CHAPTER 4 Heart failure, myocardium and pericardium
4.1 HEART FAILURE
Some studies (e.g. Framingham study) have provided epidemiological data on heart failure:
The term dilated cardiomyopathy describes large hearts with reduced contractile function in the presence of normal coronary arteries (section 4.4). It is usually of unknown cause. When a cause is established, the term is sometimes preceded by a qualifier, such as alcoholic dilated cardiomyopathy. Hypertension has become a less common cause of heart failure as a consequence of its improved detection and treatment. It remains an important contributory factor to the progression of heart failure and is a risk factor for coronary artery disease.
Causes of chronic heart failure
Myocardial disease | |
Systolic failure | |
Dyskinesia, diffuse dysfunction, aneurysm, incoordination, stunning, hibernation | |
β-blockers, calcium antagonists, antiarrhythmic drugs | |
Diastolic failure | |
Arrhythmias | |
AF, VT, supraventricular tachycardia (SVT) | |
Complete heart block | |
Pericardial diseases | |
Valve dysfunction | |
Aortic stenosis | |
Mitral or aortic regurgitation | |
Mitral or aortic stenosis | |
Shunts | |
Extracardiac disease | |
‘High output’ failure | Anaemia, thyrotoxicosis, pregnancy, glomerulonephritis, AV fistula, Paget’s disease of bone, beri-beri |
Adapted from Kaddoura & Poole-Wilson, 1999, Cardiology, McGraw-Hill, pp. 523–533.
It is always important to seek the cause of worsening features (decompensation) of heart failure in a previously clinically stable individual. This may lead to symptoms such as breathlessness or signs such as crackles in the chest, raised venous pressure or peripheral oedema. Echo can help in the investigation of the potential causes:
Causes of acute heart failure and cardiogenic shock
Adapted from Holmberg, 1996, in Diseases of the Heart, Saunders, pp. 456–466 and Dob, 2003, in Oh’s Intensive Care Manual, Butterworth-Heinemann.
4.2 ASSESSMENT OF LV SYSTOLIC FUNCTION
M-mode (Fig. 4.1) can be used to assess LV cavity dimensions, wall motion and thickness. The phrase, ‘a big heart is a bad heart’, carries an important element of truth – poor LV systolic function is usually associated with increased LV dimensions. This is not always the case, e.g. if there is a large akinetic segment of LV wall or an apical LV aneurysm following MI, systolic function may be impaired due to regional wall motion abnormalities but M-mode measurements of LV dimensions may be within the normal range.
LVEDD is at the end of diastole (R wave of ECG). The normal range is 3.5–5.6 cm.
A number of techniques are available. Simpson’s method (Fig. 4.2) divides the LV cavity into multiple slices of known thickness and diameter D (by taking multiple short-axis views at different levels along the LV long axis) and then calculating the volume of each slice (area × thickness). The area is π(D/2)2. The thinner the slices, the more accurate the estimate of LV volume. Calculations can be made by the computer of most echo machines. The endocardial border must be traced accurately and this is often the major technical difficulty. Endocardial definition has improved with some newer echo technology (e.g. harmonic imaging) and automated endocardial border detection systems are available on some echo machines. The computer calculates LV volume by dividing the apical view into 20 sections along the LV long axis.
An estimate of cardiac output can be obtained using LV volumes:
Regional LV wall motion
The LV can be divided up on 2-D imaging of apical 4-chamber and parasternal short-axis views into segments (9 or 16) and an assessment can be made of these segments (Fig. 5.12). This can be useful at rest and in stress echo to determine the location of coronary artery disease (Ch. 5).
A segment’s systolic movement may be classified as:
4.3 CORONARY ARTERY DISEASE
Echo plays an increasingly important role in assessing coronary artery disease. Resting and stress echo (Ch. 5) techniques are used in:
Assessment of ischaemia
Ischaemia results in immediate changes which can be detected by echo:
These can be detected by 2-D echo but M-mode is also extremely good because its high sampling rate makes it very sensitive to wall motion and thickening abnormalities. It is essential that the beam is at 90º to the wall. There are limited regions of the LV myocardium that can be examined by M-mode – most usefully, the posterior wall and IVS (Fig. 4.3).
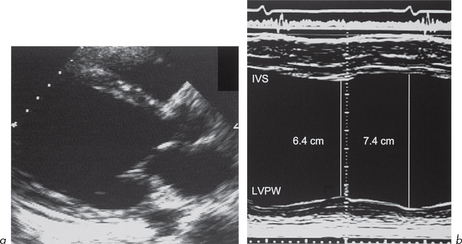
Fig. 4.3 (a) and (b) Dilated left ventricle with impaired systolic function due to coronary artery disease.
Prediction of artery involved
This is done by dividing the LV into segments as described (Figs 5.12 and 5.13). Stress echo is based on this.
Assessment of myocardial infarction
Echo can help in detecting the extent of LV infarction, assessing RV involvement and detecting complications. The changes in LV function with acute MI are similar to those described for ischaemia, but rapidly become irreversible. Detection of RV involvement is important in determining treatment and prognosis (section 4.6).
Complications of myocardial infarction
Many of the complications of acute MI can be detected by echo.
In the following 2 complications (acute MR and acute VSD), LV systolic function is very active, unlike the situation above.
Echo assessment of coronary artery anatomy (Fig. 4.6)
Abnormalities are more likely to be seen during TOE, e.g.
Useful information from echo in patients with heart failure
Left ventricle
Valves
4.4 CARDIOMYOPATHIES AND MYOCARDITIS
The most important idiopathic cardiomyopathies are:
1. Hypertrophic cardiomyopathy
The clinical features include:
The characteristic feature is myocardial hypertrophy in any part of the ventricular wall:
Echo is diagnostic of HCM. The important echo features are seen using both M-mode and 2-D imaging:
Continuous wave Doppler shows increased peak flow through the LVOT. Pulsed wave Doppler with the sample volume in the LVOT proximal to the AV shows that the increase in velocity occurs below the level of the valve, distinguishing the obstruction from valvular aortic stenosis. The peak in maximal velocity across the AV is often bifid in HCM. There may also be features of LV diastolic dysfunction due to LVH (e.g. abnormal transmitral flow pattern with E-wave smaller than A-wave, section 4.5).
2. Dilated cardiomyopathy
This is characterized by dilatation of the cardiac chambers, particularly the LV (although all other chambers are often involved) with reduced wall thickness and reduced wall motion (Fig. 4.8). The incidence is estimated at 6.0 per 100 000 per year. Most cases are isolated although some familial forms have been identified. The reduced LV wall motion is usually global rather than regional, as seen in LV systolic impairment due to coronary artery disease (ischaemia or infarction).
Doppler studies may show functional MR and TR.
3. Restrictive cardiomyopathy
Echo features of restrictive cardiomyopathy
Infiltration
The findings are similar whatever the underlying cause. Amyloid is the most common infiltrative disease (Fig. 4.9). The features are: