Fig. 1.1
Domingo Liotta and the Liotta Artificial Heart (1969)
The meaningful pursuit of heart replacement began in 1964 when Dr. Michael DeBakey secured funding, mainly through the auspices of then President Lyndon B. Johnson, to pursue the development of an artificial heart. It was unusual for the National Institutes of Health (NIH) to support such a project; in general, they confined their grants to pure research without any immediate clinical objective. So, this funding was unique in that regard and probably would not have been granted without Dr. DeBakey’s leadership. Also, I remember well those heady times, when we were going to the moon, among other grandiose objectives. Creating an artificial heart, comparatively speaking, seemed like a simple side project.
The funding for the artificial heart went primarily to BCM, where I was then a medical student. During that time, BCM required us to participate yearly in research projects as part of our medical school education. Although I had no particular interest in surgery, my research projects, by sheer chance, began in 1963 with Dr. Domingo Liotta, who was developing heart replacement pumps. Dr. Liotta was mainly interested in the total artificial heart [1] but was primarily occupied with developing temporary left ventricular assist devices (LVADs) . This work was initiated by Dr. DeBakey in 1964; it continued after 1972 in the THI research labs. This research initially was dedicated exclusively to pulsatile pumps. By 1989, the NIH had spent more than $266 million developing pulsatile pumps, and the companies contracted to develop this technology had spent at least as much. In all, probably more than $450 million was spent by the NIH and an equal amount by the private companies on developing pulsatile pumps [2]. At the time, the pulsatile pumps seemed to be logical candidates for both temporary and total artificial heart development.
When I completed my surgery training at BCM in 1974, I renewed my direct involvement with the development of cardiac replacement pumps at THI. The work at THI at that time (1972–1980) was directed by Dr. Jack Norman, a capable Harvard-trained physician. This was the only research on pumps being conducted in the Texas Medical Center at the time; Dr. DeBakey had suspended work on the artificial heart in 1969 after his dispute with Dr. Cooley. Under Dr. Norman’s direction, we implanted 22 intra-abdominal LVADs between 1976 and 1979, and one of the patients became the first to be bridged to transplant with an LVAD [3]. Unfortunately, none of the 22 patients were long-term survivors, but the pump itself worked well in all cases.
By the early 1980s, it seemed to me that the limiting factor in developing pulsatile pumps might be as simple as the durability of the membranes. The normal heart of an inactive adult beats approximately 100,000 times every 24 h. This poses quite a challenge to the membrane technology in pulsatile pumps , as well as to the additional technological complexity that a completely implantable total artificial heart would require.
The technological challenge of making this device fully implantable was further compounded by the fact that the left and right ventricles do not pump the same amount of blood. The left heart receives blood directly from the bronchial artery circulation; thus, in the normal adult, the amount of blood ejected from the left heart with each heartbeat is 1–2 cc more than the right heart [4]. This is not much, but in the course of a 24 h period, the difference amounts to more than 100,000 cc. This necessitated finding a way for a totally implantable artificial heart to adjust automatically for the imbalance between the left and right flow. The AbioCor total artificial heart addressed this problem primarily by shifting the blood to the right side when the left-sided pressures became elevated [5]. Although this solution seemed effective in the short term, its long-term application was never tested beyond one 17-month survivor.
The durability of the membranes seemed to be limited to about 24 months in the pump made by Thermo Cardiosystems, Inc. (TCI) and a bit longer in the Novacor pump. The Jarvik 7 total artificial heart had a similar durability problem.
By the mid-1980s, it became apparent to me that the best approach to the durability and flow imbalance problems would be a continuous-flow heart pump. Continuous-flow pumps are inherently inflow sensitive in that the higher the inflow becomes, the more they will pump (if the outflow resistance is constant) without increasing the pump speed (Fig. 1.2). This would allow more or less a physiologic Starling-type response, as well as physiologic adjustment, to control flow imbalance between the right and left heart.


Fig. 1.2
Inflow sensitivity results in a Starling-like response without changing the pump speed
However, probably the most pressing reason to pursue implantable long-term continuous-flow pumps was the durability problem. I realized that if a pump had only a 2-year life span, the pump could serve only as a prolonged bridge to transplant; therefore, although the device could be lifesaving in individual cases, it would have no epidemiologic impact on the heart failure population. The problem of changing the pump every 2 years, or else simply adding another patient to the transplant list, was and remains a barrier to the further development of pulsatile pump technology.
I had become interested in continuous-flow pumps in the late 1970s and early 1980s, when I used the Biomedicus pump (a constrained vortex continuous-flow pump) in my extracorporeal membrane oxygenation patients, as well as for temporary LVAD support. I had used this pump in 1987 in a 9-year-old patient who became the first pediatric patient to be bridged to transplant. Using this device not only enabled our patient to survive, but as I stated in the discussion of the report of the case, it also “prompted us to speculate about broader application of nonpulsatile flow, to the development of fully implantable devices for long-term cardiovascular support of the terminal heart disease patient….The potential for long-term benefit lies in meeting the requirements of the circulatory system with a nonpulsatile pump [italics added]” [6].
Making a continuous-flow pump implantable seemed to be a significant challenge. During that era, I became involved in numerous debates and discussions at meetings on this subject. Skeptics of such implantable continuous-flow pump technology cited numerous potential problems, mechanical as well as physiologic. The physiologic aberration of the baroreceptor response and potential disruption of the juxtaglomerular response were only a few of the many physiologic changes that would be produced by implantable long-term continuous-flow pumps.
In addition to these physiologic challenges, there were two important engineering barriers that were thought to be insurmountable. In the mid-1980s, the only type of implantable continuous-flow pump available used axial-flow technology . Axial-flow pumps require bearings, and you could not have a nonlubricated bearing in the bloodstream (or anywhere else)—at least, that was the conventional thought. This was an engineering axiom. (In fact, the only nonlubricated bearings I know to be in use today are those in axial-flow blood pumps.) In addition, the pump speed required to produce significant flow seemed to be, by definition, a barrier to using axial-flow technology: Speeds of more than 2500 rpm in a small device were believed to be too damaging to the blood (the “Waring blender effect”), causing too much hemolysis to have any practical value in producing meaningful blood flow.
At a National Heart, Lung, and Blood Institute (NHLBI) contractor’s meeting in Louisville, Kentucky, in 1985, I was approached (separately) by Drs. Richard Wampler and Robert Jarvik. Although they were not acquainted, they were both looking independently at engineering solutions to this problem. Dr. Wampler showed me his concept for a temporary implantable continuous-flow device that would spin at 25,000 rpm (although at the time I thought he had said 2500 rpm). Shortly afterward, Dr. Jarvik showed me an implantable, long-term axial-flow pump that would use blood-washed bearings. I recommended to Dr. Jarvik that this smaller pump be placed in the ventricle to avoid the inlet problems that had plagued the pulsatile pumps. I agreed with both of these investigators, independently, to proceed with this research in our labs at THI. (Although I am not sure I would have proceeded with Wampler’s design had I really understood that it spun at 25,000 rpm!)
Initial work with what Dr. Wampler called the Hemopump was very promising. This small pump—the size of the eraser on a #2 lead pencil (Fig. 1.3)—could produce 4–5 L of outflow. Furthermore, the device caused only minimal hemolysis in the experimental animal. Because of these promising experimental findings, we introduced this pump clinically in April 1988 in a patient dying of heart allograft transplant rejection [7]. We were able to support this patient with the Hemopump for 5 days, during which time we reversed his organ rejection. The patient survived this potentially mortal event and became a long-term transplant survivor. We used this pump in several more patients, with excellent results [8].


Fig. 1.3
The Hemopump , a tiny axial-flow pump designed to provide temporary circulatory support
The Hemopump became the first implantable continuous-flow pump to be presented to the US Food and Drug Administration (FDA) for approval. It was developed without any NIH funding. I funded the laboratory work (done in my lab), and Nimbus, a small research company, funded the manufacture of the pump. The company received the bulk of its money from investors who, naturally, wanted to apply this pump to the largest patient population possible. Therefore, for the initial clinical trial of this device (which had excellent results), the entry criterion was heart failure of any cause. The FDA, however, wanted more precisely defined entry criteria, and they recommended performing a new trial with such criteria. However, rather than fund further studies, the venture capitalists withdrew their funding and invested in a more profitable stent technology.
Fortunately, I found new support for the development of continuous-flow pumps in Helmut Reul, a German friend of mine who earned, at the University of Houston, what was probably the first Ph.D. in bioengineering. I had met him during his time in Houston, after which he had returned to Aachen, Germany, and initiated a research program. At a medical meeting in Germany in 1994, I advised him of the potential of the Hemopump technology and that it would not be further pursued in the United States. Subsequently, at his research base in Aachen, he began developing similar technology based on the Hemopump principle. The resulting device subsequently was acquired by the Abiomed company in Boston and is now in widespread use as the Impella pump, a temporary assist device.
Dr. Jarvik began working on long-term implantable axial-flow pump technology in my lab in 1985. The development was much more challenging than it had been for the Hemopump . The first few pumps made by Dr. Jarvik lasted only a short time before the nonlubricated bearing would accumulate debris and occlude the pump. However, after many revisions and experimental animal implantations, Dr. Jarvik produced a workable nonlubricated, blood-washed bearing in an axial-flow pump by the early 1990s [9].
This research showed the feasibility of continuous-flow implantable pumps for both long-term and temporary use. All of this research on continuous-flow pumps was funded internally with personal research funds of mine, by the Nimbus Company, and by Dr. Jarvik’s company, Jarvik Heart, Inc. No NIH funding supported the feasibility studies performed in the 1980s and early 1990s. This work formed the foundation for all future clinical applications of continuous-flow blood pumps.
Soon after the initial clinical success of the Hemopump , the Nimbus Company also became involved with the development of an implantable long-term continuous-flow pump. Because I was the only clinician involved in developing this technology at that time, I was the medical advisor for both Dr. Jarvik’s company1 and the Nimbus Company, which was a very small research company based in Sacramento, California (Fig. 1.4).


Fig. 1.4
Drawing of an implanted Jarvik 2000. This device was first placed in a patient in April 2000
At that time, the engineering leader at Nimbus was Dr. John Moise, a recognized expert and one of the best engineers in his field. He was struggling to develop a magnetically levitated axial-flow pump. At that time (the early 1990s), we were a small group—never more than 20 people—and we worked collegially with one another. I had shared Dr. Wampler’s research success with the Hemopump with Dr. Jarvik, and I thought nothing of doing the same with Dr. Jarvik’s success with blood-washed, nonlubricated bearings. Our primary goal was to make a pump that would ultimately benefit patients. I had never thought of or had any business interest in any of these projects.
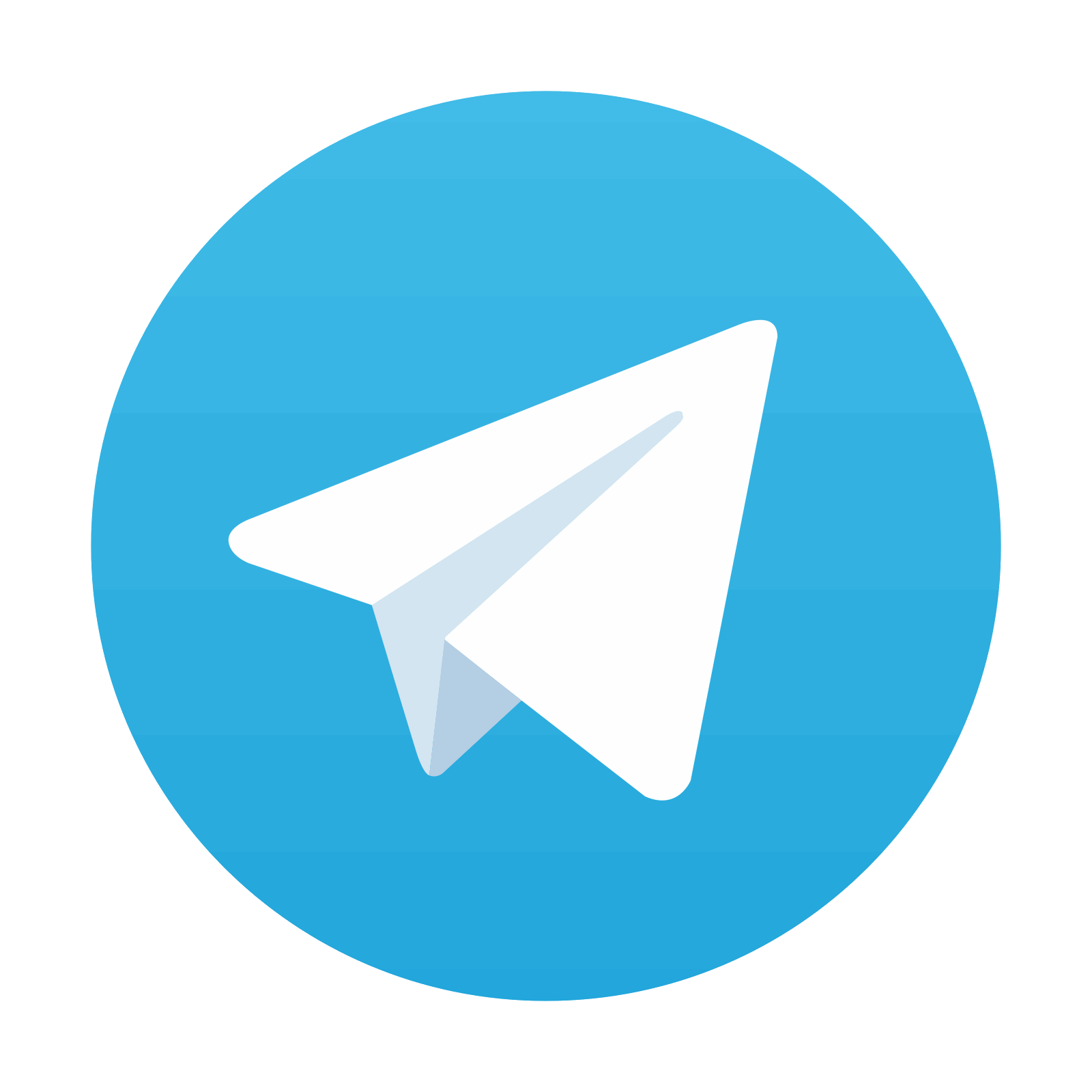
Stay updated, free articles. Join our Telegram channel

Full access? Get Clinical Tree
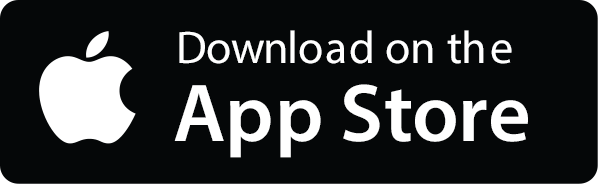
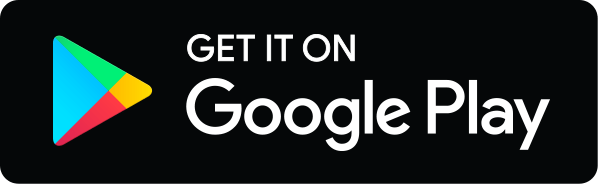