For catheterization technique, see Chapter 35, Section XXI. Summary of RA pressure abnormalities (Figures 36.5, 36.6, 36.7): PCWP is obtained by inflating the balloon-tipped catheter in a distal PA position until it occludes the PA branch. This leads to a stagnant column of blood beyond the balloon, the pressure of which equalizes with the downstream pulmonary venous pressure and thus the LA pressure. Similarly to LA pressure, PCWP has A, X, V, and Y waves. Mean PCWP is equal to mean LA pressure. However, PCWP is delayed ~50–100 ms in comparison to LA pressure because of the delay in retrograde pressure transmission from the LA through the pulmonary vasculature; therefore, PCWP’s A and V waves peak later than LA’s A and V waves. In addition, PCWP has a smoother contour with less steep and deep V downslope than LA pressure, as the pressure waveform gets damped while being transmitted from the LA through the pulmonary capillaries. Figure 36.1 The Swan–Ganz balloon flotation catheter has four ports. (1) The distal yellow port communicates with the distal tip of the catheter; it is connected to the transducer and used to obtain pressures as the catheter is advanced through the right-sided chambers (RA → PA → PCWP). (2) The proximal blue port communicates with another lumen, 30 cm proximal to the tip. When the catheter tip is in the PA, the blue port opens in the RA; at this location, the blue port is used to inject saline into the RA and obtain cardiac output by the thermodilution technique. The blue port may also be used to record RA pressure simultaneously with PA pressure, particularly when a Swan–Ganz catheter is used for continuous monitoring in the cardiac unit. (3) The balloon port communicates with the distal balloon and allows wedging of the catheter tip (PA pressure → PCWP). (4) The temperature sensor port also communicates with the distal catheter tip; after cold saline is injected through the blue port (RA), the temperature sensor (PA) analyzes the temperature change over time and allows calculation of CO. Reproduced with permission of Demos Medical from Hanna and Glancy (2012).1 Figure 36.2 Timing of atrial, ventricular, and arterial pressures in relation to each other and to the ECG. Ventricular end-diastolic pressure (EDP) is the post-A ventricular pressure and corresponds to the peak of R wave (black dot). Reproduced with permission of Demos Medical from Hanna and Glancy (2012).1 Figure 36.3 Atrial pressure tracing (RA or PCWP). A wave corresponds to atrial contraction and follows the electrocardiographic P wave. X descent corresponds to the atrial relaxation and to the downward pulling of the tricuspid annulus in early systole. V wave corresponds to atrial filling during ventricular systole while the tricuspid valve is closed. Y descent occurs in early diastole as the tricuspid valve opens and the RA rapidly empties. Thus, X descent and the upslope of V wave are systolic events (coincide with the pulse), whereas the peak of V wave, the Y descent, and the A wave are diastolic events. V wave corresponds to atrial compliance (a large V wave implies a volume overload that overwhelms the atrial compliance). On the other hand, A wave corresponds to ventricular compliance. In normal individuals, RA pressure is characterized by A wave > V wave. LA is normally less compliant than RA, because it is constrained by pulmonary veins and it is thicker than RA, and thus LA V wave > A wave. The C wave is a small positive deflection on the atrial tracing that sometimes interrupts the X descent; it corresponds to the brief protrusion of the tricuspid valve into the RA in early systole during isovolumic ventricular contraction. The A wave is only seen in sinus rhythm; it is not seen in atrial fibrillation (AF). However, a C wave may be seen in AF and may mimic the A wave. Reproduced with permission of Demos Medical from Hanna and Glancy (2012).1 Figure 36.4 RA, RV, PA, and PCWP tracings obtained while advancing the catheter from RA to PA. LV pressure from LV catheterization is shown. In general, mean RA pressure is equal to RV diastolic pressure, and mean PCWP is equal to PA diastolic pressure and LV end-diastolic pressure. RA pressure and RV end-diastolic pressure are lower than PA diastolic pressure and PCWP, except in cases of “equalization of diastolic pressure” (tamponade, constriction, severe RV failure). Reproduced with permission of Demos Medical from Hanna and Glancy (2012).1 A large V wave is a V wave that is ≥ 2× the mean PCWP, or ≥ 10 mmHg larger than the mean PCWP. While classically associated with severe MR, a large V wave implies volume overload that overwhelms the LA compliance . Thus, V wave may not be large in severe but compensated chronic MR (e.g., asymptomatic MR patients), and may, on the other hand, be large in decompensated HF even in the absence of MR. In fact, the causes of a large V wave are: (1) severe and decompensated MR; (2) decompensated LV failure; (3) mitral stenosis; (4) VSD. In one study, ~40% of patients with a large V wave did not have significant MR, while only 40% of patients with severe MR had a large V wave.2 A gigantic V wave, i.e., a V wave that is ≥ 2.5× the mean PCWP, or >50 mmHg, is usually secondary to MR. Figure 36.5 Typical deep X and deep Y descents on RA tracing, consistent with constrictive pericarditis but also with restrictive cardiomyopathy and severe RV failure. In severe RV failure, a large V wave with a flattened X descent may also be seen. V wave and Y descent become particularly prominent in inspiration (end of the tracing). Normally, mean RA pressure declines with inspiration. A flat RA pressure without significant inspiratory drop of the mean, as in this case, is characteristic of RV failure and constriction. Only the depth of Y descent changes with inspiration. In severe RV failure, RA and RV compliances are severely overwhelmed, such that RA pressure may paradoxically rise with the inspiratory rise in preload, despite the direct transmission of negative intrathoracic pressure. Reproduced with permission from Hanna and Glancy (2012).1 Figure 36.6 Deep X with flat Y on RA tracing, suggestive of tamponade. This may also be seen with sinus tachycardia, which shortens diastole and may thus attenuate Y descent. Reproduced with permission of Demos Medical from Hanna and Glancy (2012).1 Figure 36.7 Ventricularized RA pressure in a patient with severe TR. The V wave is not only ample but wide and peaks during the ST–T segment, similarly to the RV systolic pressure. Note that Y descent is deep but X descent is flattened. Compare to RV pressure in the same patient. Reproduced with permission of Demos Medical from Hanna and Glancy (2012).1 A large V wave may resemble PA pressure (Figures 36.8, 36.9). Five features help differentiate PCWP from PA pressure: Figure 36.8 On gross inspection of both figures, they may seem similar. In fact, (i) is the PCWP tracing of a patient with severe MR and gigantic V waves, while (ii) is his PA pressure tracing. Reproduced with permission of Demos Medical from Hanna and Glancy (2012).1 Keys to differentiate: Figure 36.9 (a, b) PCWP tracing shows a large V wave of ~38 mmHg, with a mean PCWP of 26 mmHg. The mean PCWP (left oblique arrow) is lower than the mean PA pressure (right oblique arrow), and is equal to the PA diastolic pressure (bar), all of which is consistent with a true PCWP. (c) Tracing C is recorded after inflation of the PA catheter balloon. It may mimic a PCWP tracing with a large V wave. However, the mean of this pressure tracing almost approximates the mean PA pressure and exceeds the PA diastolic pressure. Also, the timing does not fit with PA pressure: the presumed V waves peak as early as the PA pressure peaks, during the ECG T waves (vertical arrows), and have a sharp upslope, similar to the PA pressure upslope; the presumed A waves precede the ECG P waves. Thus, this is not a true PCWP tracing. The PA catheter is “underwedged,” which results in a hybrid PA–PCWP waveform. In severe pulmonary hypertension (PH), two issues arise. First, in severe PH, the segmental PA branches are dilated, which makes it difficult for the catheter to occlude these branches; thus, the wedged PA waveform may be a damped PA waveform and may overestimate the true PCWP (it is a hybrid PA–PCWP waveform with arterial rather than PCWP characteristics) (Figure 36.9). Second, a phasic PCWP depends on appropriate retrograde transmission of LA pressure through the pulmonary vasculature without any anatomical barrier; in case of severely elevated pulmonary arteriolar or venous resistance, retrograde transmission of LA pressure is attenuated, producing a damped and flattened PCWP that lacks distinct waves and descents. In the latter situation, mean PCWP may approximate mean LA pressure, but the waveform is flat and featureless and falsely creates or overestimates a transmitral gradient. In sum, the PCWP of PH may be a false PCWP (damped PA waveform), or a true PCWP that is, nonetheless, featureless (damped PCWP waveform). Other cases where PCWP lacks A and V waves and potentially overestimates the true PCWP: (i) catheter overwedging; (ii) catheter in the upper lung zone 1, where the pulmonary capillaries are collapsed by the alveolar pressure and where PCWP reflects alveolar pressure rather than capillary pressure (this false PCWP may exceed PA diastolic pressure). In patients with elevated PCWP, it is harder for the alveolar pressure to compress the pulmonary capillaries, and thus zone 1 significantly shrinks and is unlikely to be catheterized. LV diastolic pressure slightly increases throughout diastole, and, except in AF, has an A wave that corresponds to the atrial A wave. LVEDP is located at the downslope of the A wave. In normal individuals with compliant LV, LV pressure increases only slightly after A wave, so that post-A LV pressure is not significantly higher than pre-A LV pressure. In compensated LV dysfunction, LV pressure is normal throughout diastole but increases only after A wave; similarly, LA pressure is overall normal and increases only after A wave, explaining why mean LA pressure better correlates with pre-A LV pressure than with LVEDP. In decompensated LV dysfunction, LV pressure is high throughout diastole and increases further after A wave (Figure 36.10, Table 36.1). To identify LVEDP, search for a bump on the LV upstroke; the bump is A wave and the point that follows this bump is LVEDP (Figure 36.11). While LVEDP varies with respiration, the most accurate LVEDP is obtained when the respiratory pressure is 0 mmHg, which, unless the patient actively exhales, corresponds to end-expiration and coincides with the highest recorded LVEDP point (breath hold not recommended, due to the risk of inadvertent positive pressure strain). This end-expiratory rule applies to all measured pressures. Yet, in patients breathing deeply (e.g., obese), expiratory pressure is positive; the negative inspiratory and positive expiratory pressures cancel each other out, and averaging vascular pressures over several respiratory cycles is acceptable. Note: Normal hemodynamic values Figure 36.10 Diastolic superimposition of LA pressure (or PCWP, in blue) and LV pressure (in black). Downward arrows point to LVEDP. In compensated LV dysfunction, LV pressure is normal before A wave but increases after A wave (LVEDP). On PCWP tracing, V wave is normal, A wave is increased, but mean PCWP is overall normal and better correlates with the pre-A LV pressure than the elevated LVEDP (PCWP < LVEDP). In decompensated LV dysfunction, both pre-A and post-A LV pressures are elevated. On PCWP tracing, V wave is large, and mean PCWP is equal to LVEDP or larger than LVEDP, depending on how large V wave is. Table 36.1 Correlation between LVEDP and mean PCWP. Figure 36.11 LVEDP corresponds to the bump seen on the LV upstroke (arrows), often coinciding with the peak of R wave on the ECG (LVEDP may be further delayed from R peak in case of long tubing between catheter and transducer). This bump is LV A wave. In this figure, LV pressure steeply increases during and after A wave, leading to a pronounced A wave and a high LVEDP of 32 mmHg. LVEDP does not correspond to the flat/plateau portion of LV diastole. Always look for these bumps when assessing LVEDP, as long as the rhythm is sinus. Reproduced with permission of Demos Medical from Hanna and Glancy (2012).1 Table 36.2 Fick equation. SaO2 and SvO2 are expressed as decimal fractions. In the absence of a left-to-right shunt, SvO 2 corresponds to O 2 saturation of the PA, where the venous blood achieves its best mixing from all sources (SVC, IVC, coronary sinus); sampling of the RA may selectively capture one of these three sources rather than their mixture. True arterial oxygen content is the amount of oxygen bound to hemoglobin plus the amount of oxygen dissolved in arterial blood. The latter is negligible (~1.5%) and is ignored in this simplified equation, unless under high flow of O2 and high PaO2. CO, cardiac output; Hb, hemoglobin; SaO2, arterial O2 saturation (transcutaneous O2 saturation may be used instead of SaO2 if an arterial sheath has not been placed); SvO2, mixed venous O2 saturation. The cardiac output may be measured using three different methods: (1) true Fick method, which is the most accurate method; (2) assumed Fick method; (3) thermodilution. The simplified Fick equation is shown in Table 36.2. Short of truly measuring it, O2 consumption may be assumed to be 125 ml/min/m2 of BSA or 3 ml/min/kg at rest. This assumption is subject to a large variation between individuals and within the same individual depending on the degree of wakefulness and anxiety at different times during the procedure. This assumption may be associated with discrepancies of 25% or more in comparison with the true Fick method. Thus, using 125 ml/min/m2 of BSA may underestimate CO in a fully awake anxious or agitated young patient, or a septic patient with elevated O2 consumption, and may overestimate CO in elderly patients, in heart failure patients who have reduced O2 consumption, and in obese patients (the formula uses true body weight and surface area, which overestimates O2 consumption of the obese). In HF, O2 consumption is mainly limited during exercise, but may be limited at rest in severe class III/IV HFrEF, wherein the equation over- estimates CO by ≥25% in ~20% of patients. Aside from O2 consumption, the equation itself is subject to error in patients receiving excessive O2 therapy, that is if SaO2 is >90% at baseline or becomes ~100%, as O2 increases SvO2 disproportionately to SaO2 and leads to overestimation of cardiac output (compared to SaO2, SvO2 is on a steeper portion of the O2–hemoglobin dissociation curve, which explains the sharper rise with O2 therapy). Arterial O2 delivery increases with O2 therapy, partly through an increase in the dissolved, non-hemoglobin-bound O2 content which is trivial at baseline, but this is not reflected in SaO2 and the simplified Fick equation. Thermodilution is measured using a PA catheter that has a thermistor at its distal tip. After the catheter is positioned in the PA, 10 ml of cold (room temperature) saline is injected instantaneously through the blue RA port. The thermistor analyzes how quickly the temperature drops as blood reaches the PA and how quickly it recovers. The higher the cardiac output, the more brief and sharp the temperature change will be with a small area under the curve. The colder the injectate, and the more instantaneously it reaches the RA, the more accurate the measured cardiac output. Usually three measurements are obtained to average the slight variability of cardiac output (~10%) that occurs with various levels of wakefulness. When used for valve area calculation, the cardiac output and pressure gradient should be measured almost simultaneously to account for this variation. This method is valid in most cases, and has <5–10% error in comparison with the true Fick method, except in TR, low cardiac output, and right-to-left or left-to-right shunt:
36
Hemodynamics
I. Right heart catheter (see Figure 36.1)
II. Overview of pressure tracings: differences between atrial, ventricular, and arterial tracings (Figures 36.2, 36.3, 36.4)
III. RA pressure abnormalities
IV. Pulmonary capillary wedge pressure (PCWP) abnormalities
A. Differential diagnosis of a large V wave
B. Differentiate a large V wave from PA pressure
C. Case of pulmonary hypertension: differentiate PCWP from a damped PA pressure
V. LVEDP
In MS, end-diastolic PCWP is higher than LVEDP, whereas in MR or LV failure with a large V wave, end-diastolic PCWP remains equal to LVEDP, but mean PCWP is larger than LVEDP (driven by the large V wave).
O2 consumption (ml O2/min)
⇒ CO (liters blood/min)
⇒ CO
VI. Cardiac output and vascular resistances
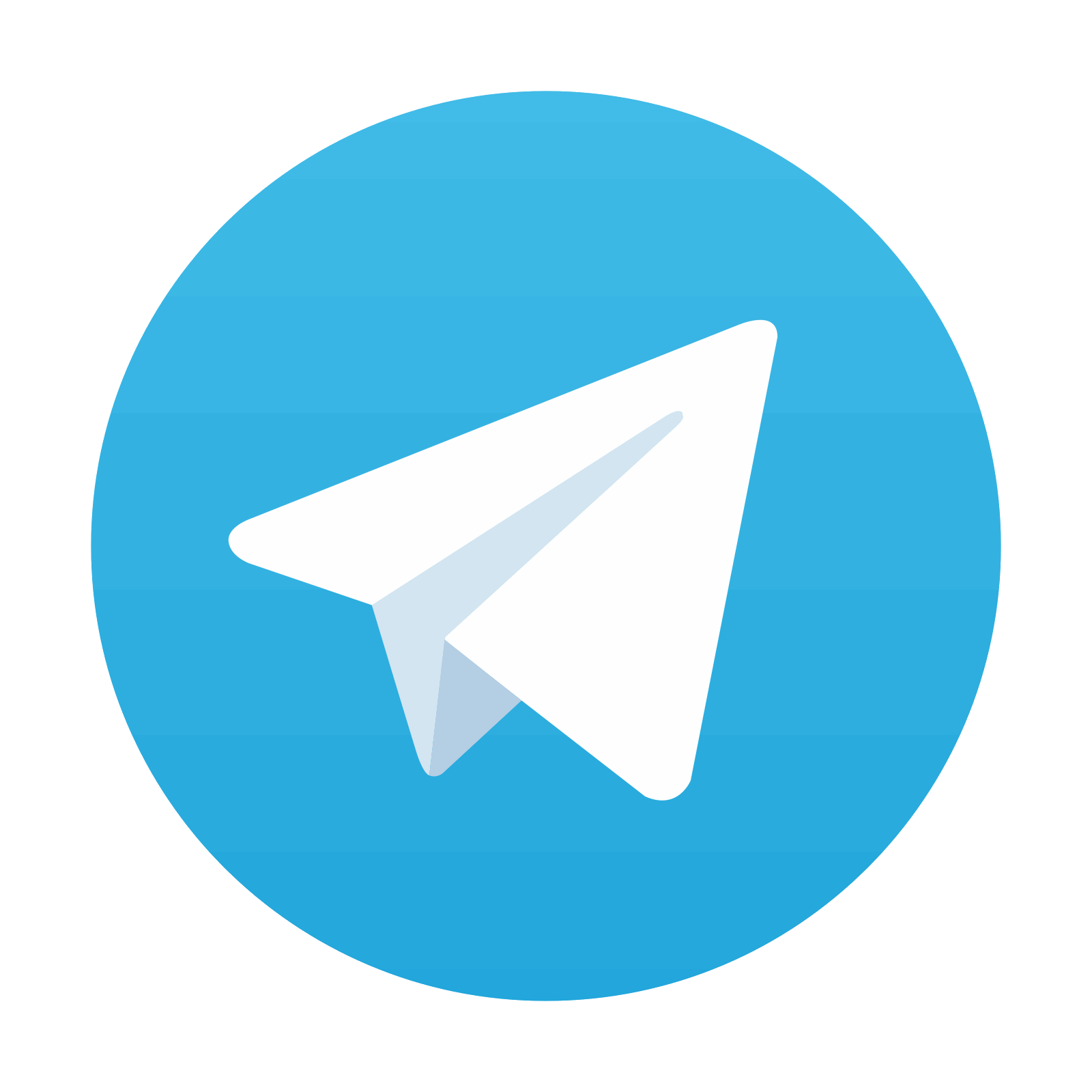
Stay updated, free articles. Join our Telegram channel

Full access? Get Clinical Tree
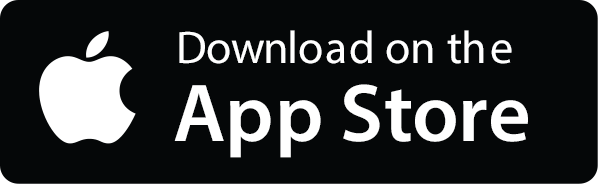
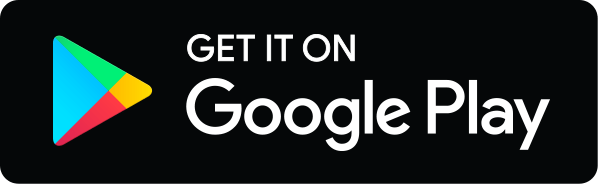