Fig. 11.1
Forssmann, Richards, and Cournand receiving the Nobel Prize in Physiology. From left to right: Richards, Forssmann Cournand, and Professor Friberg. From Werner Forssmann: A Pioneer of Cardiology Original Journal: The American Journal of Cardiology, Volume 79, Issue 5, 1 March 1997, Pages 651–660, Fig. 4. Renate Forssmann-Falck
Today, the right heart catheterization (RHC) is still the gold standard for the hemodynamic evaluation of the right ventricle (RV) and pulmonary circulation. During a RHC, right atrial pressure (RAP), right ventricular pressure (RVP), pulmonary artery pressure (PAP), and pulmonary arterial wedge pressure (PAWP) are measured and recorded. RHC can also be used to measure flow [cardiac output (CO)] using thermodilution or (in)direct Fick methods. In addition, systemic sampling of blood to determine oxygen saturations is indispensable for the evaluation of systemic-to-pulmonary shunting.
Very important for a correct hemodynamic evaluation of patients is a precise and standardized RHC procedure [2]. Kovacs et al. [3] showed that the choice of different zero reference levels strongly influenced pressure measurements. It has been recommended to set the zero reference level at the level of the right atrium. Evaluation with CT scans showed that the height of the right atrium was best estimated when the zero reference level was set at 1/3 of the thoracic diameter [3]. The RHC is a relatively safe procedure and when performed in an experienced center, morbidity (1.1 %) and mortality (0.055 %) rates are low [4].
Mean Pulmonary Arterial Pressure
Shortly after the clinical introduction of the RHC technique, it became clear that elevated pulmonary vascular pressures were related to symptoms of dyspnea and fatigue. Paul Wood defined in 1958 a mean pulmonary artery pressure (mPAP) of 25 mmHg as the upper limit of normal on the basis of measurements performed in 60 healthy subjects [5]. The same definition of pulmonary hypertension (PH) is still used today and the RHC remains the gold standard for the diagnosis of PH [2].
Wood’s studies using acetylcholine in PH patients also contributed to the classification of PH (Fig. 11.2), which has been modified several times in the last five decades. Approximately 40 causes of PH are now recognized, which are categorized in 5 main groups (Fig. 11.3). Left-sided heart failure (WHO group 2) is the most common cause of PH [2].



Fig. 11.2
Reaction of the pulmonary and systemic circulation to acetylcholine in a patient with “primary pulmonary hypertension.” Using acetylcholine as a pulmonary vasodilator, Paul Wood showed in patients with “Primary pulmonary hypertension” that the administration of acetylcholine led to a decrease in pulmonary artery pressure in combination with an increase in cardiac output and systemic blood pressure proving the increased vasoconstriction in this disease. From Wood: Pulmonary hypertension with special reference to the vasoconstrictive factor. Journal: Br Heart J 1958;20:557–570, Fig. 8

Fig. 11.3
Five main groups of pulmonary hypertension
Since the cardiovascular system is a closed loop system, different (patho)physiological hemodynamic changes can initiate an increase in mPAP. The hemodynamic mechanisms resulting in an increase in mPAP are: an increase in pulmonary vascular resistance (PVR), an increase in PAWP, and an increase in cardiac output (CO).
Pulmonary Vascular Resistance
An increase in the afterload of the RV leads to an increase in mPAP since the RV needs to build up more pressure to maintain an adequate CO. The load on the outflow of the RV can be divided into the resistance to steady state flow and the resistance to pulsatile flow from vascular impedance [6]. The resistance to steady state flow is known as the PVR and is defined as PVR = (mPAP − PAWP)/CO. An increase in PVR can be due to a decrease in the pulmonary vessel radius or due to the loss of arterial surface [6]. A decrease in pulmonary arterial vessel radius is seen in WHO group 1 and 4 PH patients and is due to thickening of the vascular wall, intravascular occlusions (either by exuberantly proliferating cells of the vessel wall or thrombosis and emboli), and loss of vessel number (rarefaction). Hypoxic vasoconstriction, also seen in WHO group 3 PH patients can contribute to a decrease in the pulmonary arterial diameter. A decrease in arterial surface area is often observed in emphysema. Vascular rarefaction as the sole cause of an increased PVR is still a matter of debate, since even in severe emphysema, PH is rare. PVR can also be increased in conditions associated with an increased blood viscosity.
Resistance to pulsatile flow is mostly described by an inverse measure, the pulmonary arterial compliance. Pulmonary arterial compliance is assessed by stroke volume (SV) divided by the pulse pressure (PP) (SV/PP). It has been shown that in the pulmonary circulation PVR and compliance are inversely related [7]. The product of PVR and compliance, known as the RC time (T ), can be calculated as T = PVR × compliance = ((mPAP − PAWP)/(SV × HR)) × (SV/PP) = T ×((mPAP − PAWP)/PP). Over a wide range of PVR, T remains relatively stable in healthy people and patients with precapillary PH [7–9].
The increase in afterload in PH requires the RV to generate more hydraulic power to maintain adequate CO. The power generated by the RV can be divided into mean (or steady) power and oscillatory (or pulsatile) power. Mean flow determines transport and is mainly determined by mean power. Oscillatory power is generated for the pulsatile component of flow. It has been shown that the ratio between mean power and oscillatory power generated by the RV is equal among healthy people and PAH patients and that oscillatory power counts for approximately 23 % of total power generated by the RV [10]. Since mean power is defined as mPAP × CO, mean power, total hydraulic power (1.3 × mean power), and oscillatory power (0.23 × total hydraulic power) can be derived from RHC measurements. Mean power is related to mPAP and therefore mean power is also related to steady state resistance (PVR) and oscillatory power is related to pulse pressure and therefore related to arterial compliance. Consequently, the constant power fractions reflect the constant RC time [7–10].
Pulmonary Arterial Wedge Pressure
mPAP can also be increased due to an increased PAWP. During RHC, a balloon can be inflated to temporarily close a small pulmonary artery branch. The pressure proximal from the inflated balloon is the PAWP and is a surrogate measure of the pressure in the postcapillary system including the left atrial pressure (LAP). Left heart failure or left-sided valvular disease results in an increase in PAWP, which can subsequently increase mPAP. A PAWP >15 mmHg is defined as abnormal and is due to left heart disease. Therefore, PH can be classified in pre- and postcapillary PH based on the PAWP [2]. The significance of a PAWP between 12 and 15 mmHg is still unclear.
In left heart disease, the elevation of the mPAP is proportional to the increase in PAWP and the PVR is often normal. An elevated PVR in combination with an increase in PAWP is seen in approximately 25 % of patients with PH due to left heart failure and has been described as “out of proportion” PH [11].
When using the PAWP to classify the PH, some shortcomings of PAWP should be taken into account. PAWP is dependent on the volume status and can be falsely low in WHO group 2 PH patients on diuretic therapy. Rapid fluid infusion or exercise testing can increase the PAWP in WHO group 2 patients and can reveal left heart disease. PAWP can also be falsely high in patients with precapillary PH, since severe precapillary PH with RV dilatation, congestion, and increased RAP can cause diastolic pressures to be transmitted across the septum causing increased left-sided filling pressures [11, 12].
Another way to distinguish pre- from postcapillary PH is to use the transpulmonary gradient (TPG) defined as TPG = mPAP − PAWP, with a TPG >12 mmHg as a threshold for precapillary PH. However, the calculation of the TPG can be misleading, because an increase in CO can lead to an increase in distensibility of pulmonary vessels, lowering mPAP and the TPG for any given flow. An increase in distensibility also lowers the slope of the mPAP–PAWP relation, falsely decreasing TPG with an increase in PAWP. Moreover, the mPAP–PAWP relation is sensitive to changes in SV and arterial compliance. An increase in SV will lead to an increase in mPAP for any given PAWP and therefore increasing the TPG [13].
Although the RC time of the pulmonary circulation in healthy subjects is stable and patients with precapillary PH, a recent study showed that this may not be true in patients with postcapillary PH [9]. The upstream load of an increased PAWP causes a relatively greater decrease in compliance than an increase in PVR, subsequently increasing the TPG.
It has been suggested that the diastolic pressure gradient (DPG), defined as diastolic pulmonary artery pressure (dPAP) minus PAWP (=dPAP − PAWP), is more accurate when it comes to distinguishing pre- from postcapillary PH, since dPAP is less sensitive to changes in SV and arterial compliance [13].
Cardiac Output
The degree to which an increase in CO leads to an increase in mPAP depends on the degree to which lung vessels can distend and be recruited. The magnitude of vascular distention and lung vascular recruitment during exercise is still hotly debated. During exercise, an increased demand for oxygen will increase CO, which is usually followed by at least some increase in the mPAP [14]. Pathophysiologic changes of the slope of the mPAP–CO relation during exercise will be further discussed in the exercise section below. Conditions like congenital heart disease, hyperthyroidism, portal hypertension, and congenital portosystemic venous shunts can also increase CO and mPAP [15–17]. Nevertheless, overwhelmingly patients with PH have a decreased CO as a result of the increase in PVR and RV failure.
Usage of Hemodynamics in Clinical Practice and Research
As already mentioned, RHC is the gold standard for the diagnosis of PH and PH is defined as mPAP >25 mmHg. To distinguish pre- from postcapillary PH, the hemodynamics are further evaluated on the basis of the PAWP, TPG, and DPG, not only at baseline but preferably also after a fluid challenge or during exercise. A proper classification is of major importance, since pre- and postcapillary PH are managed quite differently [2] (Table 11.1).
Table 11.1
Diagnostic reference values of hemodynamic parameters
Normal (mmHg) | Precapillary PH (mmHg) | Postcapillary PH (mmHg) | |
---|---|---|---|
mPAP | <25 | >25 | >25 |
PAWP | <15 | <15 | >15 |
TPG | <12 | >12 | <12 |
DPG | <5 | >5 | <5 |
Current PH guidelines advise, on a non-consensus basis, to repeat the RHC 12–16 weeks after initiating or changing PH treatment, once a year in stable patients or when signs of clinical worsening are present. Neither the baseline mPAP nor changes in mPAP during treatment have a prognostic value in PH patients [18–20]. In precapillary PH, baseline PVR has prognostic value and an increased PVR at baseline is associated with a worse survival [18]. Treatment-related changes in PVR do not predict survival or a change in RV function [20]. Only when a reduction in PVR is very substantial, as is seen after a pulmonary thrombendarterectomy for chronic thromboembolic PH (CTEPH), the decrease in PVR is accompanied by an improved RV function.
Baseline CO and cardiac index (CI = CO corrected for body surface area) are of prognostic value in PH [18, 19]. The PH guidelines describe profiles of outcome based on multiple prognostic parameters including CI, with a CI < 2.5 L/min/m2 predicting a worse prognosis. Interestingly, at the time of the writing of the guidelines, there was little scientific basis for a cutoff value of CI < 2.5 L/min/m2. A recent study in IPAH patients showed that a baseline CI > 2.5 L/min/m2 combined with a CI > 2.5 L/min/m2 follow-up was associated with the best survival. Survival rates of patients with a baseline CI < 2.5 L/min/m2 were almost as good, provided their CI after follow-up was greater than >2.5 L/min/m2. Poor survival was observed when follow-up measurements showed a CI < 2.5 L/min/m2, with little difference between patients with a high or low baseline CI. This suggests that not only baseline CI but also the change in CI to treatment is important in the evaluation of patients [21].
Exercise Testing
Pathophysiology
During exercise, the cardiopulmonary system is pushed to its upper limits. The increased demand for oxygen delivery to the tissues is met by an almost fourfold increase in CO. The entire CO passes through the pulmonary circulation and the pulmonary circulation prevents a proportional increase in mPAP by vasodilatation and vessel recruitment [22]. In other words, the slope of the mPAP–CO relationship is determined by the magnitude of vascular recruitment and distention.
PH patients often complain of exercise-induced dyspnea and this impaired exercise tolerance is mainly due to circulatory limitations. Exercise-induced dyspnea in PH patients is primarily due to the inability to increase pulmonary blood flow during exercise. This limited increase in CO is caused by an inability to increase SV and due to an abnormal chronotropic response [23, 24]. The inability to increase SV reflects an increased end-systolic volume (ESV) as a result of the increase in PVR and RV dysfunction [23, 25]. An abnormal chronotropic response is demonstrated as a decreased maximal heart rate (HR) in PH and is believed to be the result of an imbalance of the autonomic nervous system [24, 26–28]. The decrease in CO for a given workload results in insufficient oxygen delivery to peripheral tissues, anaerobic metabolism of glucose, and muscle weakness due to acidosis [29]. Together these changes limit the maximal uptake of oxygen (VO2max) and result in early exercise termination.
A second problem in PH is an increased ventilatory requirement, which is still poorly understood, but likely contributes to the patients’ sensation of dyspnea. In precapillary PH, dead space ventilation occurs because of hypoperfusion of normally ventilated alveoli due to loss of the pulmonary capillary bed. Dead space ventilation could lead to problems eliminating carbon dioxide, increasing minute ventilation (VE). However, because PH patients are often hypocapnic at rest and during exercise, it is assumed that in addition to dead space ventilation, alveolar hyperventilation further contributes to the increased ventilatory requirement [30]. It is believed that exercise-induced alveolar hyperventilation in PH is due to the summation of lactate acidosis, a hypoxemic ventilatory drive, and a sympathetic nerve overdrive. In summary, ventilatory inefficiency is a hallmark of PH and may contribute to the sensation of dyspnea [25, 26, 29].
Finally, diaphragm weakness can lead to a further increase of breathlessness during exercise [31].
To evaluate a patient’s exercise capacity and exercise-related symptoms, both the 6 min walk test (6MWT) and the maximal cardiopulmonary exercise test (CPET) are used.
Six Minute Walk Test
The 6MWT is a relatively simple and inexpensive tool to measure exercise tolerance. It measures the distance that a patient can walk on a flat floor in 6 min. The 6MWT is a measure of the integrated response of all physiological systems that are necessary to perform work. The SV response and chronotropic response to exercise are found to be the most important factors influencing the 6MWT [27].
The 6MWT is adapted from the Cooper test and is used as a surrogate for maximal oxygen consumption (VO2max). Via the Fick’s equation (VO2 = CO × arteriovenous oxygen difference), the 6MWT is associated with CO (and, hence, SV and heart rate). A limitation of the 6MWT is a ceiling effect in relatively healthy individuals, since in these individuals the increase in distance during the 6MWT does not follow the increase in VO2max [32].
6MWT in Clinical Practice and Research
In precapillary PH, baseline 6MWT is a strong predictor of survival and a baseline distance of >440 meters is associated with a better survival [18, 19]. It is found that the minimal important difference (MID) in 6 min walk distance to get a change in health-related quality of life score is 41 m [33]. This is in accordance to a recent meta-analysis using the occurrence of clinical events to assess the MID of the change in 6 min walking distance (Δ6MWD) (41.8 m) [34].
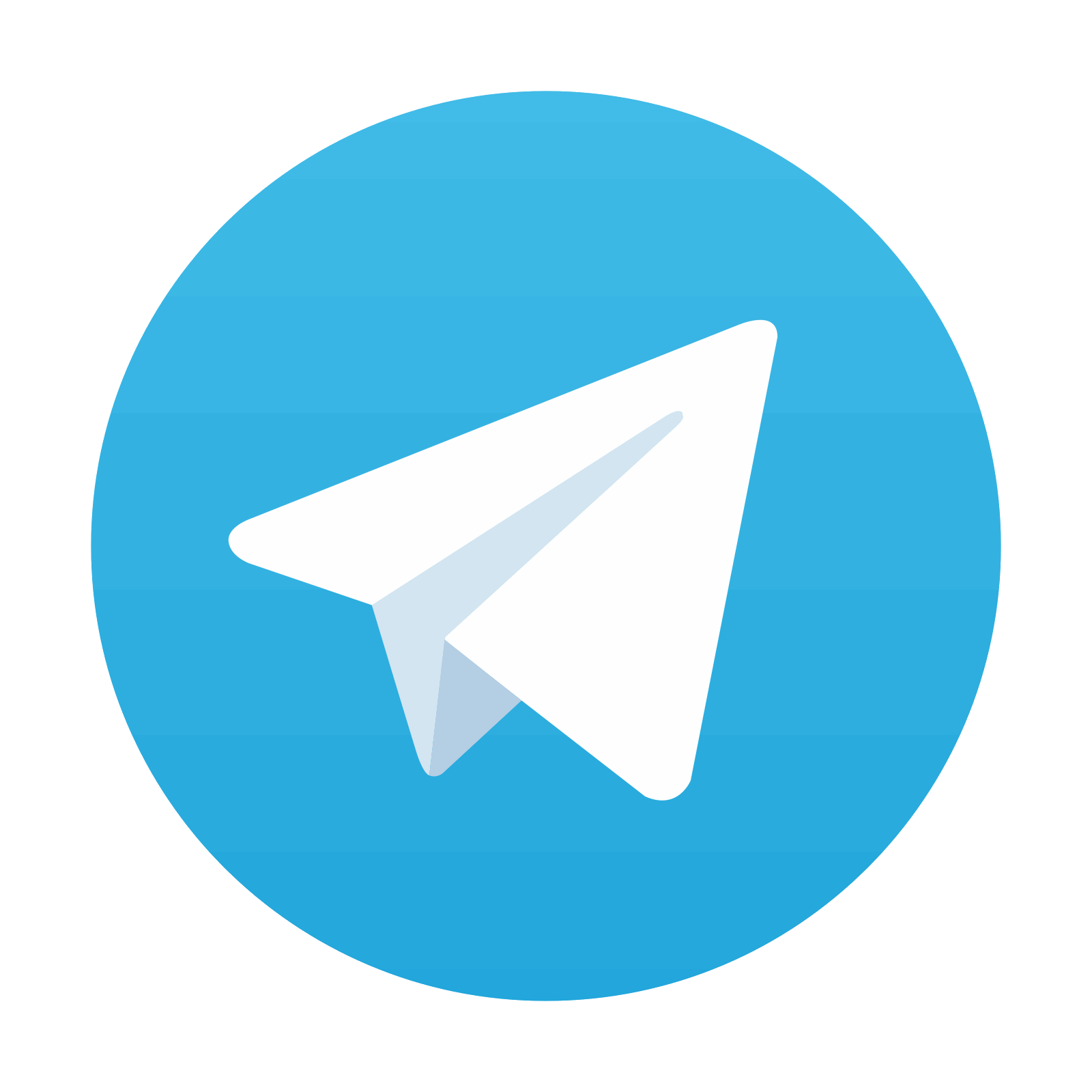
Stay updated, free articles. Join our Telegram channel

Full access? Get Clinical Tree
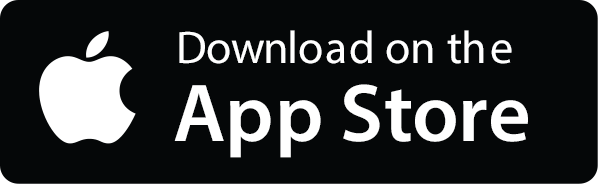
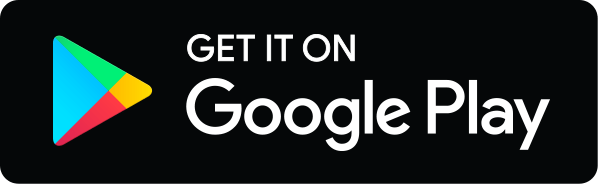