Heart Failure Pathophysiology
Luise Holzhauser
Paul J. Mather
INTRODUCTION AND CLASSIFICATIONS
Regardless of the etiology, heart failure (HF) is a complex clinical syndrome that results from any structural or functional impairment of ventricular filling or ejection of blood. The cardinal manifestations of HF are dyspnea and fatigue, which may limit exercise tolerance and cause or lead to fluid retention, which may lead to pulmonary and/or splanchnic congestion and/or peripheral edema. Some patients have exercise intolerance but with little evidence of fluid retention, whereas others complain primarily of edema, dyspnea, or fatigue. The left ventricular ejection fraction (LVEF) is a crude measure of actual cardiac function but is the clinically most widely accepted method of HF characterization and prognostication.
The categories of HF have recently been updated as follows: (1) HF with reduced EF (HFrEF), which includes patients with symptomatic HF and LVEF less than or equal to 40%; (2) HF with mid-range EF (HFmrEF), which includes patients with symptomatic HF and LVEF 41% to 49%; (3) HF with preserved EF (HFpEF), which includes patients with symptomatic HF and LVEF more than 50%; and (4) HF with improved EF (HFimpEF), which identifies patients with symptomatic HF with a baseline LVEF less than or equal to 40%, a greater than or equal to 10-point increase from baseline LVEF, and a second measurement of LVEF more than 40%.1
The causative pathophysiology is considered to be distinctly different between HFrEF and HFpEF despite a certain degree of overlap in risk factors. HFrEF is caused by either an inciting event or an inherited mechanism eventually leading to loss of cardiomyocytes, dysfunction, and dilation of the left ventricle (LV), resulting in pump failure setting off a vicious cycle of neurohormonal activation triggering further disease progression. HFpEF, on the other hand, is less a primary cardiac phenomenon but rather the cardiac manifestation of a systemic pathology. It is hypothesized that comorbidities defined by pro-inflammatory states—such as hypertension, diabetes, chronic obstructive pulmonary disease, obesity, and chronic kidney disease—eventually lead to cardiomyocyte hypertrophy and resultant stiffening of the heart muscle leading to increased filling pressures.2 Thus, although inflammation is implied among all spectrums of HF, inflammatory biomarkers are more strongly associated with HFpEF, and endothelial dysfunction and reduced bioavailability of nitric oxide are hallmarks of HFpEF pathophysiology.3
This chapter focuses on classic concepts of HFrEF pathophysiology.
VICIOUS CYCLE OF NEUROHORMONAL ADAPTIVE AND MALADAPTIVE MECHANISMS IN HEART FAILURE WITH REDUCED EJECTION FRACTION
Independent of the inciting event of HFrEF, the ultimate consequence is a reduced cardiac output (CO) detected by baroreceptors in the peripheral circulation as a low volume state leading to activation of both the renin-angiotensin-aldosterone system (RAAS) and the sympathetic nervous system (SNS). CO is maintained via increase in both contractility and heart rate (HR) accompanied by increased circulatory volume via salt and water retention and peripheral vasoconstriction. This initial adaptive response, triggered by neurohormonal activation to maintain cardiac hemostasis, ultimately leads to adverse remodeling in conditions of persistent stress. The degree of neurohormonal activation correlates with disease severity and prognosis in HF.4 Notably in chronic HFrEF, there is decrease in baroreceptor activity, increase in chemosensitivity to hypoxia and hypercarbia leading to further enhanced neurohormonal activation, and imbalance between SNS and parasympathetic nervous system with decreased parasympathetic tone manifest as enhanced HR, myocardial contractility, and peripheral vasoconstriction.5
SNS activation triggers release of renin via vasoconstriction of the renal artery and renal hypoperfusion as well as direct effects via β1-receptors on the juxtaglomerular apparatus.6 An additional trigger for renin release is decreasing chloride in the macula densa of the loop of Henle, which may be worsened by loop diuretics. Renin converts circulating angiotensinogen secreted by the liver to angiotensin I, which is then cleaved by angiotensin-converting enzyme (ACE) to angiotensin II. Angiotensin II is a potent vasoconstrictor and the most potent stimulator of aldosterone release from the adrenal glands. Both angiotensin II at the proximal tubule and aldosterone at the distal tubule increase salt and water retention. In chronic HF non-osmotic and angiotensin II triggered release of arginine-vasopressin from the thirst center in the brain further decrease free water clearance in the kidney and thus contribute to hyponatremia.4,7
RAAS activation is a pivotal player in the pathophysiology of HF, and chronic RAAS activation is a hallmark of adverse cardiac remodeling, fluid retention, and clinical manifestation of HF (Algorithm 66.1).4 Several short chain peptides
including angiotensin 1-7 are derived from angiotensin II and counteract its effects on adverse LV remodeling.8
including angiotensin 1-7 are derived from angiotensin II and counteract its effects on adverse LV remodeling.8
With the recent development of angiotensin receptor-neprilysin inhibition (ARNI), the important role of the natriuretic peptide systems has been recognized, including natriuretic peptide and B-type natriuretic peptide that are released following myocardial and atrial stretch and counteract adverse downstream effects of RAAS activity extraction via cGMP production, but peripheral resistance to natriuretic peptides in HF is common.4,9
The unique benefit of the ARNI compound lies in antagonizing downstream effects of angiotensin II and at the same time augmenting natriuretic peptide function by inhibiting neprilysin-mediated cleavage of these peptides. Thus, it augments vasodilation and natriuresis inhibited by natriuretic peptide resistance.4
Peripheral vascular vasoconstriction in HF is mediated via β1-receptors, and it augments blood pressure at the cost of reduced renal blood flow and increased myocardial afterload. In addition, neurohormonal activation enhances vascular tone, resulting in increased filling of the failing LV, thereby contributing to adverse remodeling, pump failure, and enhanced neurohormonal activation.4
MOLECULAR AND CELLULAR CHANGES
LV remodeling is driven by chronic neurohormonal activation, resulting in myocardial molecular structural and cellular changes, including alterations in myocyte biology and gene expression.
![]() ALGORITHM 66.1 The vicious cycle of neurohormonal activation in HFrEF. A decrease in cardiac output and blood pressure leads to activation of the sympathetic nervous system and the renin-angiotensin-aldosterone system. The resulting increase in preload and afterload as well as heart rate and contractility maintain cardiac output and perfusion. However, this initially adaptive process leads to adverse remodeling and sets off the vicious cycle of neurohormonal activation in chronic HF. ANP, atrial natriuretic peptide; BNP, B-type natriuretic peptide; HFrEF, heart failure with reduced ejection fraction. BP, blood pressure; CO, cardiac output; HR, heart rate; H20, water; LV, left ventricular; Na, sodium |
Myocardial Structural Changes and Extracellular Matrix
Initially, cardiomyocyte hypertrophy is compensatory and occurs in either a concentric pattern in cases of pressure overload with addition of sarcomeres in parallel or an eccentric pattern with elongated myocytes and sarcomeres added in sequence as a response to volume overload. With ongoing myocardial stress, remodeling transitions from adaptive to maladaptive, characterized by myocyte elongation, sarcomere disarray, decrease in α-myosin heavy chain (MYH6) gene expression, and increase in β-myosin heavy chain (MYH7) gene.10 α-Myosin heavy chain (MHC) has a higher adenosine triphosphate (ATP)ase activity and shortening velocity; thus, hearts expressing MYH6 have higher contractile velocity than hearts expressing β-MHC. This shift in relative gene expression is reminiscent of expression levels of the fetal gene program of these contractile proteins in the embryonic heart.11
Neurohormonal activation with elevated norepinephrine and angiotensin II levels triggers necrotic cardiomyocyte death.12,13 The human heart has minimal regenerative capacity, and cardiomyocyte necrosis, apoptosis, and autophagy are commonly observed in the failing myocardium.4,14 Aside from cardiomyocytes loss, molecular and structural alterations within the extracellular matrix (ECM) also play a pivotal role in the adverse remodeling process. The ECM not only preserves tissue integrity and facilitates force transmission, but it also serves as
a signaling hub for cardiomyocytes and as a reservoir of growth factors allowing for a fast response to injury with activation of a repair program.15 RAAS activation stimulates myocardial fibrosis via fibroblast proliferation and collagen production.16
a signaling hub for cardiomyocytes and as a reservoir of growth factors allowing for a fast response to injury with activation of a repair program.15 RAAS activation stimulates myocardial fibrosis via fibroblast proliferation and collagen production.16
As with cardiomyocytes, different injury patterns lead to different structural changes in the ECM, including activation of a matrix-synthetic program in cardiac fibroblasts with conversion to myofibroblasts and synthesis of ECM proteins. The resulting expansion in ECM can increase myocardial stiffness, thereby aggravating diastolic dysfunction of the failing LV. Further, conditions of pressure overload lead to dilative remodeling and systolic failure, possibly mediated by changes in the interstitial metalloproteinase-protease/antiprotease balance. In ischemic injury patterns, however, more dynamic changes in the cardiac ECM contribute to regulation of inflammation and repair and can mediate adverse cardiac remodeling.17
Any cardiac injury, however, leads to initiation of tissue repair mechanisms, which are closely linked to innate immunity. Tumor necrosis factor α (TNF-α), transforming growth factor β (TGF-β), and interleukins (IL) including IL-1, IL-12, IL-8, and IL-18 are major mediators of this pro-inflammatory response. TNF-α and IL-1 have negative inotropic effects mediated via β-receptor uncoupling and impaired calcium (Ca2+) signaling, which negatively affects diastolic function.18,19 In addition to adversely affecting excitation-contraction coupling, TNF-α triggers hypertrophy of cardiomyocytes and ECM remodeling via regulation of tissue inhibitors of metalloproteinases.20,21 Although preclinical data showed beneficial effects of inhibiting TNF-α signaling, clinical trials using anti-TNF therapy showed an increased risk of all-cause death and/or HF hospitalization. This could in part be explained by inhibiting nuclear factor-κB (NF-κB), a transcription regulator and key effector of TNF-α. Aside from mediating inflammation, apoptosis, and ECM remodeling, NF-κB also has cardioprotective effects, including antioxidant activity, improving mitochondrial function, and inhibiting cell death.3 This example describes the delicate balance between a necessary inflammatory response to initiate tissue repair, especially in acute ischemic injury, and the deleterious effects of persistent inflammation aggravating the adverse remodeling process.
![]() ALGORITHM 66.2 Myocardial structural and molecular changes of adverse remodeling. Adverse remodeling is characterized by myocardial molecular structural and cellular changes, including alterations in myocyte biology, excitation-contraction coupling, and gene expression. Dephos, de-phosphorylation; LV, left ventricular; Na/Ca, sodium-calcium; RyR2, ryanodine receptor; SERCA, sarcoplasmic-endoplasmic reticulum Ca2+ ATPase. |
In advanced HF injury, neurohormonal activation and inflammation eventually lead to changes in cellular composition of the failing myocardium and alterations in LV ECM, with chamber dilation, wall thinning, and change in shape toward a spherical appearance further aggravating LV dysfunction.4
Molecular Changes in the Failing Heart β-Receptor Signaling
Persistent SNS activation with high levels of catecholamines further aggravates pathologic changes in the heart, and sustained β-receptor stimulation has been shown to correlate with worsening LV dysfunction and mortality.22 Chronic Ca2+ overload mediated by β-receptor signaling may contribute to cardiomyocyte death.23 β1-Receptors mediate cardiac function via the stimulatory G-protein (guanyl nucleotide binding protein [Gs]) pathway, adenylyl cyclase, and subsequent cAMP generation. Downstream activation of protein kinase A (PKA) then leads to phosphorylation of several targets, including phospholamban (PLB) and the sarcoplasmic/endoplasmic reticulum calcium ATPase (SERCA), thereby maintaining intracellular Ca2+ dynamics, lusitropy (rate of myocardial relaxation), and positive inotropy. The β1-receptor is the main receptor subtype expressed by cardiomyocytes with a ratio of 4:1 to β2-receptors. In the failing heart, however, chronic adrenergic stimulation leads to downregulation of β1-receptors with a 1:1 ratio of β1 and β2 as well as decreased sensitivity of cardiomyocytes to endogenous and exogenous catecholamines with decoupling from downstream G-protein activation.24,25 The process of β-receptor downregulation and decoupling is initialed by phosphorylation via G protein-coupled receptor kinase (GRK), thus facilitating internalization of the receptors mediated via β-arrestin (Algorithm 66.2).
Along with β-arrestin, other mediators are recruited, including mitogen-activated protein kinase (MAPK). These signaling pathways are crucial components of adverse remodeling and apoptosis. The main kinase involved in the phosphorylation process is elevated in HF and inhibited by β-blocker therapy.4,26,27
Along with β-arrestin, other mediators are recruited, including mitogen-activated protein kinase (MAPK). These signaling pathways are crucial components of adverse remodeling and apoptosis. The main kinase involved in the phosphorylation process is elevated in HF and inhibited by β-blocker therapy.4,26,27
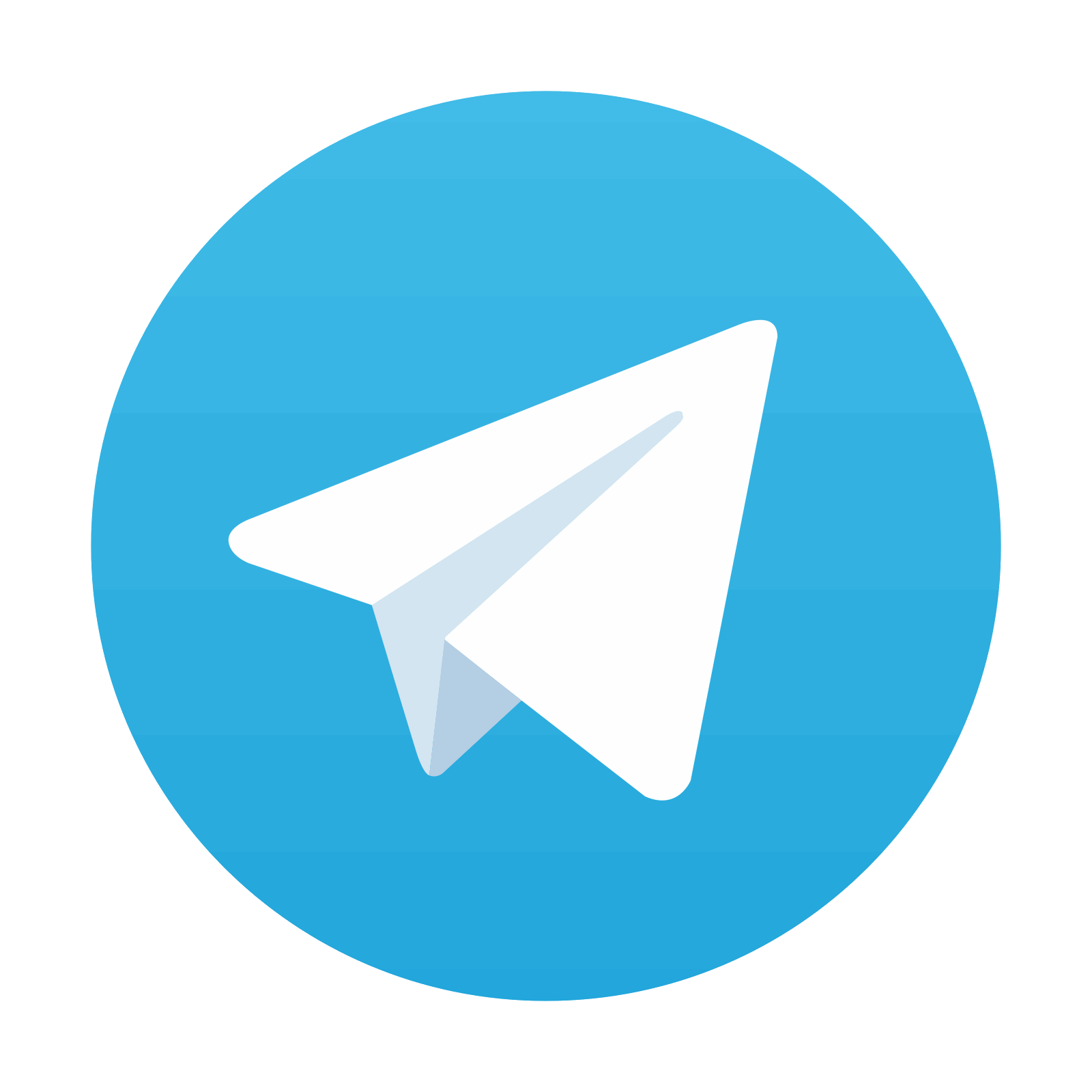
Stay updated, free articles. Join our Telegram channel

Full access? Get Clinical Tree
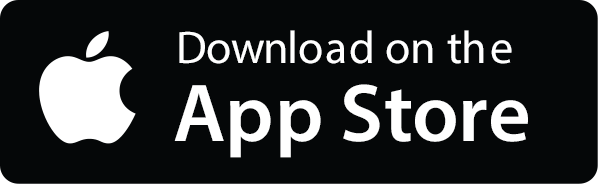
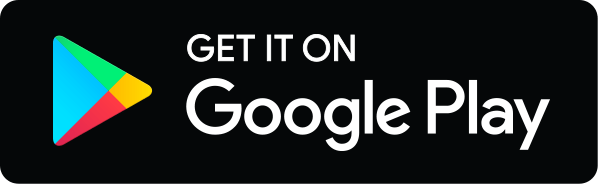
