Serum creatinine rise by ≥ 0.3 mg/dl within 48 h or
Serum creatinine rise ≥150 % from baseline value within 1 week or
Urine output <0.5 ml/kg/h for >6 consecutive hours [6]
A widely used simplified definition of CI-AKI is the rise of serum creatinine by ≥0.5 mg/dL within 24–72 h after exposure to contrast media [4, 7]. Barrett et al. [5] proposed that CI-AKI can be diagnosed in the presence of an absolute increase in serum creatinine by ≥0.5 mg/dL or a relative increase in serum creatinine by ≥25 % from baseline within 72 h after contrast administration. The most recently published Kidney Disease: Improving Global Outcomes (KDIGO) definition of CI-AKI has been widely adopted by the majority of the national cardiovascular intervention societies and is based on the fulfilment of at least one of the following criteria: (a) an absolute increase in serum creatinine by ≥0.3 mg/dL from baseline within 48 h, (b) a relative increase in serum creatinine by ≥1.5 fold from the baseline value, which is known or presumed to have occurred within 1 week from administration of contrast agent, or (c) a decline in urine output by ≤0.5 mL/kg/h for a period of at least 6 h [6].
The Acute Dialysis Quality Initiative (ADQI) RIFLE and Acute Kidney Injury Network (AKIN) staging systems have demonstrated that the risk of mortality is higher when the stage of AKI is higher [8, 9]. Patients with CI-AKI should be staged according to the criteria (serum creatinine or urine output) that give them the highest stage. The staging criteria are summarized in Table 14.2.
Table 14.2
RIFLE and AKIN criteria for staging of contrast-induced acute kidney injury (CI-AKI)
AKIN | Serum creatinine | Urine output | |
Stage 1 | ≥0.3 mg/dl or ≥150 to 200 % | <0.5 ml/kg/h for >6 h | |
Stage 2 | >200 to 300 % | <0.5 ml/kg/h for >12 h | |
Stage 3 | >300 % or ≥4.0 mg/dl with an acute increase of at least 0.5 mg/dl or on RRT | <0.3 ml/kg/h for 24 h or anuria for 12 h |
RIFLE | Serum creatinine or GFR | Urine output | |
Risk | Increase in serum creatinine × 1.5 or decrease in GFR >25 % | <0.5 ml/kg/h for >6 h | |
Injury | Increase in serum creatinine × 2.0 or decrease in GFR >50 % | <0.5 ml/kg/h for >12 h | |
Failure | Increase in serum creatinine × 3.0 or serum creatinine >4.0 mg/dl with an acute increase of at least 0.5 mg/dl or decrease in GFR >75 % | <0.3 ml/kg/h for 24 h or anuria for 12 h | |
Loss | Persistent acute renal failure = complete loss of kidney function >4 weeks | ||
ESRD | ESRD > 3 months |
Incidence of Contrast-Induced Acute Kidney in CTO Procedures
The incidence of CI-AKI in patients undergoing CTO intervention varies in different registries according to the used diagnostic criteria. In a recently published large scale meta-analysis including almost 19,000 CTO cases the pooled estimated rate of CI-AKI was 3.8 %, although only 20 % of the studies reported data on contrast nephropathy [10]. In another meta-analysis including 3493 CTOs a lower incidence of CI-AKI at 1.8 % was found [11]. The incidence of CI-AKI in the most recently published registries and studies varies between 0.9 and 5.4 % (Table 14.3). No significant differences with regards to contrast volume have been shown between different subsets such as femoral vs radial access [22], in-stent restenosis vs de novo lesions [23] and patients with vs without previous coronary artery bypass grafting (CABG) [13]. The recent development of the “hybrid approach” to CTO crossing has resulted in a significant reduction in total fluoroscopy time and contrast utilization along with an improved technical success rate [24].
Table 14.3
Prevalence of contrast-induced acute kidney injury (CI-AKI) and contrast volume use in most recent CTO registries and meta-analyses
Study type | Characteristics | Year | CTO lesions (n) | CI-AKI (%) | Contrast (ml) | |
---|---|---|---|---|---|---|
Lin et al. [12] | Retrospective study | 2014 | 516 | 5.4 | 296 ± 225a 277 ± 121b | |
El Sabbagh et al. [11] | Metanalysis | Retrograde only | 2014 | 3493 | 1.8 | 350 ± 71 |
Christopoulos et al. [13] | Registry | 2014 | 496 | NR | 250 (180–360)c | |
Karmpaliotis et al. [14] | Registry | Retrograde only | 2012 | 462 | NR | 345 ± 177 |
Michael et al. [15] | Registry | 2013 | 1361 | NR | 294 ± 158 | |
Tsuchikane et al. [16] | Registry | Retrograde only | 2013 | 801 | NR | 307 ± 137 |
Patel et al. [17] | Metanalysis | 2013 | 18,941 | 3.8 | NR | |
Danzi et al. [18] | Registry | Multiple CTOs | 2013 | 249 | NR | 400 (300–500)c |
Galassi et al. [19] | Registry | 2011 | 1983 | 0.9 | 313 ± 184 | |
Morino et al. [20] | Registry | 2010 | 528 | 1.2 | 293 (53–1097)c | |
Aguiar-Souto et al. [21] | Retrospective study | 2010 | 227 | 0.88–6.16d | 260 (200–350)c |
Risk Factors for Contrast-Induced Acute Kidney in CTO Procedures
The risk of CI-AKI in patients undergoing a coronary procedure may be estimated using a range of validated scores. The most commonly used scoring system, the Mehran score, includes variables of age, gender, eGFR or serum creatinine, haematocrit, diabetes mellitus (DM), presence of congestive heart failure, contrast volume, hypotension and haemodynamic support with intra-aortic balloon pump (IABP) [2].
The presence of established renal impairment is the single most important risk factor for development of CI-AKI following a CTO procedure. The incidence of CI-AKI in patients with pre-existing chronic kidney disease (CKD) undergoing standard PCI procedures has been reported as high as 55 %, despite adequate pre-procedure hydration [25]. The correlation of contrast media volume during CTO PCI and the development of CI-AKI has been well documented in several studies [26, 27]. During CTO PCI patients receiving ≥400 ml of contrast have an almost twofold higher incidence of CI-AKI compared to those receiving <400 ml of contrast [21]. However, in the absence of coexistent CKD and DM the incidence of CI-AKI remains low, even after high volumes of contrast media [28]. Although the existing data is limited, no significant correlations between the CTO lesion characteristics and the development of CI-AKI have been shown. Lin et al. [12] showed that severe tortuosity of CTO lesions was an independent predictor of CI-AKI, however none of the other established J-CTO criteria for lesion complexity (calcification, length, ambiguous cap) reached statistical significance. In the same study, a Mehran score >11 was clearly correlated to the development of CI-AKI after CTO PCI. On the contrary, Aguiar-Souto et al. [21] showed that clinical parameters, procedural characteristics, target vessels and Mehran scoring were not predictors for CI-AKI in CTO intervention. The risk factors for CI-AKI in CTO procedures are summarized in Table 14.4.
Table 14.4
Risk factors for development of contrast-induced acute kidney injury (CI-AKI) in patients undergoing CTO interventions
Patient-related | Procedure-related |
---|---|
Chronic kidney disease (eGFR <60 ml/min/1.73 m2) | High contrast media volume |
Renal transplant | Multiple procedures |
Older age (>75 years old) | Lesion complexity (e.g. tortuosity of CTO segment) |
Female sex | IABP |
Congestive heart failure | Hemodynamic instability |
LV impairment | |
Volume depletion | |
Nephrotoxic medication (diuretics, NSAIDs) | |
Anemia | |
Low serum albumin (<35 g/L) |
Prognostic Impact of Contrast-Induced Acute Kidney
The occurrence of CI-AKI has been associated with poor short and longterm outcomes, including increased cardiovascular mortality [29]. Patients with CI-AKI have up to fivefold higher risk of death [30, 31], while CI-AKI has been shown to be an independent predictor of 1-year mortality [32, 33]. In the majority of cases CI-AKI is transient and complete or near-complete recovery of renal function occurs within a period of 3 months [33]. However, all patients who develop AKI following coronary interventions are at increased risk for progressive longterm renal impairment [34].
In the Alberta registry [34], which included a large number of patients who underwent coronary angiography, CI-AKI was independently associated with a twofold increase in the risk of death, fourfold increase in the risk of end-stage renal disease, 1.5-fold increase in the risk of hospitalization for heart failure, and twofold increase in the risk of hospitalization with acute renal failure. This can only partially be explained by the co-existence of several comorbidities in these patients including hypertension, DM, impaired left ventricular function or heart failure. Patient with CI-AKI have been shown to be more susceptible to persistent microvascular renal dysfunction after an acute episode of ischemic tubular injury, while recurrent CI-AKIs due to frequent exposures to contrast or recurrent acute clinical events can also contribute to the chronic deterioration of renal function towards end-stage disease [32].
Prevention of Contrast-Induced Acute Kidney in CTO Procedures
Several preventive methods have been described in the literature, however the data remain controversial for the majority of them. So far, no strategies have been shown to be effective in preventing CI-AKI beyond thorough patient selection, minimizing the amount of contrast media and meticulous hydration of the patient. Preventive modalities can be distinguished in pre-procedural and intra-procedural methods and follow up. A proposed algorithm for prevention of CI-AKI in CTO interventions is shown in Fig. 14.1.


Fig. 14.1
Proposed algorithm for prevention of contrast-induced acute kidney injury in CTO interventions. CTO chronic total occlusion, eGFR estimated glomerular filtration rate (ml/min/1.73 m2), MACD maximal acceptable contrast dose, IVUS intravascular ultrasound, LV left ventricle
Pre-procedural Strategies
Patient Selection
The first step in prevention of CI-AKI in CTO procedures is the identification of patients at high risk. A thorough assessment of the risk factors and calculation of validated scores, such as the Mehran score, should be performed routinely for all patients in the CTO pre-assessment clinic. Nephrology consultation should be obtained prior to procedure for all patients at very high risk (eGFR <30 ml/min/1.73 m2).
Hydration
Patients at high risk of CI-AKI should have a thorough assessment of their volume status and receive appropriate volume expansion prior to the procedure. Intravenous 0.9 % sodium chloride has been shown to be more effective than 0.45 % sodium chloride or oral hydration in prevention of CI-AKI [35, 36]. Although most of the trials have not directly addressed the ideal protocol, the most widely used approach is the administration of intravenous 0.9 % sodium chloride at a rate of 1 mL/kg/h for 24, beginning 12 h before administration of the contrast medium, in order to achieve a urine output of >150 ml/h. This approach seems to be superior to either bolus volume expansion during the procedure [37] or removal of restrictions on oral fluid intake [36]. Patients with moderate to severe left ventricular dysfunction should receive cautious hydration with isotonic 0.45 % saline and close monitoring of urine output aiming to maintain a euvolemic state [38].
Calculation of Maximum Allowable Contrast Dose
In 1989 Cigarroa et al. [39] reported an empiric formula for calculating the maximal acceptable contrast dose (MACD):
MACD = 5 ml × weight (kg)/baseline serum creatinine (mg/dl)
N-Acetylcysteine
N-acetylcysteine (NAC) has been shown to reduce the nephrotoxic effects of contrast media via antioxidant and vasodilatory mechanisms. The initial enthusiasm following publication of the study by Tepel et al. in 2000 [42], which showed the beneficial effects of NAC in preventing CI-AKI, has now subsided following numerous heterogeneous studies and meta-analyses [43–45]. More recently, a large randomized trial demonstrated that NAC does not reduce the risk of CI-AKI or affect any relevant outcomes in high risk patients undergoing percutaneous coronary procedures [46]. There is currently no compelling evidence for the routine use of N-acetylcysteine to prevent CI-AKI [47].
Sodium Bicarbonate
Sodium bicarbonate prevents CI-AKI by inhibiting the production of free radicals. Several recent trials [48–50], including a large meta-analysis [51], suggest that volume expansion with intravenous bicarbonate is more effective compared to normal saline. Nevertheless, other studies have failed to show a clear benefit for bicarbonate volume expansion in preventing contrast-induced AKI [52, 53]. Whilst more convincing data are awaited, intravenous bicarbonate can be a useful alternative in patients with impaired LV function or heart failure undergoing prolonged CTO interventions.
Hemodialysis and Hemofiltration
Studies have shown that 2–3 h of hemodialysis can eliminate up to 90 % of the contrast [54]. Hemofiltration reduces oliguria and volume overload and maintains electrolyte balance. However, only limited data on the efficacy of hemofiltration in preventing CI-AKI in high risk patients undergoing PCI exist [55] and until the results of large randomized trials are available, it cannot be recommended as standard prophylaxis in patients undergoing CTO procedures.
Statins
Statins are known to exert several pleiotropic effects including decreased systemic inflammation and improvement of endothelial function. They can therefore demonstrate beneficial effects in CI-AKI prevention by decreasing the inflammatory response induced by contrast media and improving the renal tubular endothelial function. Indeed, several studies have demonstrated reduced CI-AKI rates in patients with renal impairment undergoing PCI following treatment with statins [56, 57], although these results have not been consistent [58, 59]. In a meta-analysis of 8 trials, Giacoppo et al. [60] showed that pre-treatment with statins was associated with significant reduction in CI-AKI, both in patient with and without baseline renal impairment. Despite the above findings the use of statins prior to PCI in order to prevent CI-AKI is not yet routine practice.
CTO Road Mapping with Computed Tomography
Computed tomography coronary angiography (CTCA) is rapidly emerging as an important component to CTO planning. It provides important information regarding the length of the occlusion, the presence of tortuosity in the occluded segment and the amount of calcification. More importantly, it is extremely useful in identifying an ambiguous proximal cap and assessing the presence of potential interventional collaterals. Several prospective studies have correlated the lesion length and the presence of calcification, measured with pre-procedure CTCA, to the procedural success [61]. Disadvantages of CT angiography include the lack of soft tissue contrast which can impair localization of the luminal borders of the CTO, a relatively high degree of radiation exposure and the use of contrast, although in the majority of cases adequate information can be achieved by a single venous injection of only 100 ml of contrast [62]. Therefore, CTCA is an extremely useful tool for CTO planning which can be translated in significant peri-procedural contrast reduction.
Intra-Procedural Strategies
General Measures
General principles for preventing CI-AKI during CTO recanalization include the administration of the lowest possible dose of contrast medium, use of iso-osmolar contrast agents, such as Iodixanol, and maintenance of haemodynamic stability throughout the procedure in order to ensure adequate renal perfusion. Further interventions to bystander coronary disease or other CTOs should be performed as staged procedures in patients with pre-existing renal impairment.
Procedural Techniques
CTO operators should be trained in all key skillsets of antegrade wiring, antegrade dissection-re-entry and retrograde CTO PCI. Adoption of the hybrid approach with early switch from a failing strategy maximizes the chance of procedural success, reduces procedure time and minimises radiation and contrast use [63]. Although there are no specific time limits for each of the algorithm steps, operators should stop pursuing a technique that has not resulted in any significant progress during a reasonable period of time [63]. In particular, antegrade contrast injections are avoided in order to prevent hydraulic expansion of the sub-intimal space. Meanwhile the use of knuckle wire techniques and specific catheters such as the CrossBoss (Boston Scientific, USA) may allow the operator to cross long vessel segments where there is anatomical ambiguity without the need for the use of contrast.
The benefits of intravascular ultrasound (IVUS) in CTO recanalization have been very well validated [64]. IVUS use during CTO interventions can reduce radiation exposure, contrast volume and procedure time. It is an excellent tool for identification of the proximal cap in stumpless occlusions, guidance of re-entry from sub-intimal tracks, assessment of appropriate stent coverage of the diseased segment and confirmation of satisfactory stent expansion and apposition. During reverse Controlled Antegrade and Retrograde Tracking (reverse CART) it can be utilised to assess the position of the retrograde wire in the subintimal space and the size of the subintimal space for optimal balloon dilatation. Moreover, tracking of the antegrade (CART) or the retrograde wire (reverse CART) towards the true lumen can be performed using direct IVUS visualization, thereby avoiding contrast.
The use of smaller size 5 Fr diagnostic catheters for retrograde opacification and tip injections via the retrograde microcatheter in order to assess the appropriateness of retrograde interventional collaterals can reduce the amount of contrast volume and should be considered in high risk patients. Uehara et al. [65] reported a case of reverse CART CTO recanalization of a right coronary artery in a high risk patient where only 10 ml of contrast media was used. The procedure was successfully performed with tip injections via the retrograde Corsair microcatheter and utilization of IVUS to guide balloon dilatation, stent sizing and post-deployment optimisation.
Forced Diuresis (RenalGuard System)
The RenalGuard system (PLC Medical Systems, Milford, Massachusetts) represents a novel approach to prevention of CI-AKI in high risk patients undergoing PCI. It is designed to reduce the toxic effects of contrast media on the kidneys by achievement of high urine output rates while maintaining euvolemia. The physiological benefits include a more rapid transit of contrast through the kidneys and reduced oxygen consumption in the medulla of the kidney [66].
The RenalGuard system is comprised of a urinary collection bag, which connects to the patient’s Foley catheter, and a high volume infusion set which connects to a standard intravenous catheter (Fig. 14.2). A console measures the volume of urine in the collection set and continuously adjusts the infusion rate to an equal volume to match the patient’s urine output. An initial bolus of ~3 ml/kg is initially infused over 30 min (pre-procedural phase). In the presence of impaired LV function (ejection fraction ≤30 %) the bolus is reduced to ≤150 ml. Following the initial bolus, diuresis is initiated with a small dose of furosemide (0.25 mg/kg). Once an optimal urine flow of ≥300 mL/h has been achieved the patient is transferred to the catheterization laboratory and the procedure begins. Controlled hydration by the RenalGuard system continues throughout the procedure (procedure phase) and for 4 h after the procedure (post-procedural phase). Additional furosemide doses should be administered when there is a decrease in urine flow below the target value. A schematic representation of the RenalGuard protocol is depicted in Fig. 14.3.



Fig. 14.2
The RenalGuard system (Reproduced with permission from PLC Medical Systems)

Fig. 14.3
Treatment protocol for the RenalGuard system (Reproduced with permission from PLC Medical Systems)
The first trial was conducted in 2012 [67] and randomized 170 patients with eGFR <30 ml/min/1.73 m2 undergoing coronary procedures to standard intravenous isotonic saline hydration versus forced diuresis with the RenalGuard system. A percentage of 4.6 % of the patients in the RenalGuard group developed CI-AKI versus 18 % in the saline group (p = 0.005). The threefold reduction in CI-AKI was also associated with a lower incidence of post-procedural major adverse clinical events, although the study was not powered to detect differences in clinical outcomes. In this study PCI was performed in 59 % of the patients.
The REMEDIAL II trial [68] was a randomized, open-label controlled trial which assigned 292 patients with baseline eGFR ≤30 ml/min/1.73 m2 or a Mehran risk score of ≥11 to either RenalGuard and NAC or intravenous sodium bicarbonate and NAC. PCI was performed in 55 % of the patients. CI-AKI occurred in 11 % in the RenalGuard group and 20.5 % in the control group (p = 0.025). Major adverse cardiac events (MACE) were similar in the two groups after 1 month of follow up, while 3 patients in the RenalGuard group and 1 patient in the control group developed congestive heart failure post-procedure.
The CIN-RG trial is a pivotal trial which is currently in progress and aims to compare RenalGuard and NAC against intravenous saline plus NAC in high risk patients scheduled to receive at least 75 ml of contrast during angiography [69]. Although the use of the RenalGuard system in high risk CTO patients has not yet been standardised, it appears to be an attractive solution for preventing CI-AKI.
Coronary Sinus Contrast Media Extraction (CINCOR System)
Another promising strategy to reduce contrast volume in CTO procedures is the coronary sinus contrast media extraction system (CINCOR Contrast Removal System, Osprey Medical, St. Paul, Minnesota). The CINCOR system comprises of an 11 Fr coronary sinus aspiration catheter which is inserted via the jugular vein. Upon activation it exerts a vacuum effect and removes contrast from the coronary sinus. Duffy et al. [70] reported no change in renal function from baseline in 26 patients with eGFR <60 ml/min/1.73 m2 undergoing coronary angiography and PCI with the use of the device. The safety and capacity of the CINCOR system to reduce the risk of CI-AKI are currently being evaluated in a large-scale randomized trial (PRESERV: Prospective Randomized Evaluation to Study the Effects of Reduced Contrast Media on the Vitality of the Kidney) [71].
Follow Up
Repeat contrast administration within a short period of time should be avoided in patients that have undergone complex CTO recanalization procedures. Renal function should be routinely assessed 48–72 h following the procedure to ensure stable renal function.
Key measures for prevention of CI-AKI during CTO are listed in Table 14.5.
Table 14.5
Methods for prevention of contrast-induced acute kidney injury (CI-AKI) in CTO interventions
Pre-procedure | Intra-procedure |
---|---|
Patient selection and risk assessment | Limitation of contrast volume |
Calculation of MACD | Iso-osmolar contrast (Iodixanol) |
Intravenous hydration | Maintain haemodynamic stability |
NAC | Hybrid CTO strategy |
Sodium bicarbonate | Stage procedures for other lesions or multiple CTOs |
Hemodialysis or hemofiltration | IVUS |
Statins | Tip injections |
Antioxidants | Reverse CART |
Theophylline | Small size retrograde catheters |
Withdrawal of nephrotoxic agents | Forced diuresis (RenalGuard) |
CTCA | Coronary sinus contrast media extraction |
Radiation Exposure Complications in CTO Interventions
Radiation exposure is higher during CTO PCI compared to non-CTO interventions because of the prolonged fluoroscopic time and repeated cine angiography [72]. Although radiation skin injury is rare, the risks of radiation-related complications are greater in CTO procedures. Moreover, operator and lab staff exposure can result in longterm adverse outcomes, such as cataract and malignancies, therefore reducing radiation exposure is a key factor in CTO interventions.
Radiation Doses in CTO Procedures
There are three different values that are currently measured by modern interventional fluoroscopic equipment: (a) the entrance surface air kerma (ESAK), measured in Gray (Gy), which represents the radiation energy released at the point where the X-ray beam enters the patient’s skin surface and includes both the incident air kerma and radiation backscattered from the tissue, (b) the dose area product (DAP), measured in Gy.cm2, which represents the product of the dose in air within the X-ray beam and the beam area, and is therefore a measure of all the radiation that enters the patient and (c) Fluoroscopic time (FT), measured in minutes, which is the time during a procedure that fluoroscopy is used. FT does not include cine acquisition imaging and is therefore inadequate to assess patient radiation. The ESAK is used to measure the deterministic risk to the patient such as skin injury, while the DAP is used to measure the stochastic risk of the patient, which involves the likelihood of developing malignancies or genetic defects in the future.
A plain chest x-ray produces a DAP of 0.08 Gy.cm2 and a background equivalent of 3 days, while the equivalents for a non-CTO PCI with one stent are 36 Gy.cm2 and 3.7 years [73]. According to a study by Suzuki et al. [72] the median ESAK for CTOs PCI was 4.6 Gy, compared to 2.4, 1.5 and 1.2 Gy for multivessel, single-vessel multiple stenosis and single stenosis PCIs respectively. Several lesion- and patient-related risk factors have been shown to affect radiation dose during percutaneous interventions. In a study of 1933 PCI procedures Fetterly et al. [74] found that lesion complexity, PCI of left circumflex artery, previous CABG, body mass index (BMI) and the number of treated lesions correlated to an increased ESAK. Similar results were found in a larger study by Delewi et al. [75] which included 9850 PCI procedures. They demonstrated that high BMI, previous history of CABG, the number of treated lesions and CTO interventions were associated with the highest patient radiation exposure.
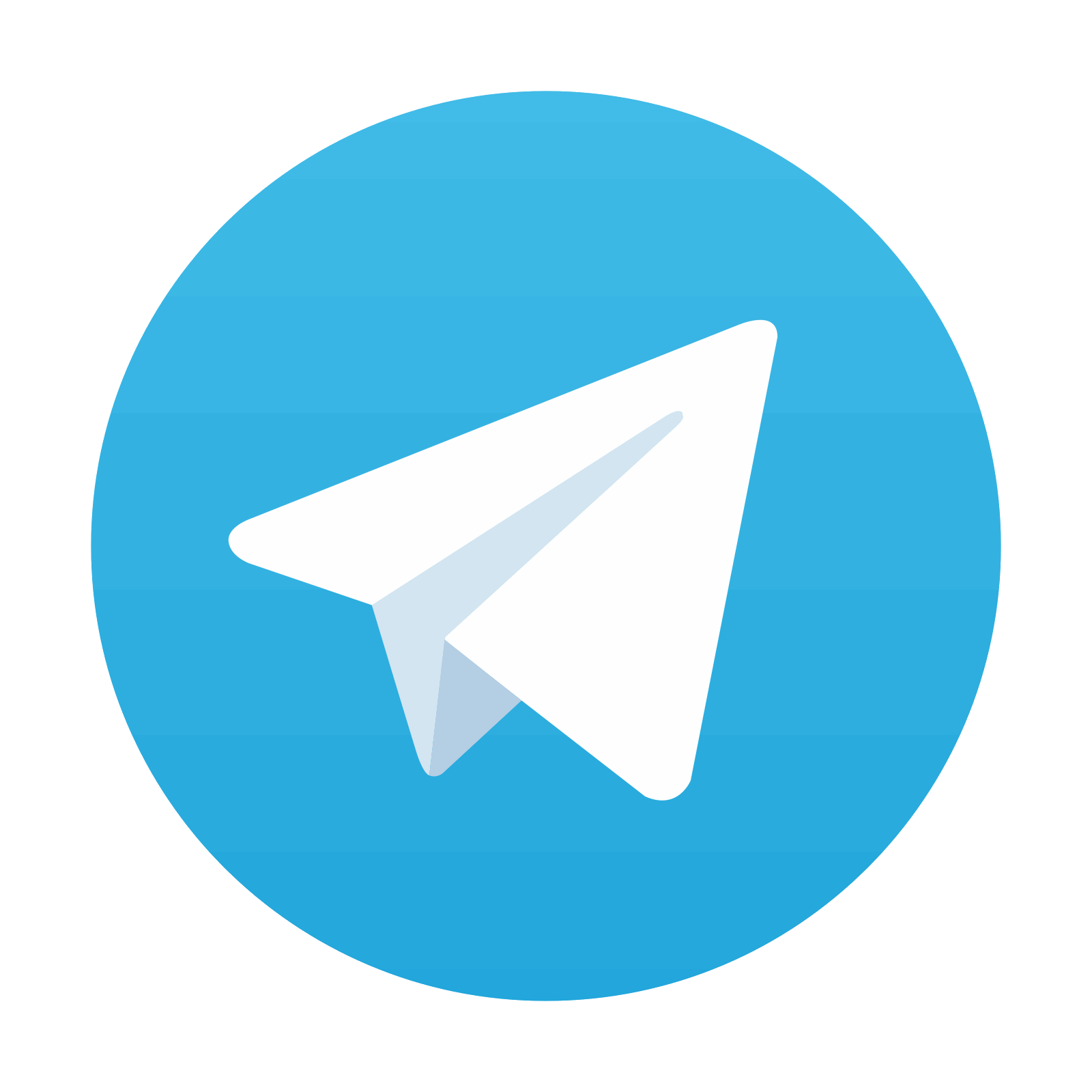
Stay updated, free articles. Join our Telegram channel

Full access? Get Clinical Tree
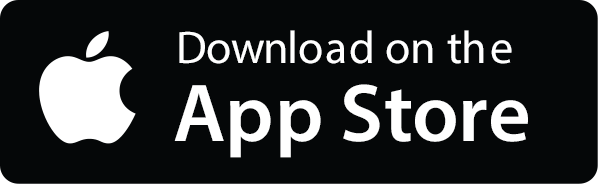
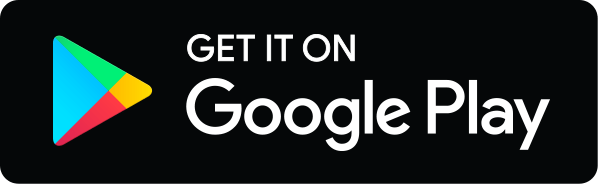