascertain which arrhythmias are the clinical VTs. Programmed stimulation is usually performed from 2 ventricular sites (RV apex and outflow tract) with up to 3 extra-stimuli after basic drive cycle lengths of 600 and 400 ms. Most patients have multiple inducible arrhythmias that include hemodynamically unstable and morphologically distinct VTs that do not allow extensive mapping during arrhythmias. Electroanatomical characterization of the arrhythmia substrate during sinus or paced rhythm should therefore be performed to minimize VT inductions, especially in patients with advanced structural heart disease. Substrate characterization identifies areas of abnormal myocardium, particularly areas of slow conduction within the low voltage scar that may constitute the potential reentry circuit. It allows more detailed mapping focusing on limited areas of interest and facilitates the design of the ablation lesions. Even for hemodynamically tolerated VTs, sustained arrhythmias of prolong duration may induce ischemia or exacerbation of heart failure. Substrate mapping is therefore recommended even in patients with hemodynamically stable VTs. Integrating substrate mapping and conventional mapping techniques is essential for successful and efficient ablation procedures. The use of a multi-electrode mapping catheter should be considered for detailed tissue characterization with high-density recordings. Substrate mapping techniques include (1) local EGM voltage analysis (voltage mapping), (2) defining the “conducting channels,” (3) identifying EGMs with late, fractionated local abnormal ventricular activities (LAVAs), (4) pace mapping, and (5) detecting the electrical unexcitable scar (EUS). Detailed descriptions of the substrate mapping techniques are beyond the scope of this chapter and only a brief discussion is provided. A 3D anatomical shell of the cardiac chamber coupled with bipolar ventricular signals is constructed by using the CARTO system. The normal endocardium is defined as bipolar EGM voltage ≥ 1.5 mV. “Dense scar” is arbitrarily defined as areas with the voltage less than 0.5 mV. The “border zone” is defined as the transition zone between dense scar and normal tissue (0.5 to 1.5 mV).45 Such voltage profile has been correlated to human histopathology.46 Most isthmus sites are located in areas of low voltage scar (< 0.5 mV) while exits are often located in the border zone (0.5 to 1.5 mV).34,47 Analysis of the voltage profiles identifies the approximate location of the VT circuit, and further mapping efforts should be then directed toward the low voltage scar (< 0.5 mV) for localizing the potential VT isthmus. By careful voltage color adjustment, VT-related conducting channels may be identified as visible corridors with consecutive EGM recordings of relatively higher signal amplitude bordered by lower voltage scars (Figure 39.4). However, the scar tissue is not homogeneous and a single cutoff threshold value is not feasible in most cases. The color voltage threshold has to be individualized to optimize identification of potential conducting channels. The majority of channels can be identified when the voltage threshold is set between 0.2 to 0.3 mV.34,48 Figure 39.4 Identification of the conducting channels of ventricular tachycardia (VT) reentrant circuits. Bipolar voltage maps in 2 patients with monomorphic VT are shown. Endocardial sites within the circuits were identified by entrainment mapping for the corresponding VTs. The standard color range for the voltage maps were depicted on the left panels, and the maps after voltage threshold adjustment was depicted on the right panels. Panel A: RV maps in a patient with an arrhythmogenic right ventricular cardiomyopathy (ARVC) and sustained LBBB–left inferior axis VT. At a voltage threshold of 0.4 mV (dense scar depicted by gray at 0.38 mV), a corridor characterized by a higher voltage followed the path defined by orthodromically activated VT sites was visualized. Panel B: LV maps in a patient with an ischemic cardiomyopathy and prior infarction who presented with a RBBB–right superior axis VT. At a voltage threshold of 0.5 mV (dense scar depicted by gray at 0.4 mV), evidence of a conduit with a higher voltage that follows orthodromic activating sites during reentrant VT was identified. (Adapted with permission from Hsia et al., Heart Rhythm. 2006;3:503–512.) Nevertheless, the mere presence of voltage channels has a low specificity in predicting the location of the VT isthmus.49 Additional mapping efforts are needed to establish its functional significance, such as activation mapping, pace mapping and entrainment mapping. The presence of late fractionated potentials/LAVA within the voltage channel significantly improves the specificity for identifying the clinical VT isthmus. LAVAs reflect local conduction delay in the diseased myocardium and have been associated with the VT isthmus.48,50,51 Using entrainment mapping, the majority of VT isthmus sites are associated with the presence of late potentials, which are commonly found inside the dense scar. Furthermore, the degree of local conduction delay (QRS–late potential interval) is significantly longer (≥ 200 ms) at sites near the entrance and mid-isthmus of a VT circuit compared to that of an exit site (Table 39.1, Figure 39.5). LAVA may be better identified during RVA pacing or introduction of ventricular extrastimuli compared to sinus rhythm, suggesting that changes in wavefront activation or decremental impulse propagation might unmask areas of block and slow conduction into the channels.48 Figure 39.5 The relationship between late potentials and the VT circuit. A LV voltage map shows the patient to have a large inferolateral scar and a RBBB–right superior VT. The exit (Exit), isthmus (Isth), entrance (Ent), and the outer loop were defined by entrainment. The locations of late potentials are shown (yellow lines), and the QRS–late potentials (ms) are measured from the beginning of QRS to the latest late potentials. The degree of local conduction delay (QRS–late potential interval) was significantly longer at sites near the entrance and isthmus of the VT circuit. The yellow lines depict dyskinetic areas noted on ICE. Since the reentrant circuit may be defined by fixed or functional block, the VT isthmus may also be identified by pace mapping.52 Pacing near or at the exit of a VT should produce a QRS morphology similar to that of the VT. After defining the VT exit, pacing progressively away from the border zone into the dense scar (< 0.5 mV) should be performed to identify the potential isthmus site. Pace mapping in the VT isthmus should produce a QRS similar to that of the VT with a longer stimulus-to-QRS interval (Stim–QRS) due to slow conduction within the channel (Figure 39.6). A long Stim–QRS (> 40 ms) recorded deeper into the scar is a marker for abnormal conduction that is often associated with the VT isthmus.52 Figure 39.6 Pace mapping for identification of the VT isthmus. An epicardial voltage map in a patient with ARVC (left) are shown. Large low-voltage areas were found in the anterior aspect of the RV epicardium. A potential “channel” was identified that corresponded to the VT isthmus. Pace mapping along the “channel” (blue dots) resulted in progressively longer Stim–QRS intervals. The pace map (arrow) showed a good match of RBRI VT with a long Stim–QRS interval of 128 ms, suggesting at the VT isthmus. Radiofrequency ablation transecting the isthmus rendered the VT noninducible. Scar areas with noncapture at high-output, unipolar pacing (> 10 mA at 2-ms pulse width) defines the electrically unexcitable scar (EUS).53 These areas have bipolar EGM amplitudes of ≤ 0.2 mV and are often located in proximity to the reentry circuit isthmuses and conduction channels. Information obtained during substrate map should be classified and tagged on the voltage map for reference (the voltage map records potential VT-related conducting channel, EUS, late potentials, good pace map sites). These data help to define the geometry of the circuit and its relationship to the underlying scar, to identify the VT isthmus and to formulate the ablation lesions. After substrate mapping, the putative VT circuit must be confirmed by collaborative mapping methods. The mapping/ablation catheter should be placed at the putative isthmus locations within the VT circuit for limited activation mapping and entrainment mapping during arrhythmias. The criteria for identifying the VT isthmus in substrate mapping may not be applicable with the advent of multi-electrode mapping catheters. The local EGM voltages are dependent on the recording electrode size, inter-electrode spacing, and the angle of wavefront propagation.54 Comparing the standard ablation catheter with the 3.5- to 4.0-mm distal electrode, tissue characterization using the small (1.0 mm), closely spaced electrodes (PENTARAY® NAV, Biosense Webster) resulted in a smaller low-voltage area and dense scar size.55 High-density, high-resolution mapping minimizes the effects of far-field recordings and helps to identify heterogeneity within the low-voltage scar, localizing channels of surviving myocardial bundles (Figure 39.7).55,56 However, prospective studies comparing multi-electrode mapping with point-by-point, standard-size electrode recording are needed to establish new criteria/thresholds to define the potential VT isthmus sites. Figure 39.7 Utility of a multipolar catheter to identify VT substrate. Panel A: Bipolar voltage map of LV with large anterior infarction and apical aneurysm was shown. A multipolar catheter (PENTAARAY ® NAV, Biosense Webster) was located on LV anterior septum within the dense scar. (Panel B) During VT, mid diastolic potentials preceded QRS by 152 ms were recorded on Pen5-6 that propagated toward other adjacent electrode pairs. Pace mapping from Pen5-6 located near the mid-anterior scar showed a perfect QRS match to VT with a long Stim–QRS interval of 170 ms.
How to Map and Ablate Hemodynamically Tolerated Ventricular Tachycardias
Kojiro Tanimoto, MD, PhD; Henry H. Hsia, MD
Introduction
Preprocedural Planning
History and Data Review
Assessment of Myocardial Ischemia
Evaluation of Cardiac Function and Wall Motion
The Electrocardiogram (ECG)
Procedure
Patient Preparation
Anesthesia
Anticoagulation
General Principles
Substrate Mapping
Voltage Mapping
Conducting Channels
Local Abnormal Ventricular Activities
Pace Mapping
Electrically Unexcitable Scar (EUS)
High-Density Multi-Electrode Mapping
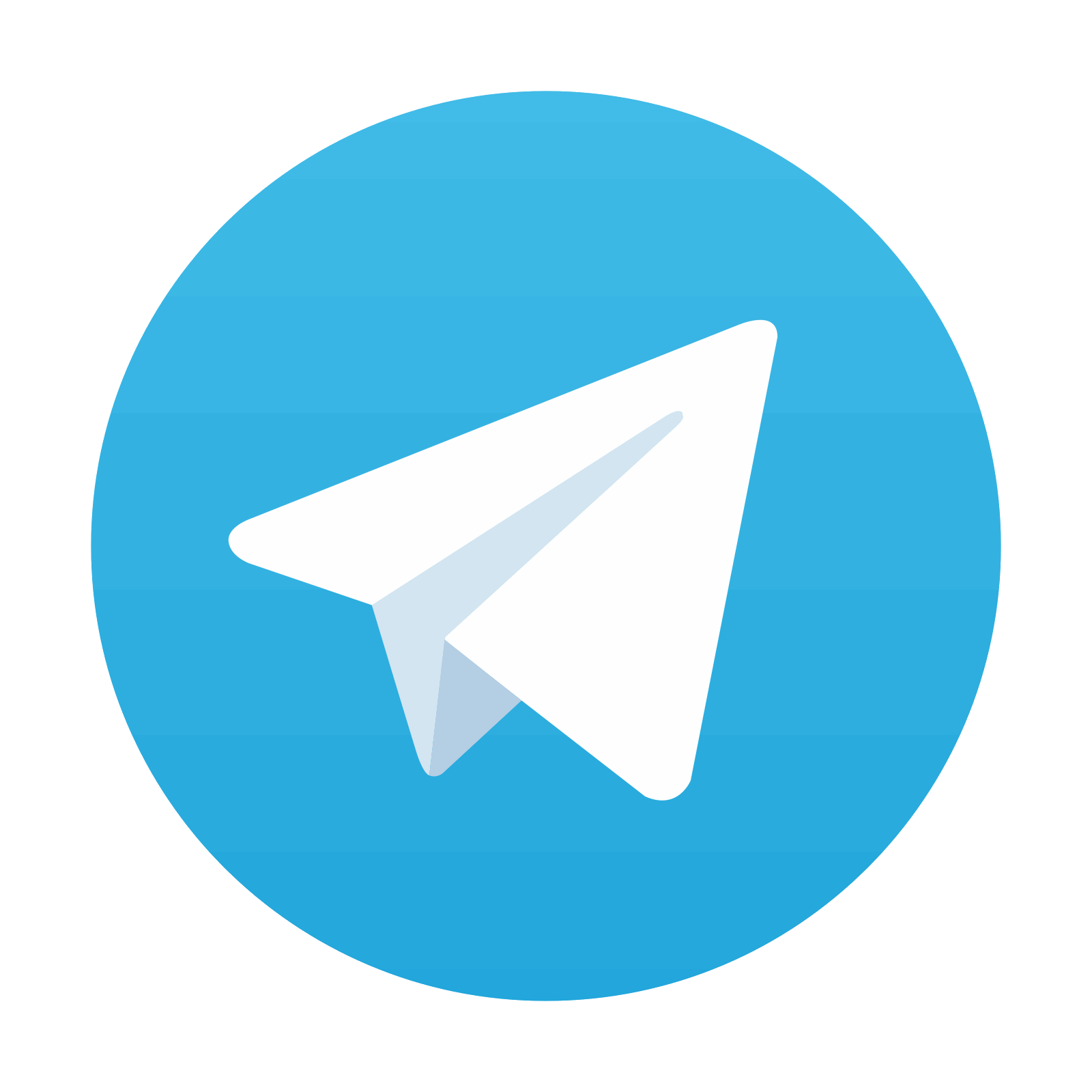
Stay updated, free articles. Join our Telegram channel

Full access? Get Clinical Tree
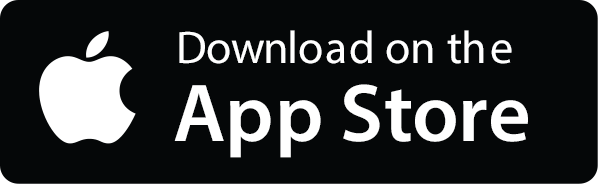
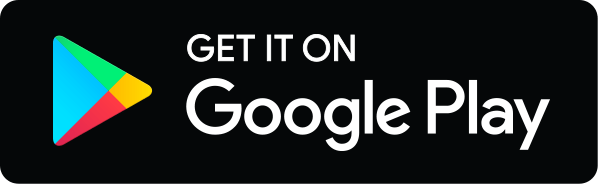