A thorough understanding of the cardiovascular hemodynamics of valvular heart disease is essential to the medical, interventional, and/or surgical management of patients with heart valve disease. Understanding the pathophysiology of valvular heart disease requires knowledge of normal cardiovascular hemodynamics, the dynamic nature of those hemodynamics, and how abnormalities of hemodynamics affect overall heart function. This chapter describes in detail the cardiovascular hemodynamics of valvular heart disease, enabling the reader to understand the pathophysiology of valvular heart disease.
Valve stenosis describes a valve that does not open fully. The orifice is narrowed or “stenotic.” The resultant physiologic abnormality is a pressure phenomenon. Additional pressure is required to force blood across the stenotic valve, resulting in pressure elevation in the chamber in front of the valve and a pressure gradient across the valve. Chamber hypertrophy is a more likely response to pressure abnormalities than chamber dilatation. Both can occur, but dilatation is slightly more likely in the atria than ventricles. The degree of pressure increase (and thus the gradient) is an indicator of severity of stenosis.
Valve insufficiency describes a valve that does not close completely, thus resulting in “leaking” of that valve with regurgitant flow retrograde across the valve. This is the only situation where flow is “backward.” The physiologic abnormality of an insufficient valve is a volume phenomenon. The volume of blood crossing the valve retrograde is the determinant of severity of valve insufficiency. That volume is difficult to measure accurately and often can only be estimated. The size (degree of dilatation) of the receiving chamber is helpful in determining the severity of the valve insufficiency.
Combined stenosis and insufficiency describes a valve that neither opens fully nor closes completely. A stenotic valve may have a mild or moderate leak. An insufficient valve may have some leaflet thickening, leading to impaired opening of that valve. Alternatively, a valve can have a “fixed orifice,” indicating equal degrees of impaired opening and closing. Of note, a severely stenotic valve cannot leak severely—a valve cannot leak more than it can open. The worst it can be is a fixed orifice valve.
The above physiologic descriptions pertain to chronic valve disease. Both pressure phenomena and volume phenomena have been present long enough to result in upstream and downstream compensation and physiologic adjustments to the abnormalities. The hemodynamics of acute valvular heart disease are different because the heart has not had time to adjust to or compensate for sudden malfunction of a valve. Acute insufficiency of a valve is more common than acute stenosis, but either can occur.
Acute valve stenosis can occur with a prosthetic valve when a leaflet or poppet becomes “stuck.” Acute inflammatory disease (eg, rheumatic, vasculitis, lupus) can result in rapid valve swelling and narrowing. Acute endocarditis will occasionally result in valve narrowing. Thromboembolic events may occasionally result in acute valve opening impairment.
Acute valve insufficiency from endocarditis, leaflet rupture, chordal rupture, or papillary muscle rupture is a more frequent manifestation of acute valve disease. The characteristic hemodynamic findings of acute valvular disease are low cardiac output, hypotension frequently to the point of cardiogenic shock, and tachycardia.
The history and physical examination provide clues to the diagnosis, etiology, and severity of heart valve abnormalities.
Symptoms may be absent to severe and correlate with the severity of the valve disease and the degree of compensation that has occurred. Compensation is more likely to occur over time, and thus is more likely to be present with chronic rather than acute valve issues. Symptoms include fatigue, dyspnea, edema, palpitations, dizziness, and syncope. Each of these symptoms is a manifestation of a hemodynamic abnormality (Table 1-1). Symptoms may also correlate with physical position and/or degree of physical or emotional activity.
Symptom | Abnormality |
---|---|
Fatigue | Reduced cardiac output Inability to increase cardiac output |
Dyspnea | Inadequate cardiac output |
Edema | Increased venous pressure Right heart failure Tricuspid valve disease Pericardial disease |
Palpitations | Cardiac arrhythmia Hyperadrenergic state |
Dizziness | Hypotension Arrhythmia |
Syncope | Reduced cardiac output Arrhythmia Hypotension Cardiogenic shock |
Physical examination findings are present specifically related to the valve abnormalities and any associated hemodynamic changes or compensation that may have occurred. There are specific alterations in physical findings directly related to the cardiovascular hemodynamics. Blood pressure, heart rate, and heart rhythm are likely affected. Variations in heart sounds and presence of heart murmurs are indicative of valve issues. Precordial lifts, apical impulse abnormalities, palpable precordial thrills, venous pressure elevation, abnormalities of venous wave forms, and signs of fluid retention are all clues to hemodynamic alterations. The physical examination findings will change with position change and/or activity level, indicative of the dynamic nature of the cardiovascular system.
Specific symptoms, physical findings, and hemodynamic correlates of the valve abnormalities will be addressed later in this chapter.
Echo-Doppler assessment is an essential diagnostic tool to aid in the diagnosis of patients with heart valve disease. The echo-Doppler assessment is particularly good for assessing chamber size and flow abnormalities, with other hemodynamic changes inferred from the study. However, this chapter will not address echo-Doppler studies.
Cardiac catheterization studies offer the absolute best ability to measure and define the hemodynamic abnormalities associated with heart valve disease. A carefully and meticulously performed heart catheterization study provides hemodynamic data that correlate directly with physical findings. Several general principles of cardiac catheterization need to be discussed prior to addressing the abnormalities associated with each valve lesion.
The catheterization laboratory must have state-of-the-art physiologic monitoring capability and technologists and nurses trained and experienced to assist the physician in the performance of careful hemodynamic studies. It is difficult to perform an excellent hemodynamic study in a laboratory that is predominantly designed and used for coronary angiographic studies and interventions and with a staff trained specifically to perform coronary angiography studies.
Advance planning for cardiac catheterization is required. Anticipated findings should be discussed with the entire team, along with the planned techniques to measure and identify those findings. In most cases, a minimum of 2 pressure transducers will be needed to measure pressures on both sides of an affected valve. Two catheters (or a dual lumen catheter) with identical flow characteristics should be used. Careful attention to pressure gauge zeroing and transducer balancing is essential. In low-pressure systems (right heart studies), even minor deviations can be indicative of major valve issues.
When a pressure gradient is identified across a valve, it is essential to be certain that the pressure gradient is real and not secondary to inaccurate or nonbalanced pressure transducers or other technical issues (Figure 1-1) (Tip: The best way to be certain of a real pressure gradient is to measure and record the pressure gradient and then disconnect both catheters from the pressure transducers and then reconnect them to the opposite transducers. If the pressure gradient is identical to the initial measured gradient, then the system is accurate and appropriately balanced.) Do not rely on withdrawing or advancing a single catheter across a valve to obtain a pressure gradient because conditions can change in a single heartbeat, leading to inaccurate measurements.
Avoid rhythm irregularities when measuring pressures. Catheters in a cardiac chamber are likely to make physical contact with a chamber wall, resulting in premature beats (Figure 1-2). A single premature beat (either atrial or ventricular) may result in pressure and waveform abnormalities for many subsequent beats. Allow time for the heart rhythm and pressures to stabilize before measuring and recording pressures and gradients.
Make certain that the patient’s respiratory effort is not affecting pressure recordings. Breathing requires alteration in intrathoracic pressures, which are also reflected in intracardiac pressures. Hyperventilation, obstructive lung disease, and ventilators may significantly affect pressure measurements. Quiet stable breathing is best, but if a significant respiratory effect is noted, then it is essential that the patient stops or minimizes respiratory effort. (Tip: Avoid asking the patient to stop breathing; almost all patients will do a Valsalva maneuver when asked to stop breathing. This will markedly increase right heart pressures and significantly alter right heart blood flow, rendering any measurements useless. The preferred technique is to ask the patient to inspire very slowly over a prolonged time. This will result in minimal effect on pressure and allow for a more accurate measurement. Several attempts will be necessary before the patient “gets it right” [Figure 1-3].)
Remember that the patient is supine and inactive during a heart catheterization procedure. This is not particularly physiologic and likely does not reflect the conditions when the patient is symptomatic. Consider some type of intervention to alter pressures or magnify a gradient. A pharmacologic agent, exercise, oxygen, and intravascular volume adjustment are some examples, although caution is necessary when applying such intervention.
Occasionally, heart rate and rhythm and/or blood pressure may require attention before or during a heart catheterization procedure. Atrial fibrillation with rapid ventricular rate, extremes of heart rate (either fast or slow), and blood pressure extremes will affect hemodynamic findings. Pacing and/or an occasional stimulated isolated single premature beat may be necessary to bring out physiologic abnormalities.
Reliable, reproducible, and valid cardiac output determinations are necessary to calculate valve areas. Thermodilution outputs offer the benefits of ease and repeatability but may not be ideal in the setting of valve insufficiency. Consider the injecting site and the sampling site carefully to determine the appropriate technique. Calculation of the “regurgitant volume” of a valve that is insufficient requires knowing the stroke volume of the chamber as well as the “effective” stroke volume (that which reaches end organs). Unfortunately, the measurement of cardiac output is, at best, accurate to ± 25% to 30%. An accurate cardiac output is essential to determine flow across a valve. Flow is necessary for calculation of the valve area. Flow must include both the “forward” stroke volume and the “regurgitant” volume for valves that are insufficient (Figure 1-4).
Figure 1-4
Calculation of regurgitant fraction requires measuring stroke volume reaching end organs (by Fick or thermodilution output) and measuring total stroke volume of the chamber (by angiography), which will include both regurgitant output and forward output. This is usually the left ventricle.

The Gorlin formula1 (Figure 1-5) for valve area was based on a study of 10 patients. Its accuracy has been established over the years and is reliable for the aortic and mitral valves. Use of this formula for calculation of pulmonary valve area and tricuspid valve area has not been validated. Symptoms are likely to occur with a reduction of cross-sectional area of 80% to 85%.
Figure 1-5
Calculation of valve area using the Gorlin formula requires measuring cardiac output, calculating the diastolic filling period (DFP) in seconds per minute for the mitral valve or the systolic ejection period (SEP) in seconds per minute for the aortic valve multiplied by the heart rate. This number is the flow across the valve, which is then divided by the square root of the pressure gradient across the valve times an empiric constant of 37.7 for the mitral valve or 44.5 for the aortic valve. The constant corrects for comparison of this calculation with postmortem measurement in addition to orifice contraction and other factors.

The Hakki formula2 for valve area provides a very rapid (and on the fly) calculation that provides results nearly identical to calculations from the Gorlin formula. The Hakki formula is as follows: Valve area = Cardiac output/Square root of valve gradient. The mean gradient is used for the mitral valve, and either the mean or peak gradient is used for the aortic valve.
In low-output states, calculation of the aortic valve resistance3 may be a more reliable determinant of severity of valve stenosis than calculation of valve area:
Valve resistance = (Mean gradient) × (Systolic ejection period × Heart rate × 1.33/Cardiac output).
The potential errors in valve area calculations include the assumption that the valve is round, the accuracy of measurement of the pressure gradient, the calculation of flow rate, the variability of heart rate, and the uncertain effect of chamber or root enlargement.
Errors in measurement of pressure gradients are “mechanical” errors and include incorrect calibration of pressure transducers, improper alignment of pressure wave forms, failure to obtain simultaneous pressure measurements from both sides of the affected valve, catheter “occlusion” of a valve, and incorrect location of catheters. A false gradient can result from “pullback” gradients, damped pressure wave forms, prosthetic valves, pulmonary hypertension, and arrhythmias. Errors of flow calculation can occur with arrhythmias, inaccurate measurement of cardiac output, use of an “incorrect” cardiac output, and/or incorrect measurement of diastolic filling period or systolic ejection period.
Valve areas for normal adult valves are as follows:
Aortic valve = 3.5-4.5 cm2
Mitral valve = 4.0-5.0 cm2
Reference values using common coins are as follows:
Quarter = 4.6 cm2
Dime = 1.8 cm2
Flow-directed catheters have become the right heart catheter of choice in many laboratories. These catheters were developed for bedside use and are often not easy to position. Frequently, the lumen is very small, and determination of pressures and wave form may be adversely affected. However, an excellent pulmonary capillary wedge tracing may be easier to obtain with a balloon-tipped catheter. Caution is necessary with use of this catheter in the wedge position because the potential for pulmonary hemorrhage is increased.
Ideal catheters for the left (arterial) side of the heart should be selected based on access site and eventual location and use. In general, high-flow catheters will allow for angiography if indicated. The most accurate pressure gradient (or lack thereof) is determined with catheters (or other measuring device) on both sides of the valve in question. Occasionally, a transseptal approach (atrial septum) is the best approach to obtain a mitral valve gradient. Somewhat more challenging technically is retrograde positioning of a catheter from the left ventricle (LV) across the mitral valve into the left atrium (LA). Micromanometer-tipped catheters and 0.014-inch diameter pressure wires can be used, which may provide positioning benefit and also eliminate wave form artifacts.
When using a pigtail catheter, remember that the intracardiac pressure is measured from multiple “holes” in the catheter, both end holes and side holes. A false pressure can be recorded from a pigtail catheter if it is not positioned fully across a valve.
End hole–only catheters may provide a false pressure recording if they are in contact with a chamber wall rather than free in the chamber.
Pressures and wave forms require interpretation to understand fully the hemodynamics of any given patient. The remainder of this chapter will discuss in detail valve lesions and the associated hemodynamics. Throughout the rest of this chapter, keep in mind the following hemodynamic principles.
Pressure wave forms are generated from a combination of myocardial contraction and resistance to flow.
Pressure wave forms may also be influenced by chamber “stiffness” (compliance), volume of flow, and velocity of flow.
Pressure wave form and flow are not related.
Normal flow is always in a forward direction.
Any flow that is not forward is pathologic.
Pressure is rarely transmitted “backward.”
The physiology of valve stenosis is a pressure phenomenon.
The physiology of valve insufficiency is a volume phenomenon.
The common etiologies of aortic valve stenosis (AS) are congenital, rheumatic, or senile calcific. Congenital valve disorders are the most common pediatric congenital disorder. The valve may be unicuspid or bicuspid, or a tricuspid valve with fusion of 1 cusp effectively making it a bicuspid valve. Rheumatic AS is not likely to occur in an isolated fashion, but usually occurs in combination with mitral valve stenosis. Senile calcific AS occurs in an older population. The valve is trisinus with a central orifice that is narrowed by progressive valve leaflet fusion and subsequent calcification.
Symptoms are most often related to impaired cardiac output or the inability to increase cardiac output when the demand is there. This abnormal function is secondary to outflow tract obstruction. Symptoms may be gradual in onset and related to the development of obstruction of the valve. Patients may not be as aware of symptoms because the patient accommodates as the degree of obstruction progresses.
Physical findings include variation in the intensity of the second heart sound: loud when the valve remains mobile, and then diminished in intensity as the valve calcifies and becomes less mobile. A systolic ejection click may be audible if the valve is mobile. A harsh systolic ejection murmur is frequently associated with a palpable systolic thrill that may be palpable in the carotid arteries, suprasternal notch, and/or the aortic area. The murmur is transmitted to those areas in addition to the apex.
Supravalvular stenosis, subvalve membrane, and dynamic subvalve hypertrophic myopathy are always in the differential diagnosis and may, on occasion, be associated with valve stenosis as well. Aortic coarctation is occasionally associated. Aortic root disease with poststenotic dilatation is also a frequent associated abnormality.
The classic hemodynamic abnormality of AS is a systolic pressure gradient across the aortic valve. The location of the pressure gradient identifies the exact location of the valve obstruction. Low-gradient AS will occur as the cardiac output drops and/or LV function deteriorates. Left ventricular hypertrophy is the usual response to a stenotic valve, but over time, the ventricle may dilate. In either setting, left ventricular end-diastolic pressure (LVEDP) may increase, which can eventually result in diastolic heart failure and pulmonary hypertension. It is not clear what factors determine development of ventricular dilatation and LVEDP elevation. Concomitant coronary artery disease and/or hypertensive disease will contribute to the hemodynamic findings.
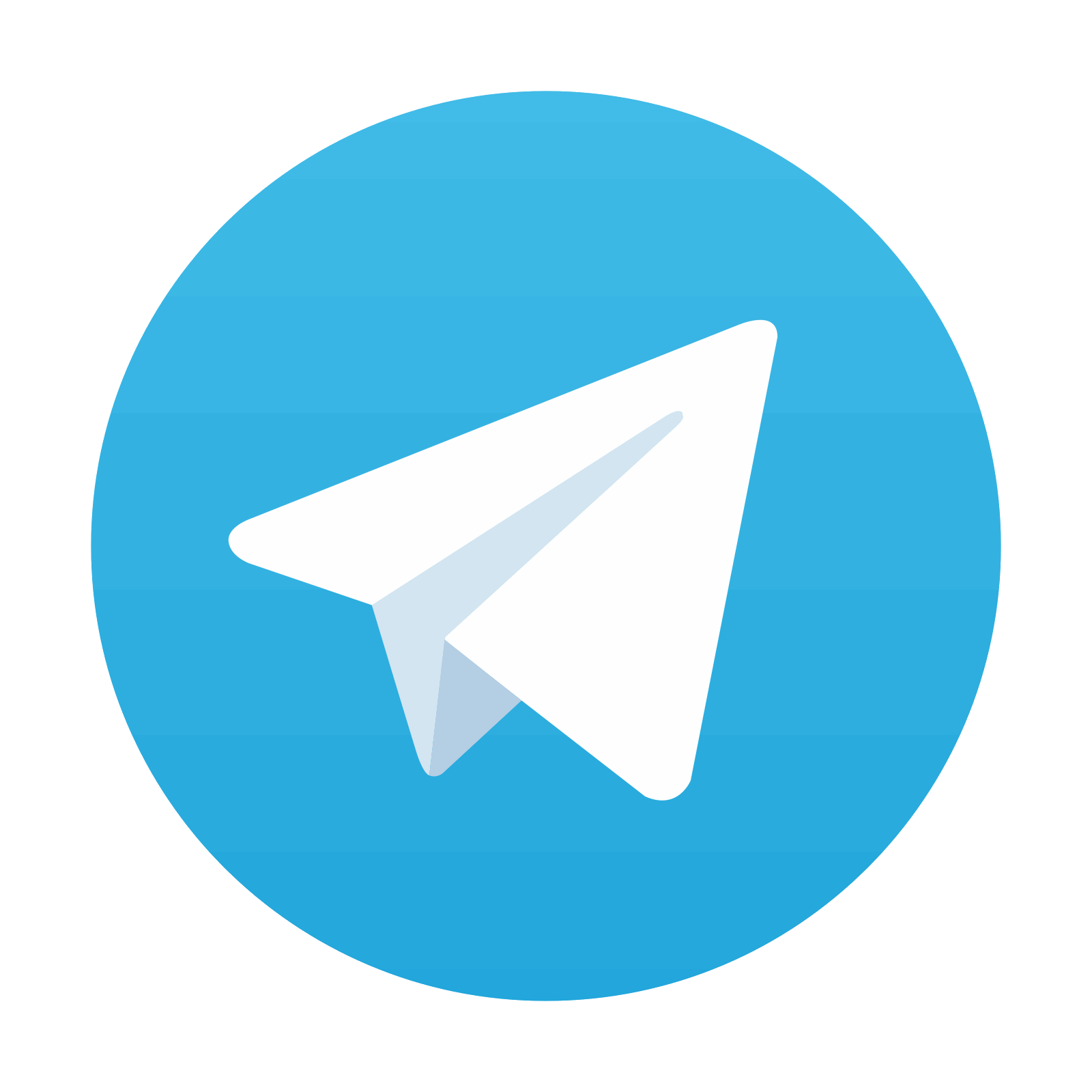
Stay updated, free articles. Join our Telegram channel

Full access? Get Clinical Tree
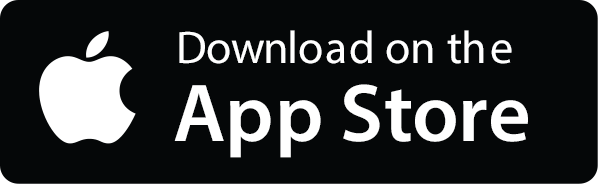
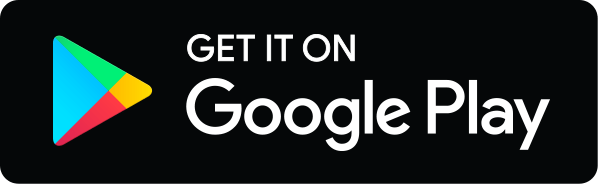