Fig. 18.1
The basic mechanism of negative remodeling and progression to dilated cardiomyopathy in hypertension
Therefore, it is plausible to hypothesize that hypertensive cardiomyopathy is a preventable disease, at least in its early stages. In patients with left ventricular remodeling, controlling blood pressure could halt or reverse this cardiomyopathic process. In patients with established heart failure, blood pressure control reduced the number of rehospitalizations, slowed the progression of disease, and improved overall mortality. In large-scale randomized prevention trials, antihypertensive therapy produced as much as a 50 % relative risk reduction in the incidence of heart failure [19–21]. Consistent with the Eighth Joint National Committee (JNC 8) and the European societies of hypertension and cardiology, antihypertensive medications should generally be initiated if the office systolic blood pressure is persistently ≥140 mmHg or the diastolic pressure is persistently ≥90 mmHg [8]. In 2015, results of the SPRINT trial (Systolic Blood Pressure Intervention Trial) showed that blood pressure control at 120/80 mmHg was associated with further significant cardiovascular risk reduction compared to blood pressure control at 140/90 mmHg [22].
Interestingly, multiple large-scale studies concluded that the degree of blood pressure reduction, not the choice of antihypertensive medication, is the major determinant of reduced cardiovascular risk in patients with hypertension. For example, the recent meta-analysis of 35 randomized controlled trials highlighted that antihypertensive medications effectively prevented new-onset heart failure, with a relative risk reduction of 37 %. It is further suggested that lowering blood pressure using calcium channel blockers is as effective in preventing “new-onset” heart failure as other antihypertensives [23]. Nonetheless, certain classes of antihypertensive medications are indicated in patients with heart failure. These are primarily angiotensin-converting enzyme inhibitors (ACEIs) , angiotensin receptor blockers (ARB), a combination of nitrates and hydralazine, and beta-blockers (BBs), given their proven mortality benefit in this population [24]. Aldosterone antagonists (especially spironolactone) are the cornerstone in resistant hypertension [25], and their administration has resulted in significant reductions in morbidity and mortality in HFpEF [26] and HFrEF [27].
Diabetes Mellitus and Heart Failure Prevention
Diabetes mellitus represents a significant health-care burden, affecting an estimated 450 million people across the globe in 2013 [28]. This endocrinological imbalance is associated with a myriad of cardiovascular conditions, including heart failure, with considerable morbidity and mortality [29]. As reported in the Framingham studies, diabetes mellitus is associated with five times increased risk in women and two times increased risk in men of attaining clinically significant heart failure [30]. Moreover, patients with diabetes mellitus aged 45–54 years are almost nine times more likely to develop heart failure [31]. The prevalence of heart failure in diabetic patients is greater than that of the general population [31, 32]; as such, every 1 % increase in glycosylated hemoglobin is linked to an 8 % increased risk of heart failure [33]. This is important, as the diagnosis of diabetes mellitus in heart failure patients is associated with a worse prognosis compared to heart failure patients without diabetes [34].
Although atherosclerotic coronary artery disease is the major cause of heart failure in diabetic patients, the pathogenesis of heart failure in this population remains multifactorial and includes diabetic cardiomyopathy, hypertensive cardiomyopathy, and atherosclerosis [35]. The entity of diabetic cardiomyopathy, first described by Rubler in 1972, represents the direct impact of insulin resistance and hyperglycemia on the myocardium, irrespective of the presence of significant atherosclerosis of epicardial vessels [36].
Disease progression in diabetic patients is unclear, but evidence suggests it starts with the formation of diabetic cardiomyopathy without any systolic or diastolic dysfunction. Both the Framingham study and the Strong Heart Study showed early evidence of left ventricular hypertrophy development associated with diabetes [37, 38]. Hypertrophy of cardiomyocytes is initially related to increased insulin levels and, subsequently, to insulin resistance [39]. One consequence of insulin resistance is an enhanced release of free fatty acids with a resultant reduction in myocardial glucose transporter expression and glucose uptake. This causes the activation of myocardial peroxisome proliferator-activated receptor-α that stimulates the transcription of multiple genes responsible for the increase in mitochondrial transport and oxidation [40]. In response to myocardial stress, fatty acids accumulate within the cardiomyocytes, and toxic lipid intermediates such as diacylglycerol and ceramides and myocytic apoptosis are produced.
Diabetes is also associated with abnormalities related to cardiomyocyte contractile protein expression and calcium (Ca) sensitivity. These abnormalities include a shift in myosin isoenzyme composition and the predominance of the fetal β myosin heavy chain expression with respect to α-myosin heavy chain [41]. Both lead to depressed ATPase activity of myofibrils and reduced contractile force. In addition, oxidative stress in cardiomyocytes incubated in a high-glucose medium has been shown to inactivate sarcoplasmic reticulum Ca-ATPase (SERCA) 2 activity. This hyperglycemic state results in inefficient sequestration of Ca in the sarcoplasmic reticulum, Ca overload in the cytosol, and impaired relaxation [42]. Ultimately, the formation of advanced glycation end products, lipid oxidation, impaired nitric oxide production, endothelial dysfunction, and microvascular remodeling will cause the early diastolic dysfunction evident in patients with diabetes.
Using tissue Doppler imaging, left ventricular diastolic dysfunction was observed in upwards of 75 % of asymptomatic diabetic patients [43]. As ventricular diastolic dysfunction progresses, activation of the sympathetic nervous system and the renin-angiotensin-aldosterone system would favor myocardial fibrosis, apoptosis, vascular inflammation, and oxidative damage, leading to systolic dysfunction and dilated cardiomyopathy [44]. Individuals with diabetes mellitus characteristically develop atherosclerotic coronary artery disease at an early age that is also more likely to include the microvascular bed [45]. They also have decreased angiogenesis, leading to reduced collateral vessel formation, with increased risk of heart failure [46]. Finally, comorbidities that commonly accompany diabetes, such as hypertension, dyslipidemia , microvascular dysfunction, and renal impairment, may accelerate the progression of cardiac dysfunction toward end-stage heart failure [47] (◘ Fig. 18.2).


Fig. 18.2
A schematic of the molecular pathways involved in the development of diabetic cardiomyopathy in diabetes mellitus. LV left ventricle, LVH left ventricular hypertrophy
From the earliest stages, a treatment strategy that decreased insulin resistance and hyperglycemia would prevent the progression of the cardiomyopathy [48]. Once there is evidence of cardiomyopathy, standard heart failure therapies should be implemented. However, it is unclear whether the mortality of diabetics with heart failure remains higher than their nondiabetic counterparts—in spite of the advanced therapies used. Ongoing discussion has addressed the intrinsic effects of oral antidiabetic drugs and protection from cardiovascular disease, including heart failure. The potential underlying mechanisms are speculative and complex. In 2015, the cardiovascular safety study of empagliflozin use in diabetes mellitus (EMPA-REG OUTCOME trial ) showed that empagliflozin not only reduced the cardiovascular death rate (a 38 % relative risk reduction), but it also significantly reduced the risk of hospitalization for heart failure (a 32 % relative risk reduction) [49].
Heart Failure Prevention and Dyslipidemia
Dyslipidemia is a well-studied risk factor for coronary atherosclerotic disease, and it contributes to the incidence of ischemic cardiomyopathy and heart failure [50] and diabetes mellitus [51]. However, the direct effect of dyslipidemia on the myocardium remains controversial. Cardiomyocytes have limited lipid storage capacity, and excess fatty acids are shunted into non-oxidative pathways that generate toxic by-products leading to contractile dysfunction [52, 53]. Evidence has implicated high levels of free fatty acids and triglycerides in cardiotoxicity, and elevated levels of lipid fractions may be involved in cardiac remodeling and heart failure [54]. However, reports from observational studies on the associations of triglycerides and total cholesterol (TC)/high-density lipoprotein (HDL) cholesterol ratio with incident heart failure have been inconsistent [51]. In the Multiethnic Study of Atherosclerosis (MESA) study, neither high triglyceride nor low HDL was a significant predictor of future occurrence of heart failure [55]. In a later report from the same study, lipid measurements were not associated with the occurrence of heart failure in individuals without diabetes mellitus. However, in diabetics, high triglyceride, low HDL, or high total cholesterol/HDL-C ratio were significantly associated with heart failure [51]. In other studies, low HDL but not high triglyceride was an independent predictor of heart failure in all patients [56, 57]. In the Physicians’ Health Study, neither HDL nor TC/HDL ratio was independently associated with heart failure [54]. In the Framingham Heart Study, elevated levels of non-HDL, decreased levels of HDL, and a high TC/HDL ratio were associated with increased risks of heart failure [58, 59].
The effects of HDL on heart failure are less clear. Small- plus medium-diameter HDL particles are an independent predictor for chronic inflammation-related death and hospitalization and for coronary heart disease events in subjects initially free of overt cardiovascular disease. These findings support the hypothesis that smaller HDL particles having a diameter <9.4 nm have anti-inflammatory properties in the myocardium [60]. Due to these inconsistencies, a paucity of data governs the use of lipid-lowering agents in the primary or secondary prevention of heart failure.
The Controlled Rosuvastatin Multinational Trial in Heart Failure (CORONA) [61] and the GISSI-HF [62] randomized controlled trials were specifically designed to investigate the effect of statin (rosuvastatin) on the prognosis of patients with heart failure. In the CORONA study, patients were randomized to receive either rosuvastatin (10 mg) or placebo for a median follow-up of about 3 years. Despite a 45 % reduction of LDL, the primary cardiovascular end point of mortality was not significantly different compared to placebo. However, hospitalization due to heart failure was significantly reduced by rosuvastatin administration. Prognosis was improved among patients with plasma N-terminal B-type natriuretic peptide (NT-proBNP) levels in the lowest tertile, suggesting a benefit for patients with less-severe heart failure [61]. In the GISSI-HF randomized, double-blind trial, patients were randomized to rosuvastatin (10 mg) or placebo. Surprisingly, rosuvastatin failed to improve mortality despite a substantial reduction of LDL [62]. Subsequently, in a recently published meta-analysis of studies in primary and secondary prevention trials, statins modestly reduced the risks of nonfatal heart failure hospitalization and a composite of nonfatal heart failure hospitalization and death [63]. Nonetheless, as coronary artery disease is the determinant risk factor of heart failure, and often is caused by dyslipidemia, its treatment would ultimately reduce the incidence of heart failure (◘ Fig. 18.3).


Fig. 18.3
Cholesterol metabolism pathway. Endogenous production of cholesterol begins via the mevalonate pathway where two molecules of acetyl coenzyme A (CoA) react to form acetoacetyl-CoA. This is followed by a second reaction between acetyl CoA and acetoacetyl-CoA to form 3-hydroxy-3-methylglutaryl CoA (HMG-CoA). This molecule is then reduced to mevalonate by the enzyme HMG-CoA reductase, which is the rate-limiting step of cholesterol synthesis—site of action of statins. Cholesterol is amphipathic, thus it is transported inside lipoprotein complexes in the bloodstream. There are several types of lipoproteins, including chylomicrons, very-low-density lipoprotein (VLDL), low-density lipoprotein (LDL), intermediate-density lipoprotein (IDL), and high-density lipoprotein (HDL). LDL particles are the major blood cholesterol carriers. Each one contains approximately 1500 molecules of cholesterol ester. LDL molecule shells contain just one molecule of apolipoprotein B100, recognized by LDL receptors in the peripheral tissues. Via binding of apolipoprotein B100, LDL receptors-LDL complexes concentrate in clathrin-coated vesicles that get internalized via endocytosis and directed for metabolism. Proprotein convertase subtilisin/kexin type 9 (PCSK9) is an enzyme that binds to the LDL receptor. In the liver, the LDL receptor scavenges the LDL molecules from the bloodstream. When PCSK9 binds to the LDL receptor, the receptor gets inhibited. If PCSK9 is blocked (site of action of PCSK9 inhibitors), more LDL receptors will be present on the surface of the liver and will remove more LDL cholesterol from the bloodstream, thus lowering LDL serum levels
Prior large prospective clinical trials involving statins have provided clear evidence of the effect of LDL reduction on cardiovascular events. However, there remains a population of patients who need dyslipidemia therapy but have contraindications to or are intolerant of statins. Several agents are being developed with higher efficacy than statins. Proprotein convertase subtilisin/kexin type 9 (PCSK9) inhibitors were developed to significantly impact on the LDL levels. The PCSK9 protein produced by the hepatocyte binds to the LDL-LDL receptor (LDLR) complex on the surface of the hepatocytes, resulting in its internalization. LDL binding to LDLR on the hepatocyte is the mechanism for eliminating LDL and other non-HDL molecules. However, when PCSK9 is bound to the LDL-LDLR complex, it leads to lysosomal catabolism of LDLR within the hepatocyte and prevents LDLR recycling [64]. By sequestering PCSK9, the PCSK9 inhibitors block the binding of PCSK9 protein to the LDLR. This prevents LDLR catabolism which, in turn, preserves LDLR recycling and increased receptor density on the hepatocyte surface. Increased LDLR density increases LDL binding and the clearance from the blood, resulting in reduced LDL serum levels.
In 2009, two PCSK9 inhibitors in the form of monoclonal antibodies alirocumab and evolocumab were used in clinical trials in humans, resulting in Food and Drug Administration approval in 2015. In the first published phase I trials on the use of PCSK9 inhibitor, alirocumab significantly reduced LDL cholesterol levels (as high as 60 %) in healthy volunteers and in subjects with familial or nonfamilial hypercholesterolemia [65]. Although the efficacy and safety of alirocumab and evolocumab have been demonstrated in multiple trials, clinical trials addressing their effects on cardiovascular outcomes, including heart failure, are underway [66].
Social Behavior and Prevention: Polysubstance Abuse
Cocaine–induced cardiomyopathy : Cocaine, benzoylmethylecgonine, is a potent neurostimulant used illicitly for recreation [67]. It is commonly nasally snorted or smoked. According to the World Health Organization (WHO), nearly 6.9 million individuals were estimated to have cocaine dependence in 2001, with 25,000 years of life lost due to dependency [68]. It is associated with multiple cardiovascular complications, including myocardial ischemia and infarction, cardiomyopathy, arrhythmias, stroke, and hypertension [69]. Cocaine-induced dilated cardiomyopathy remains a rare cause of heart failure [70]. The mechanisms underlying cocaine cardiomyopathy are not fully understood but are hypothesized to include sympathomimetic activation, increased calcium influx and oxidative stress, and myocardial ischemia [71].
Strong clinical suspicion should be considered in young men without traditional cardiovascular risk factors who are presenting with heart failure. While the management of cocaine cardiomyopathy is similar to other forms of dilated cardiomyopathy, BBs should be avoided. Left ventricular function will likely improve dramatically with abstinence [72]. Unfortunately, addiction and relapse are high, and left ventricular dysfunction often persists, resulting in high mortality. Additional epidemiological studies are needed to fully assess its impact on health care (◘ Fig. 18.4).


Fig. 18.4
Cocaine has an adverse impact on the heart. (a) The molecular conformation of cocaine. (b) The side effects of cocaine abuse
Androgenic steroid–induced cardiomyopathy : Anabolic steroids, also known as anabolic-androgenic steroids, are hormones with cyclic ring conformation with similar effects to endogenous androgenic steroids such as testosterone. Thus, they have androgenic properties, including the development of masculine male characteristics. They also increase protein production and accumulation, especially in skeletal muscles. Anabolic steroids were initially developed in the early nineteenth century as therapeutic options for patients with puberty disorders and cachexia [73]. However, it was not until the 1980s that the American College of Sports Medicine acknowledged their use by athletes to increase muscular mass and physical endurance [74].
Unfortunately, anabolic steroid abuse among athletes has dramatically increased—with the use of much higher doses for improved muscular appearance. One survey found that two-thirds of professional body builders have reported use of anabolic steroids to enhance performance [75]. These steroids cause several deleterious effects on the cardiovascular system, including cardiomyopathy, atherosclerosis, increased vascular tone, dyslipidemia, and sudden cardiac death [76]. Via their interaction with androgenic receptors in a dose-dependent response, anabolic steroids induce left ventricular hypertrophy, which progresses to dilated cardiomyopathy [77, 78].
Several studies showed that high doses of anabolic steroids such as nandrolone may lead to a phenotype similar to hypertrophic cardiomyopathy, followed by apoptosis which is mediated by intracellular Ca influx and mobilization [79, 80]. This ultimately leads to ventricular remodeling, failure, and sudden cardiac death. In animal models, although a short course (<2 weeks) of nandrolone improved postischemic myocardial performance in postconditioned myocardium, the prolonged administration (>10 weeks) increased myocardial susceptibility to ischemia-reperfusion injury and abolished cardioprotection. This increased susceptibility might be related to steroid-induced hypertrophy or increases in the preischemic myocardial cAMP concentrations [81]. In addition, anabolic steroids have previously been linked to Takotsubo “stress” cardiomyopathy [82]. Finally, anabolic steroids induce a decrease in HDL by 20 % and an increase in LDL by 20 % due to lipolytic degradation of lipoproteins and their removal by receptors through modification of apolipoprotein A-I and B synthesis [83]. Thus, use of these steroids increases the risk for coronary artery disease and myocardial ischemia. Whether the abstinence of steroids would result in reverse remodeling of the myocardium is still unclear (◘ Fig. 18.5).


Fig. 18.5
Androgenic steroids have an adverse impact on the heart. (a) The molecular conformations of the more commonly used androgenic steroids, compared to testosterone. (b) The side effects of androgen abuse
Amphetamine–induced cardiomyopathy : Amphetamines, belonging to the phenethylamine class, are potent central nervous system stimulants used primarily for treating attention deficit hyperactivity disorder [84]. However, amphetamines are being used recreationally as an illicit drug [85]. The chronic use of amphetamines has been associated with the development of cardiomyopathy and systolic heart failure [86, 87]. The mechanism of the dilated cardiomyopathy is unclear but is likely driven by the adrenergic signaling pathway [72]. Amphetamines have a dopaminergic substrate with sympathomimetic qualities. They are also potent reversible inhibitors of monoamine oxidase-A, the neuronal enzyme that inactivates norepinephrine and dopamine [88]. In addition to causing hypertensive emergency or tachycardia, direct myocardial toxicity can lead to left ventricular failure, recurrent coronary vasospasm, and accelerated atherosclerosis [89, 90].
Autopsy studies of methamphetamine users have shown myocardial necrosis [91]. 3,4-Methylenedioxymethamphetamine, known as ecstasy, has been shown to cause myocarditis with inflammatory cellular infiltrates and necrosis [92]. Eventually, this drug causes eccentric left ventricular dilation and diastolic dysfunction, leading to systolic dysfunction. Although evidence has shown that the myocardial pathology may be reversible with early cessation of exposure to amphetamine [93], complete abstinence and standard heart failure therapy should be implemented.
Alcohol Abuse and Heart Failure Prevention
Excessive alcohol intake is the third leading cause of premature death among males aged 15–59 years in the United States [94]. Alcohol abuse, defined as three or more standard drinks per day, has been associated with cardiovascular disease. Cardiovascular complications include alcoholic cardiomyopathy, arrhythmias, and hypertension [95]. By comparison, daily consumption of low to moderate amounts of alcohol has beneficial effects on cardiovascular health and is associated with reduced all-cause mortality [96].
Alcoholic cardiomyopathy is an acquired dilated cardiomyopathy associated with long-term heavy alcohol consumption (>80 g of ethanol per day for at least 5 years) [97, 98]. Epidemiological studies that investigated the prevalence of alcohol abuse among heart failure patients have found high alcohol consumption in 3.8–47 % of dilated cardiomyopathy cases [99]. The relationship between alcohol intake and survival is described as a J-shape. More than moderate drinking—defined as up to one drink per day for females and up to two drinks per day for males—induces more harmful effects. Furthermore, low to moderate levels of alcohol intake are associated with improved cardiovascular health [100]. For example, the Atherosclerosis Risk in Communities (ARIC) study reports that males who consume up to seven drinks per week had a 20 % reduced risk of heart failure compared with nondrinkers. This effect was observed less frequently in females [101].
About 50 % of asymptomatic alcoholic subjects have a mild increase in left ventricular wall thickness without echocardiographic evidence of decreased myocardial contractility [102]. From another perspective, alcohol intoxication can produce acute asymptomatic left ventricular dysfunction even when ingested by healthy individuals in quantities typical of social drinking [103]. Binge drinking is associated with transient myocardial changes that are detectable by cardiac magnetic resonance imaging, elevated serum markers for myocardial injury such as troponins, and coronary arterial vasospasm [104]. However, the development of alcoholic cardiomyopathy is not only related to the mean daily alcohol intake but also to the duration of use [105]. Alcoholic cardiomyopathy is characterized by increased ventricular mass, diastolic dysfunction, left ventricular dilatation, and, eventually, systolic failure. Although cardiac magnetic resonance imaging is extremely useful in analyzing the cardiomyopathic heart, alcoholic cardiomyopathy does not have specific structural features compared to idiopathic dilated cardiomyopathy [106].
The early stages of alcoholic cardiomyopathy are characterized by spatial disorganization of the mitochondrial ultrastructure followed by irreversible mitochondrial damage and lipid accumulation [107]. Additional mechanisms of myocardial injury include apoptosis [108], excitation-contraction coupling dysfunction [109], decreases in contractile proteins and myofibrils [110], upregulation of the L-type calcium channels and calcium inflow [111], and activation of the renin-angiotensin system and the sympathetic nervous system [112]. Histologically, myocardial fibrosis, focal myocardial edema, endocardial fibroelastosis, and vascular thrombosis are abundant [113]. However, earlier mitochondrial changes seem more specific to alcohol ingestion [114].
Treatment of alcoholic cardiomyopathy is total and permanent abstinence from alcohol consumption [115]. Some studies have suggested that moderation of alcohol consumption may produce similar outcomes as abstinence. Though some studies have cited the lack of myocardial interstitial fibrosis as a potential indicator of reversibility, the limited data on morphometry have yielded mixed results [116, 117]. Small observational studies have suggested that left ventricular function improves in some patients with abstinence, as early as 6 months. A shorter duration of heart failure symptoms prior to abstinence may favor recovery [118]. These notions were illustrated in a prospective study of 55 men with a cardiomyopathy who consumed ≥100 g alcohol per day for at least 10 years [119]. During a 1-year follow-up, the improvement in systolic function was the same in abstainers and those who controlled their intake. In contrast, patients who continued to drink >80 g per day had a further deterioration in the ejection fraction.
In patients with asymptomatic left ventricular dysfunction, BBs and ACEIs/ARBs coupled with abstinence may halt or reverse the negative remodeling of the myocardium. Patients should also eat a balanced diet and correct any nutritional deficiencies.
The prognosis of alcoholic cardiomyopathy varies depending on the presence and extent of continued alcohol use. Patients who abstain from alcohol or continue moderate alcohol use have a prognosis better than or similar to that seen with idiopathic dilated cardiomyopathy, while continued heavy alcohol use is associated with a worse prognosis. In a study by Gavazzi et al., non-abstainers had a lower 7-year, transplant-free survival rate of 27 % compared with those who stopped drinking (45 %) or those with an idiopathic cardiomyopathy (53 %) [120]. Among patients with alcoholic cardiomyopathy, independent predictors of death include heart transplantation, atrial fibrillation, QRS duration >120 ms, and a lack of BB therapy [121] (◘ Fig. 18.6).


Fig. 18.6
Alcohol-induced cardiomyopathy . The side effects of chronic ingestion of excessive amounts of ethanol
Human Immunodeficiency Virus and Heart Failure Prevention
Human immunodeficiency virus (HIV ) is a global pandemic affecting more than 40 million individuals worldwide [122]. With the introduction of antiretroviral therapy (ART) , HIV infection has become a chronic disease with improved morbidity and mortality. One result of increased life expectancy, however, is that cardiovascular complications associated with HIV infection are becoming more significant. The constellation of cardiac sequelae includes HIV-associated cardiomyopathy, coronary artery disease, hypertension, and pulmonary hypertension [123].
The advent of ART has significantly changed the incidence and prevalence of cardiac complications—both as a result of HIV infection modulation and medication-related side effects [124]. For example, in the Strategies for Management of Anti-Retroviral Therapy (SMART) study, earlier initiation of ART resulted in decreased inflammatory markers (C-reactive protein, IL-6, and D-dimer), portending less cardiovascular events in the future [125]. Coronary artery disease was the third most common cause of death in HIV-infected patients [126]. Contrarily, the incidence of HIV cardiomyopathy is difficult to assess, because very few studies actually evaluated this outcome. A meta-analysis of 11 studies in the ART era showed a prevalence of systolic dysfunction of 8.3 % and diastolic dysfunction of 43.4 % [127]. The African Heart of Soweto Study investigated the impact of HIV infection on de novo occurrence of heart disease [128]. In that study, 9.7 % of newly diagnosed heart disease patients were identified as HIV positive. Of these patients, 38 % had clinically symptomatic HIV cardiomyopathy. Nonetheless, subclinical myocardial changes are likely underreported. For example, cardiac magnetic resonance imaging detected subtle systolic dysfunction, cardiac lipid deposition, and myocardial fibrosis in almost 100 % of HIV patients on ART therapy [129].
The pathophysiology of HIV cardiomyopathy is multifactorial and complex. It includes direct myocardial infection by HIV-1, toxicity from the ART therapy, coronary artery disease, opportunistic cardiac infections, and nutritional deficiencies [130, 131]. Infection of the myocardium with HIV-1 virus has been postulated as one of the key mechanisms for the development of impaired systolic and diastolic dysfunction [130]. Interestingly, in situ hybridization of HIV-1 using myocardial samples showed that the myocardial interstitial cells, rather than the cardiomyocyte, are primarily infected with the virus [132, 133]. This would ultimately culminate in an autoimmune response to myocardial epitopes and the generation of cardiomyocyte-specific autoantibodies. Furthermore, overexpression of tumor necrosis factor-alpha, reactive oxygen species, and other cytokines ultimately contribute to the more advanced systolic dysfunction [133, 134]. Mitochondrial toxicity, especially by zidovudine, clevudine, and lodenosine, has been shown to cause significant myocardial damage, starting with subtle cardiac abnormalities and leading to more symptomatic cardiomyopathy [135–137]. This is especially significant given the hypothesized synergistic effect with direct HIV myocardial infection. Deficiency of trace elements such as selenium has been associated with cardiomyopathy, as well [138].
Finally, HIV infection represents an independent risk factor for the development of coronary artery disease [139]. Consider that the incidence of acute myocardial infarction occurred at 11.13 per 1000 person-years in HIV patients compared with 6.98 per 1000 person-years in healthy controls [140]. This increased risk was multifactorial and attributed to the HIV virus itself, ART therapy, metabolic derangement, endothelial dysfunction, and high prevalence of recreational drug abuse such as cocaine and tobacco [139]. Not only do these patients have a high incidence of coronary artery disease but their risk of subsequent acute coronary syndrome remains significant. A meta-analysis of 11 studies focusing on acute coronary syndrome in HIV patients found that HIV patients admitted for acute coronary syndrome have a significant short-term mortality of 8 %, a significant long-term risk of myocardial infarction of 9.4 %, and a 20.1 % need for percutaneous coronary revascularization [141]. A landmark study has shown that ART was independently associated with a 26 % relative increase in the rate of acute myocardial infarction per year of exposure during the first 5 years of therapy [142]. However, in the SMART study, treatment interruption was associated with a 57 % increased risk of acute coronary syndrome [143]. More studies are needed to dissect the subpopulations primarily at risk.
Severe systolic dysfunction has a grave prognosis [144]. In a study by Felker et al., the hazard ratio for death was 5.86 compared with patients with idiopathic cardiomyopathy [145]. The impact of diastolic dysfunction on survival is yet to be elucidated. Unfortunately, little is known about the optimal therapy for HIV cardiomyopathy and the response of known heart failure medications in HIV patients. No randomized trials of heart failure medications have been performed at this date. Common recommendations include the use of beta-blockers, reverse remodeling agents, and afterload reduction. Refractory cases could be managed with chronic resynchronization device therapy [146]. However, mechanical circulatory support and even heart transplantation might be needed in end-stage cases [147]. Transplantation outcomes have been favorable, with no increases in acute rejection or worsening of HIV status with immunosuppression [148] (◘ Fig. 18.7).


Fig. 18.7
HIV cardiomyopathy . The retrovirus HIV-1 has a constellation of effects on the cardiovascular system, in addition to direct myocardial damage. The end result is end-stage dilated cardiomyopathy. HIV human immunodeficiency virus
Cancer Therapeutics and Heart Failure Prevention
According to the National Cancer Institute, more than 14 million cancer survivors were estimated to be living in the United States in 2014, having a 60 % 5-year survival rate [149]. Advances in therapeutics for childhood cancers have resulted in even more impressive improvements, with a 5-year survival exceeding 80 % [150]. Nevertheless, long-term side effects of these therapies are surfacing with prolonged survival rates. These side effects include chemotherapy-induced cardiomyopathy and heart failure, which have emerged as primary causes of morbidity and mortality in long-term survivors free of cancer [151]. For example, survivors of childhood cancer have more than 15 times the risk of developing heart failure compared to the general population [152]. Therefore, it is important to understand the pathophysiology of cancer therapeutics-related heart failure in an attempt to mitigate its occurrence and serve as a platform for therapeutic interventions.
Over the past several decades, distinct cardiotoxicity patterns have appeared that relate to specific therapeutics [153]. Due to the predictability of such toxicity, the American Heart Association has published guidelines that designate all cancer patients at risk of cardiotoxicity as stage A heart failure [154] and in need of aggressive cardiovascular risk modification in addition to chemotherapy-specific preventive measures.
Evidence suggests that prevention is far more efficacious than therapy. For example, in a recent study by Cardinale et al., the majority of patients with left ventricular dysfunction who were treated for heart failure within 4 months of their last anthracycline treatment had considerable improvement of left ventricular function than those who started treatment more than 6 months after chemotherapy [155].
Doxorubicin (Adriamycin) and its family of anthracyclines are antineoplastic agents with proven efficacy in treating hematological and breast malignancies. Given that this family of anthracyclines has the highest rate of myocardial injury, cardiotoxicity has been classically associated with this group of medications [156]. Although not based on long-term prospective studies, the cardiotoxicity can be divided into three phases: acute, subacute, and chronic. Acute cardiotoxicity starts within 24 h of administration and is usually asymptomatic. It occurs in 40–50 % of the cases. These include transient electrocardiographic changes such as nonspecific ST-T changes, QTc interval prolongation, premature atrial and ventricular contractions, and mild cardiac troponin leak. Subacute cardiotoxicity is characterized by interstitial myocardial edema and cellular infiltration. The chronic form may not become evident until 20 years after the last administration and is associated with progressive left ventricular dysfunction with a 50 % 5-year mortality rate [157–159].
The molecular mechanisms associated with the cardiomyopathy include oxidative stress, myofibrillar disintegration, topoisomerase-2b inhibition, endothelial apoptosis, and intracellular calcium dysregulation [160]. Interestingly, cardiomyopathy development is largely dose dependent [161] but may occur at low doses due to increased susceptibility. For example, female gender, young age, coadministration of other cardiotoxic drugs, mediastinal radiotherapy, and other comorbidities represent strong clinical risk factors indicating susceptibility [162]. Since the deleterious effects of doxorubicin on the myocardium are often not detected until years after cancer remission, the greatest impact is on pediatric cancer survivors [150].
Sunitinib, sorafenib, and bevacizumab are vascular endothelial transduction pathway inhibitors used in the treatment of solid tumors. The incidence of cardiomyopathy for these agents can be up to 13 % across clinical trials [163]. Not only did these agents directly cause cardiotoxicity, they have been implicated in the development of clinically significant hypertension, as well [164]. Trastuzumab is a tyrosine kinase inhibitor monoclonal antibody used in HER2+ breast cancer patients. Coadministration with anthracyclines has resulted in heart failure in about 27 % of cases [165]. Finally, the radiation used as an adjuvant or primary therapy can result in a myriad of effects on the heart. This includes restrictive cardiomyopathy and diastolic heart failure, pericardial disease, and valvular dysfunction [166].
Early detection of myocardial damage might enable implementation of preventive measures that could reduce the likelihood of further ventricular decompensation. Cardiac biomarkers such as cardiac troponins and atrial natriuretic peptides (ANPs) can be important indicators of myocardial injury and can provide useful diagnostic information, especially when used in combination with myocardial imaging studies [167]. Serial echocardiographic monitoring is generally used as a first-line imaging modality [168]. Left ventricular systolic function can be evaluated traditionally using the ejection fraction or, more recently, by speckle echocardiography.
For example, in the National Surgical Adjuvant Breast and Bowel Project (NSABP) , a randomized phase III trial of trastuzumab in the 1830 cancer patients with HER2-positive breast cancer, borderline low ejection fraction at baseline carried a hazard ratio of 6.72 for the subsequent occurrence of heart failure [169]. Subsequently, in the Herceptin Adjuvant (HERA) trial, a lower baseline ejection fraction was reported to be a risk factor for adverse cardiac outcomes, including cardiovascular mortality, cardiomyopathy, and heart failure [170]. From another prospective, speckle analysis is a useful measure of myocardial deformation, which is an intrinsic mechanical property of the myocardial tissue. Reduced radial strain is associated with the onset of early histological damage and myocardial injury, offering an earlier method of detection compared to standard assessment of ventricular function [171].
Although echocardiography remains the cornerstone of cardiotoxicity screening, cardiac magnetic resonance imaging is evolving as a more superior and sensitive imaging entity for diagnosis and follow-up in the field of cardio-oncology. It offers the advantage of earlier cardiotoxicity detection—within weeks of administration [159]. Signs of cardiotoxicity primarily include myocardial edema, as it appears on T2-weighted images [172, 173]. T2 images are performed with a dual-echo technique at our institution (the University of Minnesota) to improve potential detection of signal abnormality relative to normal myocardium. In fact, a recent study showed that 11 % of cancer survivors were misdiagnosed as having normal global systolic function on echocardiography, while they were abnormal on magnetic resonance imaging [174] (◘ Fig. 18.8). Finally, cardiac magnetic resonance imaging can be particularly valuable in detecting myocardial fibrosis, a chronic manifestation of cardiotoxicity. Further investigation is needed to fully assess its impact on patient outcomes in the coming years.


Fig. 18.8
Magnetic resonance imaging of chemotherapy-induced cardiomyopathy . (a) T1 image demonstrating the cardiac structures in a four-chamber view. In later stages, it presents with a dilated left ventricle (LV) with reduced ejection fraction. (b) Late gadolinium-enhanced image (short axis view) showing the lack of overt fibrosis in the myocardium
With all this evidence, several groups of investigators have supported efforts to prevent the occurrence of advanced cardiomyopathy, rather than treatment of end-stage disease. Currently, dose modulation is the single most important strategy to prevent cardiomyopathy in patients undergoing chemotherapy. Several studies have reported that a lower weekly dosage, or even continuous infusion, will suppress tumors adequately while limiting cardiotoxicity [175].
BB and ACEI medications have been successfully used when cardiomyopathy develops [155]. A recent meta-analysis of the role of cardioprotective therapy for primary prevention of cardiotoxicity with chemotherapy reviewed 12 randomized controlled trials and two observational studies [176]. Prophylaxis with an ACEI, BB, or dexrazoxane was found to be beneficial in improving the primary outcome which was primarily heart failure and ejection fraction decline. The current practice most often dictates closely following patients at risk during treatment for their cancer and starting treatment for asymptomatic left ventricular dysfunction as detected by one of the imaging modalities. Once there are signs of cardiomyopathy, standard heart failure therapy should be implemented, starting with medical therapy. In its severe forms, refractory cases can benefit from cardiac resynchronization therapy, durable mechanical circulatory support, and transplantation [177]. Transplantation is particularly relevant given the history of malignancy. Nonetheless, it is an acceptable treatment option for patients with intractable cardiac failure secondary to chemotherapeutic agents. Fortunately, it has been shown that survival is comparable with all other heart recipients and that tumor recurrence after transplantation is rare, even with immunosuppression [178]. Guidelines regarding the duration of a cancer-free interval before listing should be reexamined on an individual basis, with input from the oncologist and consideration of type of tumor, stage, grade, and response to initial therapy .
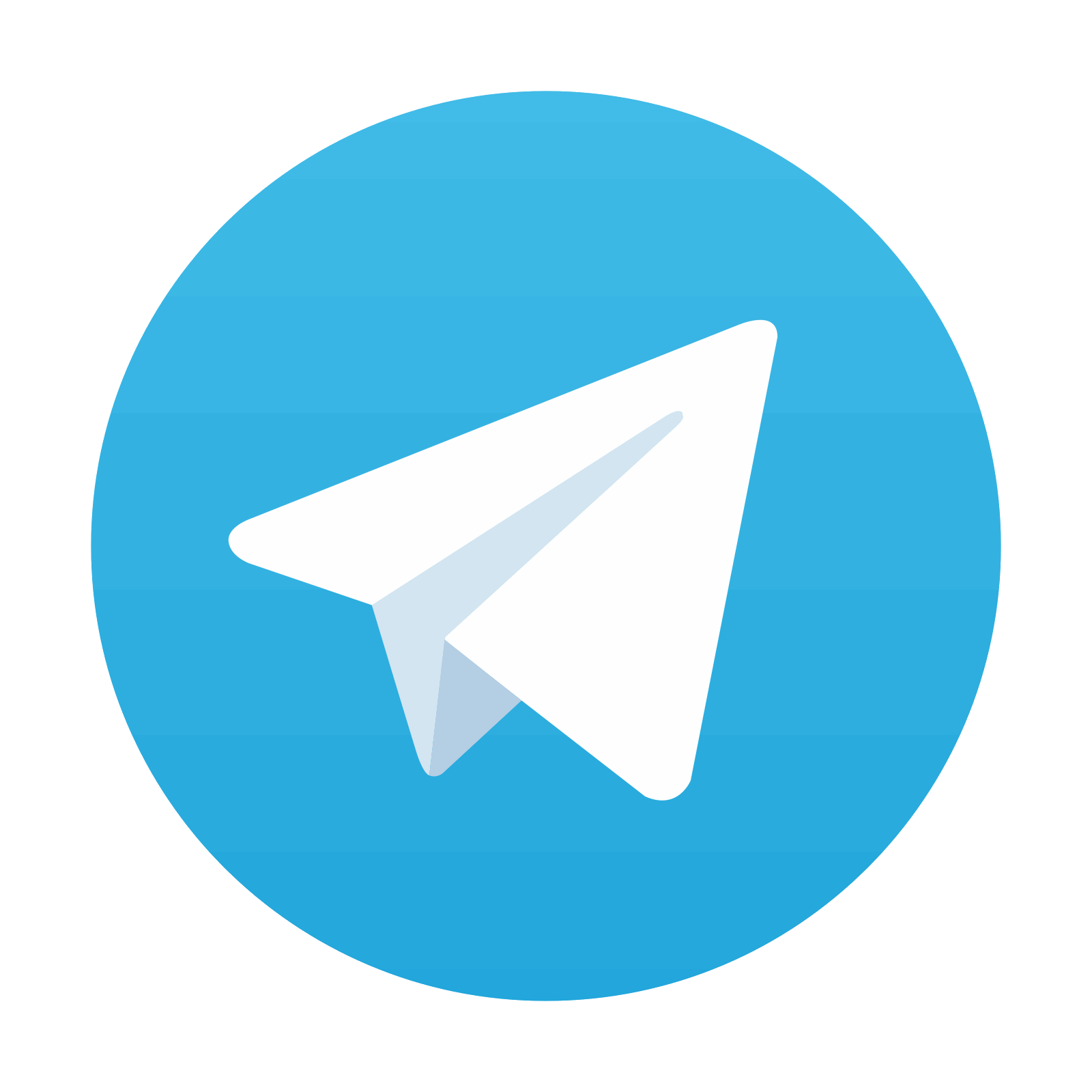
Stay updated, free articles. Join our Telegram channel

Full access? Get Clinical Tree
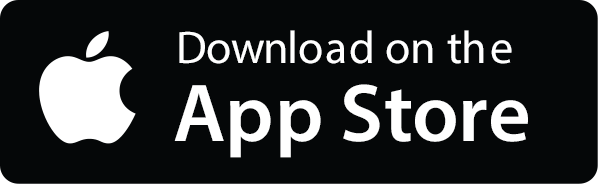
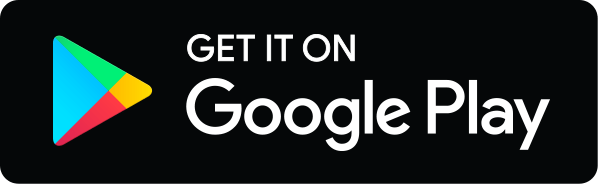