Heparin Neutralization
Justin Horricks
Mark E. Comunale
Heparin remains the anticoagulant of choice for cardiopulmonary bypass (CPB). The action of heparin is well understood, and one of its principal advantages is the ease with which it can be neutralized. Protamine remains the mainstay of this process.
This chapter discusses the process of protamine neutralization of heparin-induced anticoagulation and its side effects, including life-threatening and sometimes fatal reactions. In addition, we present recent work examining alternatives to protamine.
CHEMISTRY OF PROTAMINE
Heparin acts by enhancing the activity of antithrombin III (ATIII), a circulating proteinase inhibitor that acts on serine proteases. ATIII inhibits activated factors XIIa, XIa, IXa, Xa, thrombin (IIa), and XIIIa. Heparin-modified ATIII especially accelerates inactivation of factors Xa and thrombin (1).
Protamine, a polycationic protein derived from salmon sperm, is strongly alkaline secondary to its amino acid composition being 67% arginine. The protein has a high affinity for negatively charged sulfated glycosaminoglycans, such as heparin. However, like heparin, it also possesses some anticoagulant activity, albeit much less significant. Heparin binds to protamine ionically, producing a stable salt, which results in the loss of anticoagulant activity for both drugs. This protamine-heparin complex is subsequently cleared by the reticuloendothelial system (2).
Other clinical uses of protamine include complexing it with insulin to produce neutral protamine Hagedorn (NPH) insulin. This renders insulin relatively insoluble at neutral pH, extending the duration of action to approximately 24 hours. Similarly, complexing protamine with zinc forms protamine-zinc insulin, which has a duration of 36 hours. Protamine also inhibits angiogenesis (3), a property which led to its previously unsuccessful evaluation as an antineoplastic agent (4).
The anticoagulant effect of protamine was first demonstrated in 1937 (5). Many studies have since verified that protamine exerts a dose-dependent anticoagulant effect (6,7,8,9,10,11,12,13,14,15,16). However, debate exists as to whether this effect is clinically important and at what doses it occurs. It has been suggested that the anticoagulant effect of protamine becomes important only at doses approximately three times those required for neutralization of residual heparin (17). More recently, McLaughlin and Dunning (18) did a literature review to answer the question of whether high doses of protamine cause increased bleeding in cardiac surgery patients. They concluded that high doses of protamine can cause increased bleeding and impaired platelet function but these effects are not shown to be significant below a protamine-to-heparin dose ratio of 2.6:1 (Fig. 20.1). This relatively large therapeutic window for protamine is reassuring, but the potential for disruption of the coagulation system from protamine overdose during heparin reversal still remains. Mochizuki et al. (6) found that excess protamine prolonged the activated clotting time (ACT) and altered platelet function following CPB. A recent pilot study looked at the impact of protamine overdose following CPB, and noted that there was a trend toward an increased need for transfusion of PRBCs, FFP, and plasma coagulation factors in the group receiving excess protamine, although it was not
found to be statistically significant for the small cohort of subjects enrolled (16).
found to be statistically significant for the small cohort of subjects enrolled (16).
Many studies have looked at the mechanism by which protamine exerts its anticoagulant effect. Some have suggested that the anticoagulant effect of protamine may be attributed to the inhibition of platelet-induced aggregation. Ellison et al. (19) showed a decrease in in vitro platelet sensitivity to adenosine diphosphate (ADP) and collagen in the presence of the heparin-protamine complex. Mammen et al. (20) showed decreased platelet aggregation in response to ADP and ristocetin when protamine was administered to patients at the conclusion of CPB. They also demonstrated a decrease in platelet volume and suggested that a change in platelet surface membranes could be responsible for this altered platelet function. More recently, studies have shown that protamine mediates its anticoagulant activity through the downregulation of thrombin generation (11,14), specifically by inhibiting the activation of factor V (13).
A variety of dosing regimens have been described as appropriate for clinical administration of protamine for heparin reversal. In 1976, a human trial that compared administration of protamine equal to the total heparin dose versus protamine at a reduced dose based on a heparin half-life of 2 hours resulted in markedly decreased chest tube drainage, higher platelet counts, and postoperative clotting times closer to control values in those patients receiving the smaller protamine doses (21). Dutton et al. (22) showed that smaller protamine doses (derived by protamine titration just before the discontinuation of CPB) resulted in acceptable values for ACT at the conclusion of bypass with no subsequent evidence of heparin rebound. The previous studies indicate that most patients will tolerate an excess protamine dose of 1 to 2 mg/kg without significant adverse effects on hemostasis. However, the clinician should be aware that the greater the amount of excess protamine administered, the more likely it is that coagulation disturbances will be experienced. Therefore, a top priority should be to provide the most suitable dose of protamine for heparin reversal. The following section will examine various methods used for calculating an appropriate protamine dosage.
ASSESSMENT OF REVERSAL OF ANTICOAGULATION
Monitoring the level of anticoagulation during and following CPB is a widely accepted practice. Tests evaluating anticoagulation should yield quick results and be easily performed in the operating room. ACT, a variation of the whole-blood clotting time, has been the test most commonly utilized for this purpose and is described in detail in Chapter 18.
Calculation of Protamine Dose
The anticoagulant effects of a given dose of heparin vary considerably between patients. Bull et al. (23) demonstrated that simple weight-based dosing without monitoring of the anticoagulant effects can lead to underdosing or overdosing in some patients (Fig. 20.2). Accurate calculation of the protamine dose is therefore important because unneutralized heparin can increase postoperative bleeding. Several inherent problems in calculating protamine dosage have been pointed out by Hurt et al. (24). These include the following: (1) the dose of protamine necessary to neutralize heparin is not the same in vivo as in vitro; (2) heparin and protamine preparations vary in their potency; and (3) because heparin undergoes continuous metabolism or excretion, the dose of protamine needed decreases with time.
Many different dose regimens for protamine neutralization of heparin anticoagulation are employed. Inappropriate protamine dosage may result in inadequate reversal, protamine anticoagulation, or adverse side effects. Table 20.1 highlights the advantages and disadvantages of the different techniques.
Fixed Protamine Dose Regimen
The easiest method for calculating a protamine dose is a fixeddose ratio of protamine to heparin. This method involves giving 1.0 to 1.3 mg of protamine for each 100 units of heparin. Either the total dose of heparin administered for the case or the heparin dose given initially defines the amount of heparin to be neutralized (25,26). Simplicity constitutes the main advantage of this method, as no assays are required at the end of bypass and there is no need to measure the ACT. The disadvantage is the considerable variability in the half-life of heparin, which makes it difficult to predict the status of the coagulation system immediately preceding heparin neutralization (25).
TABLE 20.1. Comparison of methods for calculating protamine dosage | ||||||||||||||||||||||||||||||||
---|---|---|---|---|---|---|---|---|---|---|---|---|---|---|---|---|---|---|---|---|---|---|---|---|---|---|---|---|---|---|---|---|
|
Heparin-Activated Clotting Time Dose-Response Curves
This technique uses the method described by Bull et al. (27) and involves determining three ACT values and plotting them on a graph versus the heparin dose (Figs. 20.3, 20.4). The calculated amount of heparin is then neutralized by giving 1.3 mg of protamine per 100 units of heparin at the conclusion of CPB. This method is rapid and easy to use. Other advantages of this method include the following: (1) the protamine dose can be calculated more accurately than with the fixed regimen; (2) a reduced quantity of protamine is administered; and (3) infusion of blood, platelets, and fresh frozen plasma is possibly decreased (28). One disadvantage is the reliance on the ACT, which is affected by numerous factors. Culliford et al. (29) demonstrated that CPB distorts the relation between ACT and heparin levels, with the ACT becoming prolonged beyond what could be explained by the plasma-heparin concentration. The authors also noted that protamine doses calculated by this method exceeded those calculated by measuring plasma-heparin concentration, and the amount calculated by measuring plasma-heparin concentration was usually sufficient to neutralize heparin-induced anticoagulation.
Heparin Concentration and Protamine Titration
Precise determination of the plasma-heparin concentration remaining at the conclusion of bypass is a technique used by many centers. One method, which has been used as a verification test for research studies, incubates the plasma to be analyzed for heparin with factor Xa in the presence of an excess of its inhibitor (Xai). Heparin accelerates factor Xa inhibition, and residual factor X remaining after 2 minutes is measured and converted to a heparin concentration, expressed in units per milliliter (30). Culliford et al. (29) demonstrated the use of decreased protamine doses after calculation by this method as opposed to heparin-ACT dose-response curves. However, the major disadvantage of this method is the delayed acquisition of test results. Therefore, the values may not be accurate when received because the patient’s heparin concentration decreases at an unknown rate while the blood specimen is being analyzed. Furthermore, the conversion of plasma heparin concentrations into a protamine dose requires an estimation of plasma volume, which is difficult to assess at the completion of bypass (27).
Calculation of protamine dose by protamine titration was initially described by Allen et al. (31), and several variations of this method have been described (22,23,32). All methods employ tubes with several dilutions of a standard protamine
solution, to which a fixed volume of whole heparinized blood is added. The lowest protamine concentration resulting in the shortest clotting time represents the optimal neutralization of heparin. The protamine dose is then calculated based on an assumed neutralization ratio (e.g., 1 mg of protamine per 100 units of heparin) and on estimated blood volume. Therefore, this method actually constitutes an indirect way of estimating heparin concentrations. Advantages claimed for this method include administration of a lower protamine dose than with a fixed-dose regimen (23), absence of excessive postoperative bleeding response (23), and absence of heparin rebound despite reduced protamine doses (22). However, this method can be time consuming and cumbersome because of the multiple steps involved which does not lend itself well to the cardiac surgery environment.
solution, to which a fixed volume of whole heparinized blood is added. The lowest protamine concentration resulting in the shortest clotting time represents the optimal neutralization of heparin. The protamine dose is then calculated based on an assumed neutralization ratio (e.g., 1 mg of protamine per 100 units of heparin) and on estimated blood volume. Therefore, this method actually constitutes an indirect way of estimating heparin concentrations. Advantages claimed for this method include administration of a lower protamine dose than with a fixed-dose regimen (23), absence of excessive postoperative bleeding response (23), and absence of heparin rebound despite reduced protamine doses (22). However, this method can be time consuming and cumbersome because of the multiple steps involved which does not lend itself well to the cardiac surgery environment.
This paradigm changed with the introduction of automated point-of-care coagulation monitors. The Hepcon system by Medtronic has become the most well-known and utilized. The device works by using disposable cartridges that automate the protamine titration process, thereby providing a current heparin concentration along with a calculated protamine reversal dose using the patient’s height and weight. Multiple studies have looked at the Hepcon system with regard to bleeding and blood product usage with overall positive, but varying results (33,34,35,36,37,38). Dunning et al. (39) published guidelines for anticoagulation management in cardiac surgery after reviewing the evidence in 2008, and found that Hepcon monitoring is associated with a lower protamine doses and may decrease postoperative bleeding and blood product requirement. They concluded that routine use is reasonable but acknowledged that further studies are necessary. Recently, Noui et al. (40) found that protamine titration with the Hepcon device during cardiac surgery could predict a lower protamine dose and lower postoperative bleeding, along with lower perioperative red blood cell transfusion and shorter chest closure times.
OTHER DRUGS USED TO NEUTRALIZE HEPARIN
The reported incidence of severe reactions to protamine, including respiratory compromise, hypotension, and shock, varies from 0.2% to 3% in the general population to as high as 27% in patients who are allergic to fish or have received NPH insulin (41). This has led to an ongoing search for alternative agents to neutralize heparin-induced anticoagulation and also alternatives to heparin. Most of these substitutes have had limited success.
Platelet Factor 4
The α-granules of human platelets contain platelet factor 4 (PF4), which binds and neutralizes heparin when released during platelet aggregation (1). PF4 is released at the site of vascular injury, binding heparin and facilitating thrombin accumulation and clot formation (31).
Recombinant PF4 (rPF4) cloned in Escherichia coli neutralizes heparin as effectively as protamine in vitro (Fig. 20.5).
rPF4 and protamine both neutralize heparin inhibition of factor Xa and thrombin. rPF4 restored factor Xa levels more effectively than protamine (by approximately 50%-60%) and was equipotent with human PF4 (31) (Fig. 20.6). In a rat model, rPF4 was as effective as protamine in reversing heparin anticoagulation. The PF4 injection produced normal platelet counts in this model (42).
rPF4 and protamine both neutralize heparin inhibition of factor Xa and thrombin. rPF4 restored factor Xa levels more effectively than protamine (by approximately 50%-60%) and was equipotent with human PF4 (31) (Fig. 20.6). In a rat model, rPF4 was as effective as protamine in reversing heparin anticoagulation. The PF4 injection produced normal platelet counts in this model (42).
Human or rPF4 administered to Sprague-Dawley rats after heparinization had no effect on white blood cell count, platelet count, or complement levels (43) (Fig. 20.7). In contrast, protamine administered after heparin (0.1 mg/100 g) caused decreases of these same parameters. Furthermore, heparin neutralization by recombinant or human PF4 caused no decrease in mean arterial blood pressure or pathologic pulmonary changes, whereas protamine neutralization produced these adverse effects (44).
Dehmer et al. (45) compared PF4 with protamine in a prospective, double-blind study in patients undergoing cardiac catheterization. In this study, the administration of PF4 to patients was both safe and effective in the doses used. In addition, PF4 had the advantage of rapid administration, within 2 minutes, in comparison with 10 minutes for protamine. The
number of patients was small and clinical experience in humans is limited. PF4 is expensive, and its cost-effectiveness remains to be established. The advantages of rapid administration and lack of serious side effects merit a larger study in patients undergoing CPB.
number of patients was small and clinical experience in humans is limited. PF4 is expensive, and its cost-effectiveness remains to be established. The advantages of rapid administration and lack of serious side effects merit a larger study in patients undergoing CPB.
Kurrek et al. (46) showed that PF4 produces acute pulmonary hypertension in lambs. The magnitude of pulmonary hypertension was similar to that seen with protamine. However, PF4 did not cause hypoxemia and neutropenia, indicating that complement was not activated by PF4. In contrast, Cook et al. (44) studied reversal of heparin anticoagulation with PF4 in the rat and concluded that PF4 is both effective and devoid of serious side effects. Similarly, Bernabei et al.
(47) demonstrated the safety and efficacy of PF4 in baboons. The effectiveness of PF4 has been established in vitro; heparinized blood obtained from patients undergoing CPB was reversed with PF4.
(47) demonstrated the safety and efficacy of PF4 in baboons. The effectiveness of PF4 has been established in vitro; heparinized blood obtained from patients undergoing CPB was reversed with PF4.
Mixon et al. (48) performed the first open-label, phase 1 human study using rPF4. Patients received rPF4 in doses of 0.5, 1.0, 2.5, or 5.0 mg/kg over 3 minutes to reverse heparin anticoagulation after diagnostic cardiac catheterization. There were no important hemodynamic changes and the rPF4 was highly effective in neutralizing heparin. Serial measurements of rPF4 levels showed a monophasic elimination pattern with a serum half-life of 25.5 ± 13.5 minutes that was independent of dose administered. A randomized and blinded trial comparing rPF4 to protamine confirmed the safety and effectiveness of rPF4. One potential drawback to the use of rPF4 is the occasional need to emergently return CPB. In this instance, there may be a significant amount of rPF4 in circulation for as much as 2 hours (although more commonly 30-60 minutes), making reheparinization difficult.
Although rPF4 was initially being evaluated as a clinical alternative to protamine, it is not currently being developed for general clinical use. However, a recent publication revisited data from a case series that had been performed in 1995 to 1996 on patients undergoing CPB. The study involved 21 patients: 16 patients who received rPF4 for heparin reversal and 5 who received the standard protamine. This case series demonstrated that heparin anticoagulation was effectively reversed with rPF4 without serious complications and indicated that further examination of rPF4 as an alternative to protamine may be something to reconsider (49).
Protamine Variants
Wakefield et al. (50) have been working on a protamine variant, a so-called designer protamine, to neutralize the anticoagulant effect of heparin. Two such variants are currently under investigation. The first, +18BE (standard protamine being +21), has a +18 charge with acetyl and amide groups on the ends and glutamic acid replacing prolene in the background structure. The second molecule has a side group, arginine-glycine-aspartate (RGD), that is a known recognition site for platelet adhesion protein.
In a dog model (51), the reversal of anticoagulation (measured by the reversal of antifactor Xa activity) achieved with the RGD compound was superior to that achieved with standard protamine. Side effects as measured by changes in oxygen consumption, blood pressure, and cardiac output, were significantly reduced by the RGD variant. Platelet clumping and prolongation of bleeding times were also not observed with the RGD variant.
Okajima et al. (52) described the mechanism of heparin neutralization and suggested that protamine competes with ATIII for binding to heparin. Owing to a stronger affinity to heparin, protamine dissociates ATIII from the heparin-ATIII complex thereby reversing the anticoagulant function of heparin. Although protamine binds heparin through an electrostatic interaction, heparin binds ATIII through a small pentasaccharide sequence. Sela et al. (53
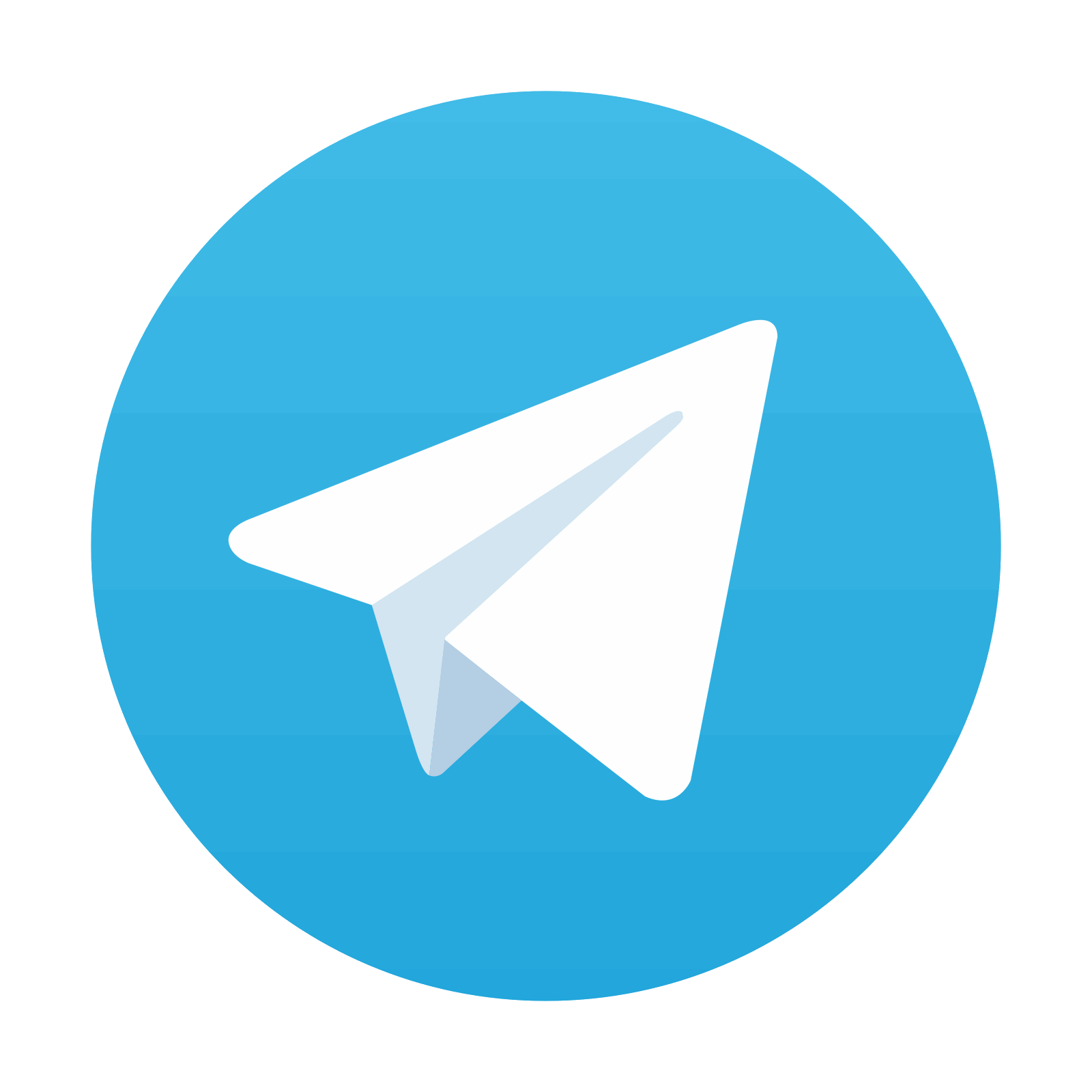
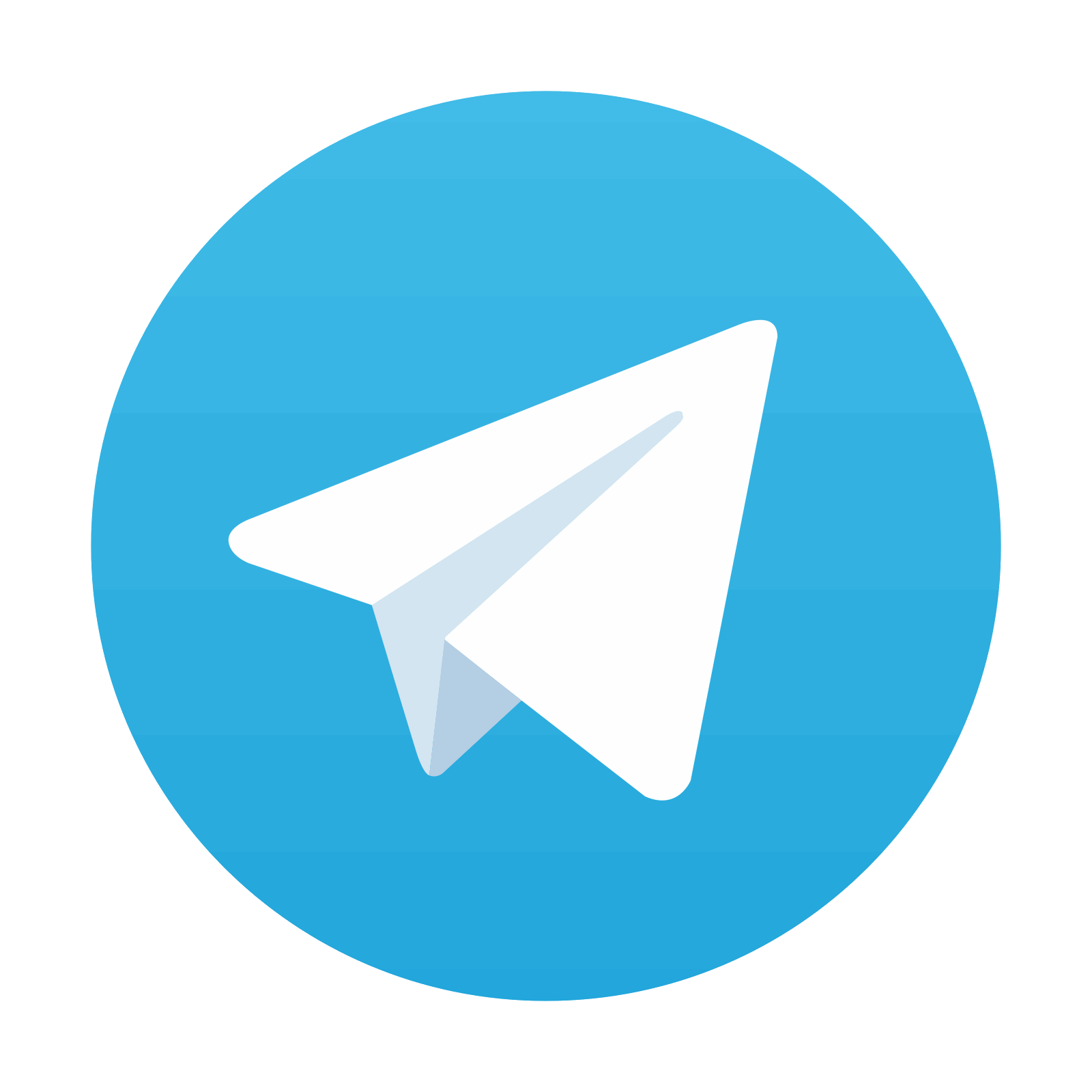
Stay updated, free articles. Join our Telegram channel

Full access? Get Clinical Tree
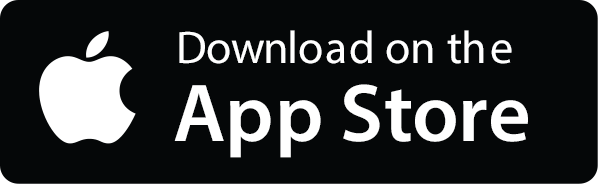
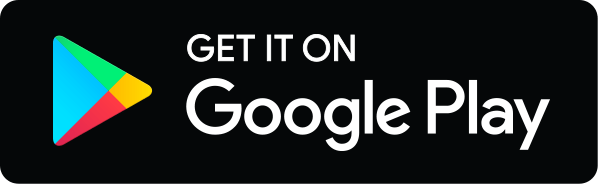
