Fig. 32.1
Deposition of C4d and C3d in biopsy sections of the cardiac allograft with AMR. (a) Immunofluorescence techniques reveal C4d deposition in capillaries of biopsy sections of the cardiac allograft. (b) Immunofluorescence techniques reveal C3d deposition in capillaries of biopsy sections of the cardiac allograft (a)
Required findings | Optional | |
---|---|---|
1. Clinical evidence of acute graft dysfunction | Recommended in combination with other evidence to support diagnosis of AMR | |
2. Histological evidence of acute capillary injury (a and b required) | (a) Capillary endothelial changes | (c) Neutrophils in capillaries (severe) |
(b) Macrophages in capillaries | (d) Interstitial edema/hemorrhage (severe) | |
3. Immunopathologic evidence for antibody- mediated injury (a or b or c required) | (a) IgG, IgM, and/or IgA + C3d and/or C4d or C1q (2–3+) by IF | |
(b) CD 68 for macrophages in capillaries (CD31 or CD34) and/or C4d (2–3+ intensity) in capillaries by paraffin IH | ||
(c) Fibrin in vessels (severe) | ||
4. Serological evidence of anti-HLA or anti-donor antibodies | Anti-HLA class I and/or class II or other anti-donor antibody at time of biopsy (supportive of clinical and/or morphological findings) |
Endomyocardial biopsy | Circulating antibody | |
---|---|---|
Methodology | Histological evaluation | Solid-phase assay and/or cell-based assays to assess for presence of DSA (and quantification if antibody present) |
Immunoperoxidase: C4d | ||
Immunofluorescent staining: C4d and C3d | ||
Intervals | Histological evaluation of every protocol biopsy | 2 weeks and 1, 3, 6, and 12 months, and then annually after transplantation |
Immunoperoxidase/immunofluorescent staining: | When AMR is clinically suspected | |
2 weeks and 1, 3, 6, and 12 months after transplantation | ||
When AMR is suspected on the basis of histological, serological, or clinical findings routine C4d (C3d) staining on subsequent biopsy specimens after a positive result until clearance |
Intravascular macrophages are pathognomonic for AMR, and the macrophage antigen CD68 can be used to distinguish AMR from cellular rejection by the demonstration of intravascular/perivascular macrophages and differentiation from lymphocytes. Further, the endothelial cell markers, CD34 and CD31, can delineate endothelial damage and intravascular location of macrophages [24].
Antibodies
Both HLA and non-HLA antibodies have been implicated in immune-mediated injury following heart transplantation. The development of HLA antibodies, particularly Class II antibodies, after transplantation has been shown to be associated with cardiac allograft vasculopathy, cellular rejection, and mortality. Furthermore, HLA antibodies directed against the donor appear to be associated with AMR in addition to cellular rejection and CAV. Non-HLA antibodies may also cause immune injury and can be present in the absence of HLA antibodies.
Clinical Features Associated with Symptomatic AMR
Patients with AMR typically present with signs and symptoms of heart failure due to right and/or left systolic or diastolic dysfunction (◘ Fig. 32.2). These clinical symptoms include: shortness of breath, orthopnea, paroxysmal nocturnal dyspnea, elevated neck veins, ascites, and peripheral edema. Acute AMR may result in hemodynamic compromise and is observed in more than 40 % of the patients [6, 15, 24–26]. Even the definition of hemodynamic compromise can be unclear and may range from a decrease in left ventricular ejection fraction to an unexplained elevation in intracardiac pressures with reduced cardiac output and the need for inotropic therapy.


Fig. 32.2
Progression of AMR ultimately results in graft failure. Schematic highlighting subclinical, clinical, and chronic disease associated with AMR (adapted from [22])
Clinical Features Associated with Subclinical AMR
Although the definition of AMR has included clinical evidence of graft dysfunction in addition to histological and immunological derangements, it has been demonstrated that complement activation may be observed in the absence of kidney or heart allograft dysfunction (◘ Fig. 32.2). Thus, the term subclinical AMR may be used to characterize these patients [27–29]. Moreover, complement deposition without graft dysfunction may be observed in accommodation, a state believed to be achieved after complement regulatory proteins successfully terminate the activated complement cascade in an attempt to achieve a state of accommodation [5]. Therefore, in a subpopulation of heart transplant recipients, complement deposition without perturbed allograft organ function represents accommodation. The induction of regulators of complement activation within the tissue concomitant with C4d deposition supports this concept [30]. Whether subclinical AMR should be treated remains unclear. There are data, however, that support the association of subclinical AMR with adverse outcomes. Wu et al. examined heart transplant recipients with subclinical AMR, heart transplant recipients that were treated for AMR and had LV dysfunction and a control group of heart transplant patients without AMR [31, 32]. Using a rigorous definition for AMR that included histology and immunohistochemistry, the 5-year survival for the subclinical AMR group, the treated AMR group and control group demonstrated no significant difference. The patients with subclinical AMR had significantly less freedom from cardiac allograft vasculopathy than the control group and similar outcomes to patients with treated symptomatic AMR. Patients with subclinical AMR have been reported to have a worse cardiovascular mortality compared to patients with acute cellular rejection and comparable mortality to those with mixed (ACR and AMR) rejection [33]. Therefore, this study supports the notion that subclinical AMR is associated with a poor outcome .
Diagnosis of AMR
In 2010, a committee and conference sponsored by the ISHLT was assembled to further the understanding of AMR. The committee and conference included transplant cardiologists, surgeons, pathologists, and immunologists. The conference discussed and defined AMR, the significance of subclinical AMR, and the contribution of donor specific antibodies (HLA and non-HLA antibodies). Although previous revisions to the definition of rejection have not recommended screening for AMR, the screening for AMR and antibodies were recommended by this committee and conference. The criteria for the diagnosis of AMR were outlined in a published scientific statement [34]. The proposed scoring system encompassed histopathology, immunohistochemistry, and immunofluorescence techniques and established the nomenclature for the diagnosis of pathologic AMR. This scoring system was based on the fact that although clinical features were taken into account, there was a definition for pathologic cellular rejection but none existed for AMR. The classification of AMR was published in 2011 and more recently in 2013 (◘ Tables 32.3 and 32.4).
Positive biopsy | Immunohistochemistry | Immunofluorescence |
---|---|---|
Capillary distribution and intensity | Multifocal/diffuse weak or strong staining of C4d | Multifocal/diffuse weak or strong staining of C4d/C3d |
Intravascular CD68 distribution | >10 % Focal/multifocal/diffuse Intravascular macrophages | – |
HLA-DR distribution and intensity | – | Multifocal/diffuse weak or strong staining |
Caveats | Focal strong C4d staining is classified as negative but warrants close follow-up | Focal strong C4d staining is classified as negative but warrants close follow-up |
Category | Description |
---|---|
pAMR 0: Negative for pathological AMR | Both histological and immunopathologic studies are negative |
pAMR 1 (H+): Histopathologic AMR alone | Histological findings present immunopathologic findings negative |
pAMR 1 (l+): Immunopathologic AMR alone | Histological findings negative and immunopathologic findings positive |
pAMR 2: Pathological AMR | Both histological and immunopathologic findings are present |
pAMR 3: Severe pathological AMR | Severe AMR with histopathologic findings of interstitial hemorrhage, capillary fragmentation, mixed inflammatory infiltrates, endothelial cell pyknosis and/or karyorrhexis, and marked edema |
While the field has advanced by the refinement of the definition of pathologic AMR, it is important to take into account the clinical presentation: symptoms, hemodynamics, and graft function. In areas where there is little information regarding treatment, the clinical presentation may be the determining factor. Finally, DSAs may also influence the decision to treat AMR. There are multiple classifications of AMR that will require decision-making by the clinician.
Imaging Modalities
Although the gold standard for diagnosing AMR is the endomyocardial biopsy, imaging may provide important clues as to the status of graft function and a potential immunological cause. Unfortunately, most imaging studies have focused on cellular rejection and there are limited data regarding their use for AMR. Left ventricular diastolic dysfunction can be one of the earliest features of acute rejection [35–38]. In order to increase the sensitivity of the imaging modality, cardiac magnetic resonance imaging (CMR) is increasingly used for the diagnosis of AMR as it is able to assay myocardial edema and increases in LV mass [39]. For example, T2 quantification is an important modality in CMR. As T2 relaxation time is the decay time constant of the magnetic signal following an excitatory pulse studies demonstrate that T2 relaxation time lengthens with the degree of myocardial edema. Of all the CMR parameters, T2 quantification correlates best with biopsy proven AMR. T2 quantification remains investigational, and future studies will be necessary to determine the role of noninvasive imaging studies for the diagnosis of AMR [40].
Serologic Markers of Rejection
B-type natriuretic peptide (BNP) has been shown to be associated with acute rejection in heart transplant recipients. Changes in BNP over time are correlated with acute rejection as opposed to specific levels. In a series of 146 patients, there was only a marginal association between nt-BNP and rejection; for every 100 pg/ml increase in nt-BNP, there was only a 1 % increase in the risk of rejection; however, a twofold increase in nt-BNP was associated with an odds of 2.4, a fivefold increase associated with odds of 6.8, and a tenfold increase associated with an odds of 21.6, after adjusting for decrease in EF and rise in PCWP [41]. Wu and colleagues demonstrated that the log BNP was associated with a greater than fivefold increase in the risk of vascular rejection [42]. Another commonly used serological marker for rejection and the detection of cardiac injury is troponin. Routinely, cardiac troponin I (cTnI) is monitored during the early stages of rejection and the response to treatment. Finally, the gene expression profile (AlloMap ) has been shown to reliably exclude acute cellular rejection; however, it has not been demonstrated to be useful in excluding AMR [43].
Pathology
The diagnosis of acute cellular rejection is made solely on the basis of pathologic findings. AMR, however, has typically relied mainly on clinical findings due to the lack of pathologic criteria. The definition of AMR published in 2009 required the presence of graft dysfunction and/or circulating donor specific antibodies in addition to the histological and immunopathological findings. These criteria were removed during the Consensus Conference in 2010. Thus a pathological definition of AMR was established. In this definition, the criteria for establishing a positive diagnosis was established (◘ Table 32.1). C4d and C3d staining are scored based on distribution of staining within the capillaries (negative, focal, or multifocal/diffuse) and the intensity of staining (negative, trace, strong) (◘ Table 32.3). In addition, HLA staining is recommended for assessment by immunofluorescence and CD68 with immunohistochemical techniques. Other markers are considered optional. Although immunofluorescence is considered the gold standard, immunohistochemistry is used by 80 % of US and European centers. During the consensus conference, both methods were evaluated. There appeared to be good reproducibility between centers using immunohistochemistry and also good correlation between immunofluorescence and immunohistochemical techniques. Immunopathology assays rely on processed tissue (fresh frozen or paraffin infiltrated and embedded) and the detection of specific antigens by using specific antibodies. Tissue (endomyocardial biopsy specimens) that is fresh frozen and sectioned typically uses immunofluorescence protocols and primary antisera directed against C4d, C3d, immunoglobulin heavy chains, fibrin, HLA-DR, and CD55. These primary antibodies are then detected using a fluorophore conjugated secondary antibody. In contrast, the detection of more stable antigens (that can withstand the paraffin processing) use an immunoperoxidase detection system to examine for the expression of C4d, CD68, C3d, CD34, CD31, CD3, and CD20. Each mode of processing (frozen vs. paraffin processing) has advantages and disadvantages. For example, the immunoperoxidase protocol uses paraffin processing which is commonly available in pathology laboratories and yields superior morphology for the analysis of both complement deposition and the surrounding tissue (i.e., analysis of the endothelium and the myocardium). In addition, the immunoperoxidase-stained sections/slides provide a permanent archival slide record that can be stored at room temperature for long periods of time. In contrast, the immunofluorescence detection method relies on the freezing of the tissue which has the advantage of rapid processing and improved antigen preservation with decreased nonspecific signal. Moreover, residual frozen tissue can also be a valuable resource for viral polymerase chain reaction or other molecular tests. The challenges with the immunofluorescence technique are the need for technical expertise to obtain high-quality frozen sections and the inability to have a permanent archival slide record as the immunofluorescent signal fades over time.
Comparison of the results using both the immunofluorescence and the immunoperoxidase staining techniques is important for the diagnosis of AMR. For example, C4d expression from endomyocardial fragments obtained at the same biopsy procedure can equally detect C4d expression using either the immunofluorescence of the immunoperoxidase technique [29, 44, 45] (◘ Fig. 32.1). Using the immunoperoxidase technique, Fedrigo and colleagues observed that C4d capillary staining was present in about 35 % of heart transplant recipients, and AMR (using the 2005 ISHLT criteria) was present in 7 % of heart transplant recipients [27]. Over a median follow-up period of approximately 2 years, cardiac transplant recipients who were C4d-positive experienced higher mortality than those that were C4d-negative, regardless of graft function. In contrast, C4d positivity that is detected only by immunofluorescence is of unclear significance. The immunofluorescent coexpression of C3d and C4d significantly enhances the diagnosis of AMR. For example, Tan et al. [4] demonstrated that endomyocardial biopsy specimens from cardiac transplant recipients having linear capillary deposition of C4d with C3d deposition was associated with allograft dysfunction (in more than 80 % of the patients) and the incidence of allograft dysfunction was more than 90 % with the presence of DSA. Only one patient with C4d staining alone (C3d negative) had concurrent allograft dysfunction. This result supports the notion that the immunofluorescent detection of C4d alone is not a specific indicator of AMR. In addition, reperfusion injury and viral infections have been shown to cause C4d deposition, but may not be associated with AMR or a poor prognosis. In summary, the diagnosis of AMR using the immunofluorescence technique should include both C3d and C4d deposition, whereas the use of the immunoperoxidase protocol requires only the expression of C4d. Importantly, it is essential to have the expertise of a dedicated cardiac pathologist to ensure timely and consistent reporting of the results to the transplant team. Areas that still require clarification include the transition to AMR, appropriate monitoring strategies, the role of serial biopsies, and the significance of late appearing DSAs .
The working formulation for pathologic criteria for AMR range from pAMR 0 to pAMR 3 based on the presence or absence of histological and immunopathological features (◘ ◘ Table 32.4 and ◘ Fig. 32.3). Routine surveillance is recommended. Evaluation during the first 2 weeks after transplant may be confounded by preoperative changes; however, two samples (2 and 4 weeks) are recommended during the first month after transplant. Monitoring following a positive result should continue until clearance although it is not clear when clearance occurs. One study using immunofluorescence techniques suggested that the clearance of capillary staining of C3d occurs within 2 weeks to 1 month and C4d within 1–2 months, although anecdotally, staining can persist for several months, although the clinical significance is not clear. Although it is recommended that AMR surveillance using immunofluorescence or histological techniques be performed, it has been acknowledged that this may represent a challenge for some centers. For those centers, it was recommended that immunostaining be guided by histological features. To be considered positive, staining for C4d and C3d may be either weak or strong in intensity but must be multifocal or diffuse in distribution. The clinical significance of lesser degrees of staining is unclear and consideration should be given to monitoring.
Histological features should also be considered. Morphological findings suggestive of AMR include the presence of intravascular activated macrophages or endothelial cells, hemorrhage, interstitial edema, myocyte necrosis, capillary damage, and evidence of inflammation [46].
Supporting features of AMR include donor specific antibodies and clinical presentation. The topic of DSAs exceeds the scope of this chapter. Currently, there is no consensus on what constitutes significant levels of DSA and how they should be managed in the absence of clinical or pathological findings. It is recommended, however, that they are monitored routinely after transplant or when rejection is suspected (◘ Table 32.5) and that solid phase or cell-based assays be used. Clinical features may include signs and symptoms of heart failure, restrictive physiology, hemodynamic and/or echocardiographic parameters, and requirement for circulatory support. It is not yet clear whether the diagnosis of pAMR alone, without clinical or supporting features is enough to warrant treatment except in the case of severe pAMR or pAMR 3. If there is clinical evidence of AMR, then treatment should be initiated regardless of the level of pAMR (◘ Tables 32.6 and 32.7). There is less clarity with intermediate degrees of pAMR and an absence of clinical findings. The decision to treat the patient should be considered carefully with collaboration between the pathologist, immunologist, and cardiologist.
Interpretation | AMR | Limitations | |
IgG/IgM | Immunoglobulin binding | + | Easily dissociated |
Short half-life | |||
Interobserver variability | |||
C3, C1q | Complement activation | + | Short half-life |
C3d/C4d | Complement activation | + | Combination more predictive of AMR than C4d alone, long half-life |
HLA-DR | Endothelial integrity | + | Staining always present, but “frayed” pattern indicates capillary injury |
Fibrin | Thrombotic environment | + | Interstitial extravasation suggests more severe AMR episode |
CD55, CD59 | Complement inhibitor | − | Long incubation and granular staining pattern |
Difficult to interpret | |||
CD31, CD34, CD68 | Intravascular macrophages | + | CD68 confirms macrophage lineage of mononuclear cells |
CD31/34 are endothelial markers which differentiate macrophages from endothelial cells and delineates intravascular location |
Theraputic modality | Mechanism of action | Adverse events |
---|---|---|
Corticosteroids Mg | Upregulation of anti-inflammatory gene expression, mediated by activated protein-1 and NF-kB, Blockade of Fc-q receptor, Complement inhibition, Downregulates B-cell receptor, Neutralizes circulating antibody and cytokines | Dyslipidemia, hyperglycemia, osteoporosis, leukocytosis |
Headache, Chills, Rigors, Fever, Myalgia, Volume overload | ||
Tissue plasma exchange (plasmapheresis) | Nonselective removal of circulating alloantibody, proteins, cytokines; IAP removes only immunoglobulins | Rebound antibodies, Bleeding diathesis, Hypotension, Allergic reaction, Transmission of blood-borne pathogens |
Photophoresis | Upregulation of costimulatory molecules, downregulation of T cells, immunoregulation via T-regulatory cells | Vascular access complications, skin erythema, pruritus, nausea, rare drug-induced lupus or scleroderma-like syndrome |
Monomurab (OKT3) | Binds CD3 antigen on T lymphocytes, leading to early activation of T cells, cytokine release, and blockade of T-cell function | Cytokine release syndrome, anti-murine antibodies, anaphylaxis, hypersensitivity, infection (viral, fungal, bacterial), increased incidence of PTLD and lymphoma |
Rabbit ATG (thymoglobulin) | Decrease circulating T lymphocytes | GI (diarrhea, abdominal pain, nausea, vomiting) myalgias, headache, dizziness, dyspnea, hypertension peripheral edema, tachyarrhythmia, hypokalemia, leukopenia, thrombocytopenia, fever, infection (viral, fungal, bacterial), increased incidence of PTLD and lymphoma |
Equine antithymocyte globulin (ATGAM) | Binds CD3 antigen on T lymphocytes | GI (diarrhea, abdominal pain, nausea vomiting) myalgias, headache, dizziness, dyspnea, hypertension peripheral edema, tachyarrhythmia, hypokalemia, leukopenia, thrombocytopenia, fever, infection (viral, fungal, bacterial), increased incidence of PTLD and lymphoma |
Rituximab | B lymphocyte depletion, antibody depletion, complement-induced cell lysis, Induction of apoptosis | Fever, chills, nausea, headache, myalgia, rash |
Alemtuzumab | Monoclonal antibody against CD52 on surface of all B and T lymphocytes, absent on platelets, hematopoietic stem cells, and lymphoid progenitors; transient depletion of mature lymphocytes without myeloablation | Lymphopenia, pancytopenia, infusion-related effectsa; increased CMV viremia, coagulopathy, cardiac toxicity (heart failure, arrhythmias) in patients receiving chemotherapy |
Bortezomib | Reversible 26S proteasome inhibitor present on plasma cells | Diarrhea, sensory neuropathy, fatigue, thrombocytopenia, conjunctivitis |
Eculizumab | Terminal complement (C5) inhibitor | Flu-like symptoms, sore throat, headache, pack pain, nausea, neutropenia, extravascular hemolysis, increased risk of meningococcal infection |
Mycophenolate | Reversible inosine monophosphatase dehydrogenase blocker that inhibits de novo guanosine synthesis, inhibits T- and B-cell proliferation | Diarrhea, esophagitis, increased lymphomas and other malignancies, leukopenia, anemia, thrombocytopenia, increased CMV infection, hypogammaglobulinemia |
Cyclophosphamide | Nitrogen mustard alkylating antineoplastic agent, targets B cells, inhibition of cholinesterase activity | Bone marrow toxicity, hemorrhagic cystitis, gonadal failure, malignancies, nausea, diarrhea, vomiting, stomatitis, mucositis, anorexia, pacytopenia, cardiotoxicity, interstitial pneumonitis, pulmonary fibrosis, hepatoxicity, toxic epidermal necrolysis, teratogenic |
Total lymphoid irradiation | Suppression of activated T cells and interleukin-2 pathway, eliminates circulating T and B cells | Bone marrow suppression, pancytopenia, nausea, PTLD, myelodysplasia, opportunistic infection, PTLD |
Splenectomy | Diminishes antibody production by debulking plasma cells activated B cells | Increased risk of sepsis and/or death (kidney transplant) |
Immunopathologic indicators | Required | Supporting features | Recommended clinical indicators |
Histology | Capillary endothelial changes and intracapillary macrophages | – | Clinical heart failure based on symptoms and signs of heart failure Hemodynamics: PCWP >20 mmHg and CI < 2.0 L min−1 m−2 |
Frozen section: immunofluorescence | C4d, C3d (2–3+ intensity) anti-HLA-DR | C1q (2–3+), Ig, fibrin, IgG, and IgM HLA for cellular integrity | Requirement for inotropes or mechanical support during hospital stay |
Paraffin section: Immunohistochemistry | C4d (2–3+ intensity), CD68 | Pan-T-cell Cd3, pan-B-cell CD20, complement C3d, endothelial cell CD31 or CD34, complement regulatory proteins | Systolic dysfunction: EF < 50 % or ≥25 % decrease from baseline |
Other | – | Presence of circulating donor-specific HLA antibodies, especially those that fix complement | Restrictive physiology characterized by the following: EF > 50 %, E/A > 2 IVRT < 60 ms, and DT < 150 ms and/or RAP > 12, PCWP > 25 mmHg, and CI < 2.0 L min−1 m−2 |
Management of AMR
As the number of cardiac transplants is limited, there are no large studies that examine treatments for AMR. ISHLT treatment guidelines have been established, but all recommendations are based on consensus (level of evidence C) [47]. Due to the limited number of heart transplants each year, transplant management typically follows the treatment protocols for the kidney transplant program (◘ Fig. 32.3). Therapies for AMR have usually been based on agents that were originally used to treat cancer, blood diseases, and autoimmune diseases [34]. The goals for managing or treating AMR include the following: (1) suppression of the T-cell mediated response (corticosteroids, mycophenolate mofetil (MMF), anti-lymphocyte antibodies, photopheresis, etc.), (2) elimination of circulating antibodies (e.g., plasmapheresis), (3) inhibition of remaining antibodies (e.g., intravenous immunoglobulins), (4) suppression or depletion of B cells (e.g., corticosteroids, rituximab, or splenectomy), (5) suppression or depletion of plasma cells (e.g., bortezomib), and (6) inhibition of complement (e.g., eculizumab, intravenous gamma globulin [IVIg]). Additional information for using these therapies for AMR is outlined in ◘ Table 32.6.
Corticosteroids
Corticosteroids were first used in clinical renal transplantation in 1963 and ultimately have become a standard immunotherapy for induction, maintenance, and antirejection in heart transplantation [48, 49]. Both corticosteroid pulse and taper regimens have been considered important components of standard therapy for acute cellular rejection for decades and have been adapted as baseline therapy for AMR [47]. Corticosteroids are potent specific and nonspecific immunosuppressive and anti-inflammatory agents with a plethora of physiologic and cellular effects by impacting the number and function of leukocytes and endothelial cells [50]. A single dose of corticosteroids has been shown to cause a transient 90 % decrease in circulating monocytes and a 70 % decrease in circulating lymphocytes ([51]). This effect appears to occur within 4–6 h and normalizes within 24 h. T cells appear to be more sensitive to suppression than B cells. The effects on immunoglobulin production appear to be variable, with low doses of corticosteroids having little to no effect, while high dose appears to suppress immunoglobulin production 2–4 weeks after treatment. The transcription factors nuclear factor-kB and activator protein-1 are the downstream effectors that mediate the cellular response to corticosteroids [52, 53]. Corticosteroids also exert their effect via suppression of IL-1 and decrease in Fc and C3 receptor expression and function [51]. The lone impact of corticosteroids for the treatment of AMR is difficult to define as essentially every treatment protocol includes corticosteroids in combination with other immunotherapies. Nevertheless, corticosteroids are a commonly used component for the treatment of AMR .
Intravenous Gamma Globulin
Intravenous gamma globulin or IVIg is pooled IgG antibodies obtained from plasma of numerous donors. IVIg contains anti-idiotypic antibodies that inhibit Class I and II HLA-specific alloantibodies, constimulatory molecules, complement, cytokine and cytokine receptors, and T-cell and B-cell receptors in vitro and in vivo [54–57].
IVIg has been used to treat the highly sensitized patient prior to cardiac transplantation but its efficacy for prevention of AMR is unknown [58]. IVIg is frequently used in combination with other immunomodulating therapies for the treatment of desensitization or AMR so the functional impact of IVIg alone is unclear. In limited studies of kidney and heart transplant recipients who developed AMR, IVIg administered with cyclophosphamide or tacrolimus was shown to successfully reverse rejection but had a high rate of recurrence [3, 59]. IVIg is less expensive and probably has a relatively more favorable side effect profile when compared to other immunomodulating agents .
Plasmapheresis
Using therapeutic plasma exchange, double-filtration plasmapheresis , and immunoadsorption pheresis, plasmapheresis removes circulating antibodies. Plasma exchange uses membrane filtration or centrifugation to separate plasma from the cellular components of blood. Exogenous albumin and/or fresh-frozen plasma or crystalloid are added back to the cellular components of the patient’s blood and reinfused to the patient. Consequently, plasmapheresis nonselectively removes all proteins. In contrast, immunoadsorption plasmapheresis removes only immunoglobulins and less efficiently removes soluble cytokines. However, immunoadsorption plasmapheresis does not require the replacement of fluids to the patient.
Plasma exchange is more widely used in the USA due to its relatively lower cost, usability, and availability [57]. The most common method used for the decrease of soluble alloantibody levels in transplantation patients with plasmapheresis is the use of plasma exchange [58]. Although plasma exchange is commonly used for the treatment and management of AMR, there have been no randomized trials using plasma exchange in cardiac transplant recipients [57]. There have been a number of case series reports that used plasmapheresis for the initial treatment and refractory AMR in cardiac transplantation in addition to several case reports describing the use of plasma exchange for the reduction of alloantibodies in highly sensitized patients awaiting heart transplant and in facilitating transplant across a positive crossmatch [60–62].
There are limited data to support the use of plasma exchange as monotherapy as its reported use is typically in combination with other therapies. Wang et al. [62] reported the use of plasma exchange in patients with cardiac allograft dysfunction, hemodynamic compromise, and biopsy-proven AMR. These cardiac transplant recipients with AMR were treated using a protocol that included 5 days of plasma exchange and 3 days of intravenous corticosteroids (methylprednisolone 1 g/day). In a number of cases, tacrolimus was substituted for cyclosporine. Although the overall survival was decreased in the patients with AMR, this treatment protocol resulted in 1- and 5-year survival rates of 75 % and 51 %, respectively.
In a similar retrospective study, 11 of 12 patients with hemodynamic compromise and biopsy proven AMR, had recovery of allograft function after treatment for 3 days with methylprednisolone 1 g/day and a 1 week protocol of daily plasma exchange [2]. In this series, baseline immunosuppression was modified with the substitution of MMF or cyclophosphamide for azathioprine and tacrolimus for cyclosporine.
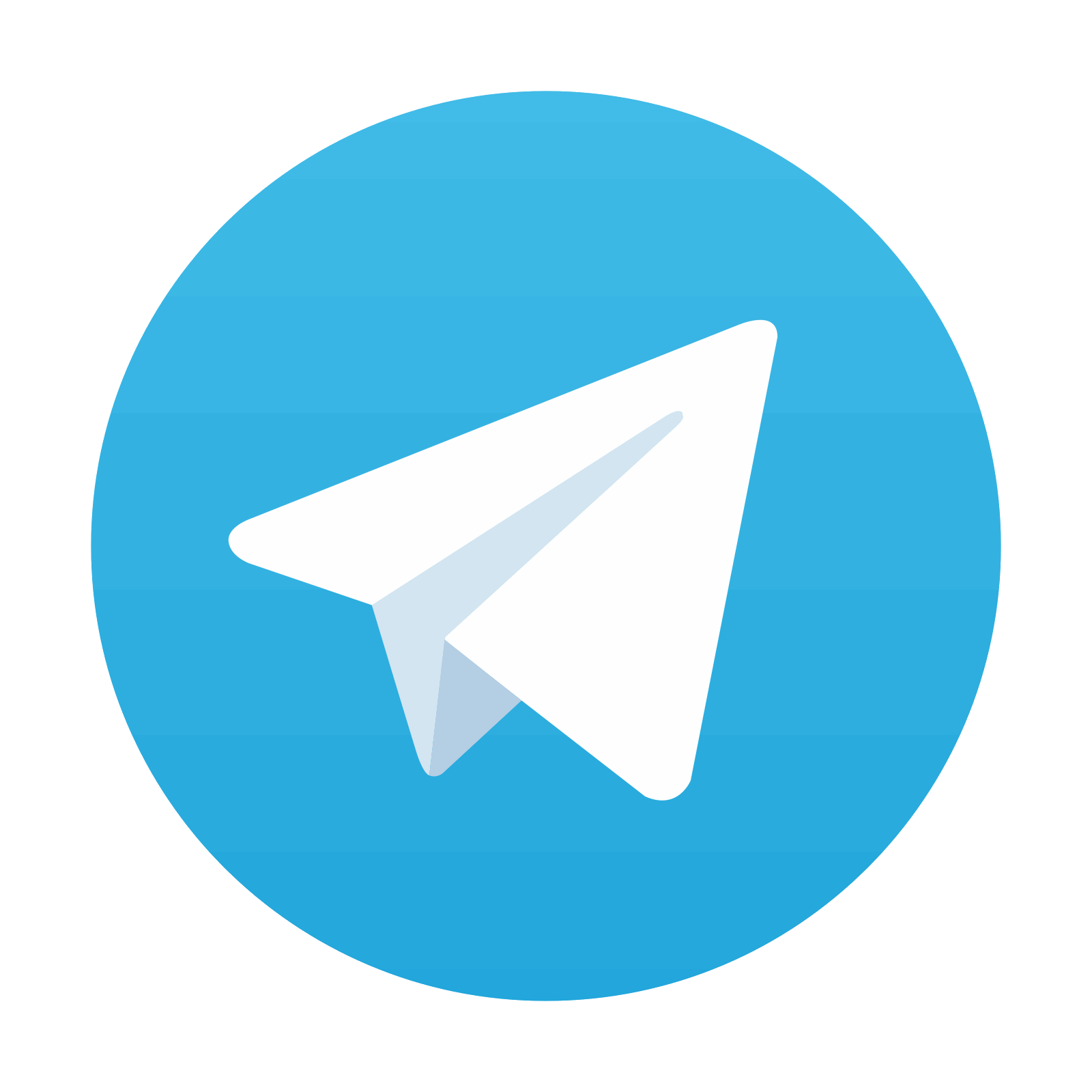
Stay updated, free articles. Join our Telegram channel

Full access? Get Clinical Tree
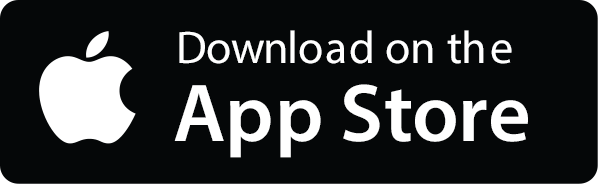
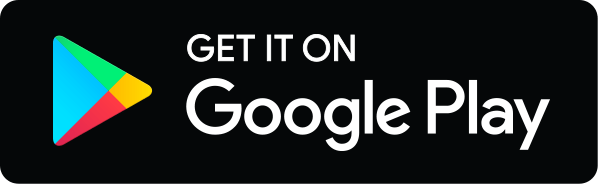