, Rohit Arora3, 4, Nicholas L. DePace5 and Aaron I. Vinik6
(1)
Autonomic Laboratory Department of Cardiology, Drexel University College of Medicine, Philadelphia, PA, USA
(2)
ANSAR Medical Technologies, Inc., Philadelphia, PA, USA
(3)
Department of Medicine, Captain James A. Lovell Federal Health Care Center, North Chicago, IL, USA
(4)
Department of Cardiology, The Chicago Medical School, North Chicago, IL, USA
(5)
Department of Cardiology, Hahnemann Hospital Drexel University College of Medicine, Philadelphia, PA, USA
(6)
Department of Medicine, Eastern Virginia Medical School Strelitz Diabetes Research Center, Norfolk, VA, USA
In most physiological conditions, sympathetic and vagal activities modulating heart period undergo a reciprocal regulation, leading to the concept of sympathovagal balance. This pattern can be indirectly quantified by computing the spectral powers of the oscillatory components corresponding to respiratory acts and to vasomotor waves present in heart rate variability. [1]
The PSNS and SNS, together, occupy a unique position within the body. In so far as they control and coordinate the organs of the body, their function tends to precede the function of the end organs. Since it is dysfunction of the end organs that tends to generate symptoms, dysfunction of the PSNS or SNS is an early indicator of impending end-organ dysfunction. This explains two significant issues regarding the knowledge of the ANS: a “good-news” and a “bad-news” deal. The bad news is the lack of P&S data, especially as it pertains to what is taught in medical school, let alone what is available to the physician. The dearth of data is due to the difficulty in measuring P&S activity. Measuring end-organ function in an attempt to understand the underlying P&S function is fraught with assumption and approximation. Measurements of end-organ function are inherently isolated from other systems, including the P and S nervous systems.
It is a fundamental mathematical law that a single measure of a system (the ANS) with two independent parts (the PSNS and SNS) cannot describe those two parts. This is analogous to analyzing cholesterol by only measuring total cholesterol. Today it is known that to properly diagnose cardiovascular risk, LDL, HDL, and triglycerides are necessary. Any attempt to assess with only total cholesterol forces assumptions and approximations, which are often misleading, incomplete, or incorrect. These assumptions and approximations are further confounded by the fact that PSNS or SNS dysfunction tends to be asymptomatic. A second measure is required. Leaving the details for later, signal processing of electrocardiographic (EKG) and respiratory activity data enables independent and simultaneous P&S measurements (P&S monitoring), which is noninvasive and quantitative.
Given the unique position of the parasympathetics and the sympathetics and the fact that changes in their function precede end-organ changes, the good news is that P&S monitoring documents early, asymptomatic, preclinical changes in a patient. This promotes preventative medicine, subserves wellness, and primary care and can lead to cost savings, both to the patient and the nation. P and S changes are best described by the term sympathovagal balance (SB) [1–3], which is measured as the ratio of SNS to PSNS activity at rest. Abnormal changes are often demonstrated as imbalances between P and S activity. Correcting P and S imbalance both relieves and prevents symptoms [4].
P&S monitoring facilitates both sides of medicine. As mandated by the US Congress in the 2003 Medicare Modernization Act and in the 2010 Patient Protection and Affordable Care Act, more information through ancillary testing is required to enable evidence- and value-based medicine and comparative effectiveness benefit analysis. Ancillary testing that fulfills these requirements supports both emergency rescue medicine, as well as, proactive, preventative healthcare, and provides more information that improves patient outcomes and reduces healthcare costs. The additional information documenting a patient’s P&S response to disease, lifestyle, genetics, injury, exposure, therapy, and treatment supports emergency, rescue medicine, and proactive, preventative healthcare and, with current ancillary tests, completes the congressional mandate.
P&S monitoring is essential in practicing evidence-based medicine. It provides additional, important, data that is specific to the individual patient’s physiology. With additional information that is more specific to the individual patient, in terms of that patient’s response to disease and therapy, P&S monitoring is value-based medicine. It guides additional testing to improve the differential diagnosis. It guides therapy and treatment, including selection, titration, and dosing of agent(s) for the individual patient. Improved titration often leads to lower dose and shorter-term therapy. The additional information from P&S monitoring documents comparative effectiveness benefit. Additional information improves the understanding of organ function and dysfunction. This and changes in organ dysfunction upon follow-up P&S monitoring provide data as to what therapies or additional ancillary tests are most effective for that specific patient. Understanding the interplay among therapies, which is often P or S active (e.g., beta-blockers, antihypertensives, antidepressants, bronchodilators, and vasopressors), helps to reduce a patient’s medication load and hospitalizations. This is most often accomplished through restoring and maintaining normal SB. When SB is normalized, secondary symptoms are often ameliorated or relieved, reducing the need for the associated therapy, treatment, or hospitalization. Throughout, additional P&S information ultimately reduces healthcare costs, both for the individual and for the nation.
As mentioned earlier, the parasympathetics and the sympathetics, together, must affect a great compromise, heartbeat by heartbeat and breath by breath, in order to properly mediate the needs of virtually all cells within the body. Variations in the instantaneous pulse rate were known since the 1700s [5]. Heart rate (HR) regularly fluctuates with physiologic processes like breathing [6, 7]. The heartbeat interval (HBI) as presented in an instantaneous HR (IHR) signal (i.e., the cardiogram) or a beat-to-beat blood pressure (BP) signal can be noninvasive measures of total ANS response. HR variability (HRV) analysis is additional useful information extracted from an electrocardiogram [8, 9]. HRV is affected by numerous factors including BP, temperature, respirations, biochemical influence of acid-based balance, state of oxygenation, ventilation, and psychological parameters. These parameters are known to affect the ANS. HRV is accepted as the reflection of the cardiovascular control exerted by total autonomic activity and a direct result of combined ANS functioning.
The observation that HR and BP vary from beat to beat was first made by Stephen Hales who, in the eighteenth century, performed the first quantitative measurements of arterial BP. He observed a correlation between the respiratory cycle, beat-to-beat systolic BP, and inter-beat interval [5]. The measure underlying HRV or beat-to-beat BP, the HBI, depends on P and S activity as well [10]. His findings were confirmed by the Dutch cardiologist Karel Frederik Wenckebach (the same Wenckebach for whom a type of second-degree heart block still bears his name) who wrote that a variable pulse rate is a sign of a healthy heart [11]. Analyzing HBI data provides one window into understanding the mechanisms of total ANS function [2]. Therefore, there is interest in the study of the HBI (whether as HRV or beat-to-beat BP) with the purpose of finding new parameters and new criteria for new and improved differential diagnoses of diseases associated with abnormal P and S activity [12]. However, as stated above, the HBI is but one measure of a two-component system; therefore, methodologies based solely on the HBI cannot independently and simultaneously measure P and S activity.
A History of Confusion
Appel et al. [13] state that cardiovascular variables such as HR, BP, stroke volume, and the shape of electrocardiographic complexes all fluctuate on a beat-to-beat basis. These fluctuations have traditionally been ignored or, at best, treated as noise to be averaged out. The variability in cardiovascular signals reflects the hemodynamic interplay between perturbations to cardiovascular function and the dynamic response of the cardiovascular regulatory systems. Modern signal processing techniques provide a means of analyzing beat-to-beat fluctuations in cardiovascular signals, so as to permit a quantitative, noninvasive, or minimally invasive method of assessing closed-loop hemodynamic regulation and cardiac electrical stability. As shown by many studies, HRV signals contain well-defined rhythms which include physiologic information [8–10, 14]. Based on dobutamine studies, Hogue et al. [15] found that the high-frequency (HF) component of HRV is a reliable measure of reflex-augmented cardiac vagal activity and lends further support to the importance of parasympathetic modulation of the low-frequency (LF) component of HRV. Central nervous system processing of environmental stimuli requires integration of sensory information with ongoing PSNS and SNS control of cardiovascular function. Rhythmic feedback of cardiac and baroreceptor activity contributes dynamically to homeostatic autonomic control. The main rhythms present in the cardiogram (the HBI signal) are respiratory sinus arrhythmia (RSA) and mean HR changes over time. RSA is purely, vagally, mediated. The vagus nerve represents a significant majority of the PSNS. Mean HR changes are mediated by both SNS and PSNS changes. RSA tends to cause faster (high frequency) changes in the cardiogram as compared with mean HR changes. However, that does not mean that RSA changes have to always be fast. Mean HR changes tend to be slower than RSA changes [2].
It is known that the SNS responds slower (three to five heartbeats) than the PSNS (one to two heartbeats) [2]. This is just like the accelerator and brakes (respectively) in your car, for example, so that the SNS cannot induce a cardiac tachyrhythm that the PSNS cannot stop from progressing to fibrillation or beyond. Rhythms in the LF range, between 0.04 and 0.15 Hz, are markers of sympathetic activity as modulated by parasympathetic activity [8, 9]. According to Malliani et al. [16], “an increase in sympathetic activity is characterized by a shift of the low- to high-frequency balance in favor of the LF component.” Heretofore, from analyses solely based on HBI, the clinical literature and clinical applications (e.g., Holter monitors) have assumed that an increase in LF power is an approximation of an increase in sympathetic activity [17]. This relationship has been criticized [18, 19]. Only in normal subjects or in certain, well-defined, patient subpopulations (i.e., advanced stages of chronic diseases like congestive heart failure or Parkinson’s disease), with both populations under highly restrictive respiratory activity criteria (i.e., respiratory rates faster than 12.5 breaths per minute), is the assumption valid that the LF term is only a sympathetic measure [8, 9]. The assumption is valid in these patient subpopulations because the parasympathetics are approximated to be very low with respect to the sympathetics. The assumption in the normal population is that P and S activity are assumed normal and SB is normal.
Without symptoms, or with more than one symptom, these assumptions are dangerous, especially as a basis for treatment or therapy decisions, including decisions not to treat. Remember, HRV-alone methods are combined measures of P and S activity. Also, remember that the P and the S control and coordinate the organs. Therefore, P and S dysfunction is asymptomatic before end-organ dysfunction. As a result, asymptomatic, apparently normal, patients may not be very normal at all, merely asymptomatic. For example, in HRV-alone methods, normal HRV measures or beat-to-beat BP measures may be normal, or they may include high sympathetic activity with normal-to-high parasympathetic activity. Since more variability is due to more parasympathetic activity, the normal-to-high parasympathetic activity masks low sympathetic activity. If the latter HRV condition is the case, a diagnosis is missed. Examples of missed diagnoses from normal parasympathetics and high sympathetics may be as follows: (1) prehypertension, or, worse, hypertension of a patient who is declared to be normotensive but may still be at risk for stroke; (2) sleep apnea of a patient who is still at risk for cardiovascular events or hypertension; (3) early stage P and S dysfunction due to chronic disease; or (4) the P and S results of an asymptomatic hypoglycemic event in patients with diabetes. These are but some of the reasons why HRV-alone methods are not widely applied in clinical medicine. Independent, simultaneous measures of P and S activity are required to provide the additional information needed to unambiguously differentiate P and S activity to diagnose risk of morbidity and mortality and to improve patient care through guiding therapy and reducing medication load and hospitalizations.
Even in the advanced chronic disease patients, HRV-alone analyses are misleading [20–22]. In these patients, it is assumed that the parasympathetic activity is very low as compared with sympathetic activity, so low as to “not exist.” This is so that the LF term may be assumed to be a sympathetic measure. Often times in these cases (especially if the breathing rate is low), the LF term increases in response to therapy. Therapy in these cases is sympathetic blockade (e.g., beta-blocker or antihypertensive) to protect the heart and other organs. An increase in the LF term in these cases is counterintuitive. Furthermore, as Singer’s lab has shown [23], more parasympathetic activity is beneficial, for it reduces morbidity and mortality risk [24, 25]. Furthermore, upon follow-up the assumption is no longer valid.
The HF range is a fixed, broadband range between 0.15 and 0.4 Hz. The HF can contain the rhythms regulated by parasympathetic activity [14]. Again, assuming the breathing frequency is high enough, parasympathetic or vagal activity (as manifest by RSA) is assumed to be the major contributor to the HF component. Issues arise when the respiratory frequency is below 0.15 Hz (12.5 breaths per minute). In these cases, the HF still provides data. What these data represent are unknown and not considered to be autonomic. Further, the frequency band of the HF range is purposefully wider [8, 9] than necessary for parasympathetic activity [14]. Therefore, additional frequency content is automatically included that will inflate the parasympathetic measure, overemphasizing its capability in any given patient. By way of examples, very low parasympathetic activity is known to be associated with risk of morbidity and mortality, including sudden cardiac death. Overemphasizing the parasympathetic measure is misleading in these cases, causing physicians to miss potentially life-threatening conditions. What may be worse, the indicated excess parasympathetic activity, which is associated with depression, may lead to additional medication that may not be necessary.
In general, low HRV results from either (or both) high LF power (activity) or low HF activity, but never high HF activity. Low HRV activity can be a result of:
1.
High sympathetic activity with respect to parasympathetic activity with low respiratory frequencies (less than 0.15 Hz)
2.
Low sympathetic activity with low breathing frequencies (less than 0.15 Hz) causing the low-frequency parasympathetic activity, thereby inflating the LF measure and the assumed sympathetic indication
3.
Low parasympathetic activity
4.
High parasympathetic activity, assuming low respiratory frequencies
A measure of low HRV does not sufficiently differentiate P and S dysfunction. Normal HRV, as indicated by sufficient HF activity, can be inflated by the broadband nature of the HF range, and high HRV, short of arrhythmia, cannot be differentiated.
Alcalay et al. [26] have shown that a respiratory peak in HR spectrum decreased as the dose of atropine (a cholinergic antagonist) increased when administered to human volunteers. This type of behavior, a direct dose-dependent change in vagal activity, is a clear indication of the sensitivity of the magnitude of the respiratory peak as a tool for vagal control. Eckberg and others have also documented the influence of respiratory activity (including RSA) on HRV power spectra, including LF and HF frequencies, and beat-to-beat BP [2, 8, 9, 18, 19, 26–50]. Strictly speaking, localization of the instantaneous respiratory frequency is required to remove the parasympathetic influence from the LF term. The remainder of the LF is then a measure of sympathetic activity. Artificially maintaining the subject’s respiratory frequency at or above 0.21 Hz leaves the entire LF as a sympathetic measure [36]. However, the same reference states that paced breathing at 0.21 Hz (12.5 breaths per minute) may cause hyperventilation [40]. Hyperventilation is a sympathetic stimulus, artificially reducing parasympathetic activity. This has forced the compromise at 0.21 Hz for the minimum respiratory frequency to attempt to differentiate LF from HF, returning us back to the initial assumptions that restrict methods based on the HBI alone to highly specific patient subpopulations. As Grossman [37] and others cite, the assumption that RSA is the only evidence of the vagal influence on cardiac function is overly simplistic. Collectively, this body of research indicates that the utility of RSA as a gauge of cardiovagal outflow may be optimized by controlling for physical activity, posture, breathing rate, and biofeedback [18, 36, 38, 40, 41]. Therefore, the peak, spectral, and respiratory frequency may be used as a marker for vagal activity and detector for normal functioning of vagal modulation [14]. See the chapter on “Heart Rate Variability Failed and Has Been Fixed.”
The combination of spectral analysis of HRV with spectral analysis of respiratory activity enables real-time, quantitative, non-invasive, independent, simultaneous measures of parasympathetic and sympathetic activity, both at rest and in response to various challenges. This technique enables early detection and quantification of CAND and CAN in terms that are clinically trendable and reflective of disease progression, therapeutic intervention, and outcomes. [p. 7] “Reduced Morbidity” [US Endocrine Disease. 2007; 2:2–9]
Low HRV is often associated with high risk of mortality such as in myocardial infarction (MI) patients [8, 9, 12, 14] and in subjects referred for Holter monitoring [16]. Low HRV is associated with low parasympathetic activity due to MI or cardiovascular disease (CVD). Also, patients with heart failure [17] and hypertensive hypertrophy [51] have been reported to have less HRV than healthy controls. These are conditions that are typically associated with severe parasympathetic insufficiency (possibly due to damage to vagal innervation of the heart) and may be explained by a shift of autonomic cardiac control toward sympathetic predominance [8, 9, 12, 14, 16, 17, 51, 52]. Atherosclerosis [26, 53] is associated with high sympathetic activity, which can also be associated with low HRV. The SNS uniquely controls the vasculature, directly, while the PSNS indirectly controls the vasculature via the SNS. Evidence suggests that prolonged sympathetic excess (SE) leads to parasympathetic insufficiency, low HRV, and increased risk of mortality [54, 55]. Historically [56], these examples provided tantalizing evidence that measures based solely on the HBI can indicate risk of morbidity and mortality, which are associated with poor outcomes. But as it has been shown, without concurrent analysis of respiratory activity, assessment based solely on HBI analyses (e.g., HRV or beat-to-beat BP (btbBP)) remains tantalizing.
Under experimental conditions, in short-term recordings, an increase of sympathetic activity, or a decrease in parasympathetic activity, leads to reduction of total HRV, primarily in the breathing-related component (the higher frequencies) [52]. Because sympathetic predominance seems to lower the ventricular fibrillation threshold [2], HRV alone has been suggested to be an important factor underlying the observed high risk of sudden death in patients with low HRV. In the Framingham Heart Study, low HRV was associated with increased risk of mortality [57]. Also, HRV is generally lower in older and sicker subjects than in younger and healthier subjects [26, 58–61]. Again, parasympathetic insufficiency can also cause low HRV. Age and high prevalence of subclinical cardiac diseases are accompanied by low HRV and preclude conclusions about the possible mechanisms underlying this association. It is believed that since the SNS directly controls BP, that btbBP is a more direct measure of sympathetic activity and therefore even less sensitive to parasympathetic insufficiency. HBI-alone conclusions often conflict with patient response to disease and therapy and do not generalize clinically. So, even in these conditions, HBI analyses (including HRV and btbBP) are not very specific or sensitive. It is well known that more parasympathetic activity reduces morbidity and mortality [23–25]. It is also known that too much parasympathetic activity is associated with depression and depression also increases mortality [62]. In these latter stages of disease, assessment based on HBI alone does not differentiate P from S activity, either in response to disease processes or in response to therapy. A second, independent measure of autonomic activity is required. Ignoring the effects of respiration in HRV or beat-to-beat BP spectra causes confounding errors, because respiration may entrain HR and BP oscillations over a wide range of frequencies, from 0.01 to 0.5 Hz [26]. Slowing of respiration causes a false-positive increase in LF power (an increase due to increased parasympathetic activity rather than sympathetic activity; see “P&S Monitoring Versus HRV Alone: Deep Breathing”). This greatly biases the results. Related to the importance of respiratory activity analysis is the logical conclusion that once the actual breathing rate is known, detection of the HF power should be centered around the fundamental respiration frequency (FRF) and not a default fixed frequency [2, 14, 58–60].
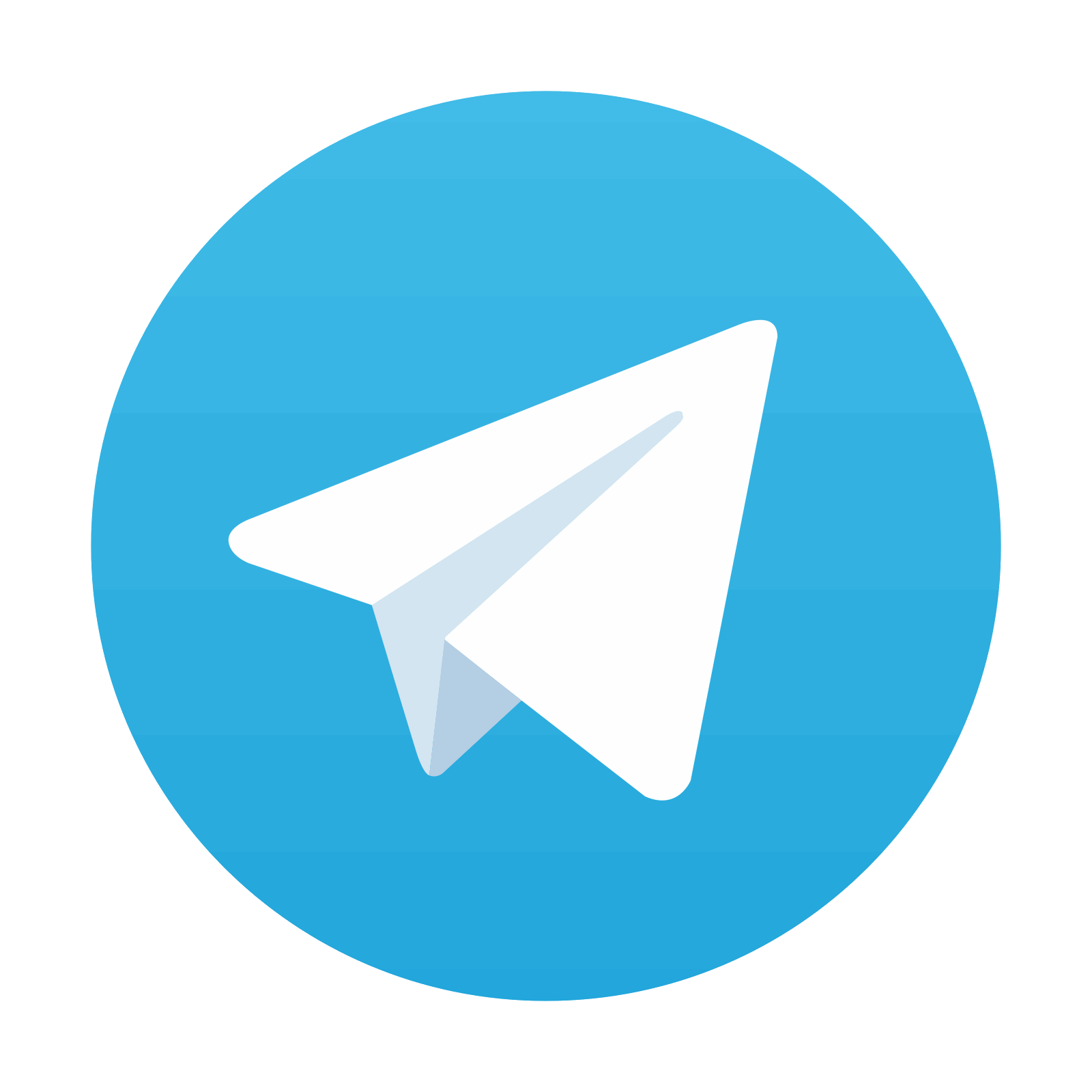
Stay updated, free articles. Join our Telegram channel

Full access? Get Clinical Tree
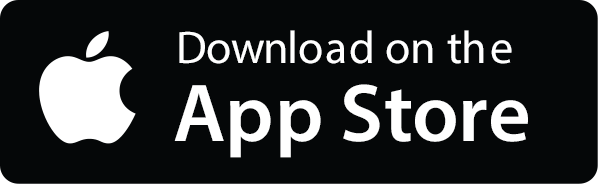
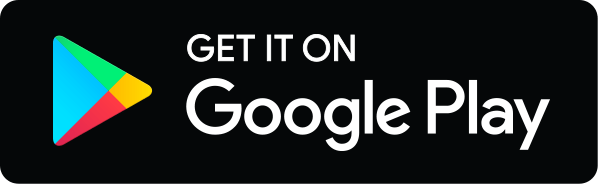