Fig. 4.1
Etiologies of heart failure (HF) differ between adults with acquired heart disease and those with congenital heart disease. In HF due to any cause, plasma levels of neurohumoral factors and inflammatory cytokine are increased, cardiac autonomic nervous activity is abnormal, the endothelium is dysfunctional, and both metabolic abnormalities and arrhythmias are frequently observed. These deficits reduce exercise capacity, further exacerbating cardiovascular pathology
The first part of this review systematically describes HF pathophysiology of ACHD patients. The second part describes three major postoperative pathophysiologies, namely, those resulting from the repair of tetralogy of Fallot (TF), morphological RV as a systemic ventricle, and Fontan circulation. Finally, current knowledge on the management of ACHD patients with HF is summarized.
4.2 Heart Failure Pathophysiology in ACHD
Heart failure is a complex pathophysiology that generally involves many noncardiac organs and organ−organ interactions (Fig. 4.2). Furthermore, postoperative ACHD patients have unique complexities that may involve pulmonary, autonomic, myocardial, conduction, arterial, and renal dysfunction as described below.


Fig. 4.2
In patients with heart failure, both cardiac and noncardiac organs are impaired. These impairments often interact, leading to additional clinical entities, such as cardiorenal syndrome
4.2.1 Pulmonary Dysfunction
The primary function of the cardiopulmonary system is to deliver oxygen to all organs efficiently, and the oxygen uptake capacity of the respiratory system imposes a critical limit on oxygenation. In patients with left-side HF, the lungs become edematous and poorly compliant due to a rise in left atrial pressure, leading to a smaller vital capacity (VC). As a result, the breathing pattern during physical activity is characterized by “rapid-and-shallow” respiration where the ventilatory efficiency progressively worsens [7]. Cardiac operations can result in multiple deleterious effects on the VC of ACHD patients [8], of which restrictive ventilatory impairment is the most common. A high prevalence of scoliosis is also seen in these patients, which has a negative impact on VC [8]. In addition, surgery-related phrenic nerve palsy reduces pulmonary gas exchange. All of these complications must be checked in routine practice because low VC has an adverse impact on ACHD prognosis [8], although the underlying mechanisms are unclear. At the same time, vagal nerve input to control cardiac sympathetic tone may decrease because of small tidal volume, which can be detected by the reduced power of the high-frequency component of heart rate (HR) variability, i.e., diminished respiratory sinus arrhythmia [9]. Restrictive ventilatory impairment and the loss of respiratory sinus arrhythmia may have adverse effects on pulmonary circulation as well as pulmonary gas exchange and may lead to hypoxia, especially in ACHD patients with right-side HF and abnormal pulmonary circulation. Furthermore, small lung capacity is a risk factor for reoperation and heart transplantation.
Recently, sleep apnea due to obesity has been recognized as an important factor contributing to HF. Obesity is one of the strongest and most common risk factors for future cardiovascular disease, and there is a growing evidence of high obesity prevalence in ACHD patients [10]. Thus, the growing obesity epidemic may further increase HF incidence/severity in ACHD by promoting sleep apnea. Such patients may benefit from respiratory assist devices.
4.3 Dysfunctional Cardiac Autonomic Nervous Activity (CANA)
The central nervous system controls the cardiovascular system through projecting sympathetic and parasympathetic nerves. In healthy adults, sympathetic and parasympathetic activities are well balanced. In non-ACHD HF patients, sympathetic dominance occurs because of diminished parasympathetic (vagus) nerve activity [11, 12]. This imbalance is usually proportional to HF severity and thus predicts prognosis. In healthy adults, low systemic blood pressure is sensed by the arterial baroreflex center, and HR increases through the suppression of vagal inputs (reduced vagal tone). Sympathetic dominance in HF can be detected by the high washout ratio of the norepinephrine analogue metaiodobenzylguanidine (MIBG) as measured by scintigraphy, which reflects the accelerated turnover of norepinephrine at sympathetic nerve terminals. These physical phenotypes of HF, low systemic blood pressure, high HR, loss of HR variability, and high washout ratio of MIBG usually parallel HF severity and predict poor prognosis [13]. Thus, assessing CANA status provides useful information on HF pathophysiology in typical non-ACHD patients.
However, the associations between CANA and HF severity differ in postoperative ACHD because of the inevitable damage to sympathetic nerves during cardiac surgery, which interrupts central nervous system signals to great vessels, conduction systems, and atrial and ventricular myocardia ([14], Fig. 4.3). The type and number of cardiac surgeries determine the degree of CANA abnormality. These abnormalities are mainly due to surgical denervation, and severe CANA abnormality can resemble that after cardiac transplantation. The effects of sinus node and ventricular myocardium denervation and damage are detailed below.


Fig. 4.3
Most repaired patients with congenital heart disease (CHD) as well as those with simple lesions show impaired cardiac autonomic nervous activity. HRV heart rate variability (a), AVSD atrial/ventricular septal defect, BRS arterial baroreflex sensitivity (b), CoA/IAA coarctation/interruption of the aorta, DCM dilated cardiomyopathy, HF high frequency, H/M ratio of heart to mediastinal metaiodobenzylguanidine (MIBG) activity (c), RVOTR right ventricular outflow tract reconstruction, WR washout ratio of MIBG (d)
4.3.1 Sinus Node Injury
Surgical maneuvers around the superior vena cava or right atrium may injure the sinus nodal artery, leading to sinus node dysfunction and slower HR. In addition, postsynaptic hypersensitivity to vagal acetylcholine may be involved in this pathophysiology [15]. Slower resting HR is frequently seen in athletic adults because of enhanced vagal tone. However, lower HR in postoperative ACHD patients with low aerobic capacity usually indicates an impaired function of the sinus node, in part, due to perioperative surgical insults [16]. Thus, some ACHD patients show inappropriately low HR that is insufficient to compensate for impaired hemodynamics and low systemic blood pressure. In addition, it is frequently difficult to maintain proper systemic blood pressure during rapid postural change because of a diminished vagally mediated quick HR response. Thus, β-receptor stimulation or atrial pacing, rather than atropine infusion, may be effective for increasing HR. Atropine infusion is effective only when vagally mediated HR is preserved.
4.3.2 Ventricular Myocardium Injury
Ventricular denervation is inevitable during arterial switch operation for the transposition of the great arteries (TGA) and Ross operation for aortic valvular disease because these procedures involve a complete transection of the cardiopulmonary nerves that run along the right and left coronary arteries [17]. Therefore, MIBG scintigraphy is not an appropriate tool to estimate sympathetic nervous activity in these ACHD patients, and this may also hold true to some extent in other surgically treated ACHD patients, especially those with a history of multiple cardiac surgeries [9]. Cardiac reinnervation can occur after these procedures as it does after cardiac transplantation; however, there is currently no effective treatment for promoting reinnervation as the factors promoting natural reinnervation are unknown. Further, postoperative ACHD patients may not complain of any chest pain from cardiac origin even if they have significant myocardial ischemia from coronary artery stenosis because of sensory denervation, especially after arterial switch or Ross operation. Thus, stress electrocardiography (ECG) and/or imaging should be applied to detect the stenotic lesion(s) of coronary arteries in these ACHD patients instead of symptoms.
4.4 Ventricular Dysfunction
The etiologies of ventricular dysfunction include pressure overload due to outflow stenosis, volume overload due to valvular regurgitation and intra/extracardiac shunting, hypoxia before definitive repair, the intrinsic problem of RV myocardium as a systemic ventricle, ischemia due to coronary artery stenosis, tachycardia-induced dysfunction, and intra/interventricular dyssynchrony [5]. In addition, the restrictive changes of the ventricle due to pericardial adhesion and myocardial fibrosis have adverse influences on ventricular function and make HF pathophysiology more complex in postoperative ACHD patients [18, 19].
Pharmacological approaches have limited efficacy in the management of pressure and volume overload due to structural abnormalities; hence, surgical or catheter-based interventions may be required in some ACHD patients. Pulmonary valve replacement (PVR) is one of these interventions [20]; however, the optimal timing of this procedure is still debated. Late definitive repair may predispose cyanotic patients to a subclinical progression of myocardial fibrotic change due to long-standing hypoxia and volume overload [21]. Right ventricular and tricuspid valve (TV) functions should be carefully monitored in ACHD patients with RV as a systemic ventricle to assess suitability for anatomical tricuspid valve replacement.
The conventional anti-HF strategy using angiotensin-converting enzyme inhibitors/angiotensin receptor blockers (ACEIs/ARBs) and β blockers may be effective for dilated LV with systolic dysfunction in some ACHD patients with relative tachycardia. However, these strategies may be harmful in postoperative ACHD patients with sinus node dysfunction and ventricular restrictive physiology. Furthermore, the long-term efficacy of these conventional strategies is uncertain for subpulmonary ventricular systolic and diastolic dysfunctions. In these scenarios, atrial pacing with appropriate HR corresponding to HF severity may be effective, although finding the optimal HR may be challenging.
Cardiac resynchronized therapy (CRT) has been applied to postoperative ACHD HF patients with some efficacy [22]. The electrophysiological assessment of QRS duration or various imaging modalities may reveal ventricular dyssynchrony; however, a standard diagnostic method has not been established, especially for patients with non-LV as a systemic ventricle. CRT focusing on the longitudinal direction in systemic RV or the site opposite two-chamber dyssynchrony in Fontan circulation with unbalanced ventricles may be effective for some cases [23].
Right ventricular restrictive physiology characterized by end-diastolic forward flow in the main pulmonary artery is a pathophysiology unique to postoperative ACHD patients, especially repaired TF patients; however, the clinical significance is controversial [24, 25]. The restrictive pathophysiology is one phenotype of the RV diastolic dysfunction and may differ from other types of RV diastolic dysfunction characterized by other criteria, such as HF with preserved RV ejection fraction [18, 19], a more severe subtype of RV pathophysiology. Management strategies for these diastolic dysfunctions are not yet established as the strategies have not been established in HF with preserved LV ejection fraction.
4.5 Electrophysiological Dysfunction
Electrophysiological abnormalities are often associated with atrial and/or ventricular remodeling. The QRS duration reflects RV volume in postoperative TF and systemic ventricular volume in Fontan patients [26–29]. Fragmented QRS complex in adult patients with Ebstein anomaly is associated with risk of arrhythmia and with the severity of the anomaly [30]. The electropathophysiology and its relations with clinical profiles and management strategies are further described in Chap. 5.
4.6 Aortopathy
In the general population, metabolic disorders and obesity lead to endothelial dysfunction, which increases the risk of arteriosclerosis. In turn, arteriosclerosis and hypertension are strong factors predisposing to HF. Arteriosclerosis and hypertension also contribute to HF in ACHD patients. In addition, stiffened dilated ascending aorta, histologically characterized by medial necrosis in the aortic wall, i.e., aortopathy, resembling that of Marfan syndrome, has been described in various types of ACHD, particularly TF [31]. The stiffened dilated aorta has adverse impacts on ventricular function by augmenting blood pressure reflection and decreasing diastolic pressure, i.e., greater pulse pressure, resulting in ventricular pressure overload with impaired coronary perfusion [32]. This may in turn reduce exercise capacity [33] and contribute to metabolic syndrome, obesity, hypertension, arteriosclerosis, and ultimately HF. As demonstrated in Marfan syndrome, ACEIs/ARBs may be beneficial by preventing further progression of aortopathy in some selected ACHD patients.
4.7 Renal Dysfunction
Renal dysfunction due to cardiovascular disease, termed cardiorenal syndrome, has a strong impact on cardiovascular prognosis [34]. ACHD patients are categorized as typical type II cardiorenal syndrome when chronic cardiac problems lead to renal dysfunction [35, 36]. Elevated central venous pressure (CVP) in ACHD patients with right-sided HF causes renal congestion, whereas low systemic blood pressure results in lower perfusion pressure in the kidney. This vicious interaction may be associated with poor cardiovascular prognosis [37].
Sinus node dysfunction with blunted HR response and stiffened arterial and venous vessels are frequently combined in postoperative ACHD patients [9, 38, 39]. As a result, all organs are perfused through stiffened great vessels by a cardiovascular system with a limited ability to homeostatically regulate cardiac output, especially during stress. For instance, dehydration by any cause may lead to prerenal failure because of impaired baroreflex function, sinus node dysfunction, and less compliant vessels.
4.8 Reduced Exercise Capacity
Impaired exercise capacity is one of the cardinal features of chronic HF. The causes of this impairment are multifactorial [6]. Peak oxygen uptake (VO2) during cardiopulmonary exercise testing (CPX) is the gold standard metric of exercise capacity. The following equations explain the major determinants of peak VO2:

4.9 Biomarkers
Biomarkers are important assessment tools for HF severity in ACHD patients [43]. The clinical significance of plasma natriuretic peptide (NP) levels, especially brain NP (BNP), has been most intensively studied and validated in some cases. However, the clinical utilities of other possible markers, such as catecholamines and renin–angiotensin–aldosterone system hormones, have not been as extensively examined. Plasma NP levels are already increased in asymptomatic ACHD patients with HF irrespective of whether RV is a subpulmonary or systemic ventricle. The determinants of elevated plasma NP include RV volume overload due to intracardiac shunting, pulmonary and/or AV valve regurgitation, RV dysfunction due to pressure overload, and hypoxia due to unrepaired complex lesions or Eisenmenger syndrome (ES). Plasma NP levels are frequently lower in postoperative ACHD patients than in adults with non-ACHD HF even if NYHA class is equivalent [9, 18, 44], and the cutoff value for prognosis is usually lower in postoperative ACHD patients because of the restrictive physiology. Nevertheless, the high levels of NP and catecholamine predict poor prognosis, including mortality in ACHD patients with NYHA class ≥ III [unrepaired, ES, and pulmonary hypertension (PH)] [45]. In particular, a progressive increase in plasma BNP during follow-up strongly predicts mortality [46, 47]. However, evidence for the prognostic efficacy of biomarkers is mainly based on studies including a relatively small number of ACHD patients with mild HF, mostly NYHA class ≥ II (repaired TGA or TF patients). These limitations weaken the associations between biomarker levels and cardiac function. However, in adult Fontan patients, the high plasma levels of NP and catecholamines are associated with poor prognosis [48, 49]. In addition, BNP reflects RV volume reduction after PVR and improved PH after medical treatment [50, 51]. Therefore, measuring plasma biomarkers can provide clinically useful information for assessing HF pathophysiology, especially in complex ACHD patients. Although the clinical significance of higher plasma NP for assessing ACHD patients with stretched atrial and/or ventricular myocardium (congestive HF) is well established, abnormally low NP may be a reflection of ventricular underfilling due to low cardiac output, for instance, from dehydration, which is also a critical pathophysiology. Thus, comprehensive HF assessment by multiple modalities, including physical examination, is mandatory for the care of ACHD patients.
The unique HF pathophysiology of ACHD characterized by surgery-related CANA abnormality and restrictive ventricular function is presented schematically in Fig. 4.4, together with a comparison of clinical variables among postoperative patients with CHD, those with dilated cardiomyopathy (DCM, no surgery), and healthy controls. The two patient groups are comparable in terms of systemic ventricular EF and functional capacity. However, they show distinct hemodynamics, CANA variables, and plasma BNP levels. Finally, HF pathophysiology is summarized in Fig. 4.5. Some of these pathophysiological features are, to some extent, applicable to the following specific postoperative ACHDs.



Fig. 4.4
Comparison of clinical variables among postoperative patients with congenital heart disease, dilated cardiomyopathy patients without surgery, and controls. The two patient groups are comparable in terms of systemic ventricular ejection fraction and functional capacity. *, **, and *** = p < 0.05, 0.01, and 0.001, vs. DCM, respectively

Fig. 4.5
The pathophysiology of postoperative ACHD is summarized
4.10 Specific Pathophysiology
4.10.1 Tetralogy of Fallot
TF is the most common cyanotic CHD. Thirty years after definitive repair, postoperative survival rate is more than 90% [52]. However, despite this low long-term mortality, repaired adult TF patients have significant postoperative residual lesions and complications that include RV dysfunction due to RV outflow problems [pulmonary stenosis and/or pulmonary regurgitation (PR)] [53], LV dysfunction partially due to RV–LV interactions [54, 55], and clinically relevant atrial and/or ventricular arrhythmias, which may cause sudden cardiac death [56].
4.10.2 Right Ventricular Problems
TF is occasionally considered as a disease of the RV infundibulum; hence, management strategies may be focused on preserving infundibulum structure and function [57]. There is wide agreement on interventions for significant RV outflow stenosis because it is a major determinant of exercise capacity [18] and is closely associated with RV hypertrophy and clinically relevant arrhythmias. However, mild residual RV outflow stenosis may mitigate the need for PVR, probably due to lower PR and a positive RV–LV interaction [58, 59]. Significant RV volume overload due to PR is tolerable for a long time; however, PR-related RV dilatation ultimately becomes a main driver of adult TF pathophysiology, and PVR is required in some patients with severe PR [53]. Although firm criteria for PVR are not yet established, repaired TF patients with a RV volume ≥150–170 (mL/m2) plus other clinical findings, such as QRS ≥ 180 ms, exercise intolerance, and significant ventricular arrhythmia, are considered good candidates for PVR. A meta-analysis of PVR in repaired TF revealed significant clinical benefits, including RV volume reduction, LV volume increase with improved EF due to positive RV–LV interactions, improvement of NYHA class, and shortening of QRS duration [20]. Better exercise capacity predicts better postoperative course after PVR [60]; however, the long-term prognostic impact of PVR for TF is unknown [61]. Regarding tricuspid regurgitation (TR), which is also common in late after the TF repair, significant TR has an adverse impact on prognosis in normal structural heart [62]. However, its impact on morbidity and mortality remains unclear in repaired TF. Recent study showed little beneficial impact of concomitant TV repair of TR (≥ moderate) on short to mid-term postoperative RV function and TR when compared with those without TV repair at the time of PVR although both RV function and TR improve significantly in both groups [63].
4.10.3 Restrictive Physiology (RP)
RP is a unique RV pathophysiology defined by the presence of diastolic forward flow in the main pulmonary artery [24], which can be seen in both pathophysiologies with large and small RV volumes [64]. However, the clinical significance remains unclear [18, 64]. RP is seen in pediatric TF patients with poor postoperative course [25], whereas RP prevents PR and contributes to preserved exercise capacity in repaired adult TF patients [24, 64]. Fibrotic change and/or the extracardiac restriction of RV due to pericardial issues may be responsible for RP. Right ventricular fibrotic change is closely associated with the impaired ventricular function as well as poor prognosis in these patients. In addition, diastolic dysfunction defined by echocardiographic variables, including those from tissue Doppler imaging, is associated with a high prevalence of ventricular tachycardia [65]. Furthermore, there is an additional severe phenotype of RV pathophysiology, the RV-version of HF with preserved EF (RV-HFpEF) [18]. Similar to the LV-version of HFpEF, there is currently no standard management strategy for RV-HFpEF.
4.10.4 LV Deficits
Low LVEF (≤55%) is not uncommon in ACHD (45% of patients) [66] and is a risk factor for sudden death. The rate of appropriate shock by an implantable cardioverter-defibrillator is high in repaired TF patients with LV end-diastolic pressure ≥ 12 mmHg [67], and poor LV function is a risk factor for unsuccessful anti-arrhythmic catheter ablation [68]. Furthermore, the prognostic power of LV dysfunction may be greater than that of RV dysfunction [69].
4.10.5 Stratification of HF
Plasma BNP level and CPX-derived variables can be used to stratify HF severity in repaired adult TF patients. Plasma BNP ≥ 52 pg/mL predicts fivefold higher mortality [70]. However, plasma BNP level alone may underestimate HF severity because of possible RP, which can lower myocardial stress even if end-diastolic pressure is high ([9, 18, 44], Fig. 4.4). Low peak VO2 (≤36% of predicted normal value) and low ventilatory efficiency (high VE/VCO2 slope ≥ 39) predict high mortality in these patients [71].
4.10.6 Management of HFs
RCTs examining medical management of repaired TF patients have been few [72]. In one RCT, β blockers and ACEIs/ARBs were ineffective in the overall analysis [73], but subanalysis indicated that 6-month administration of ACEI (Ramipril) improved LVEF with significant volume reduction in selected repaired TF patients with RP [74]. Another study found favorable results using CRT with biventricular stimulation in a small number of patients (n = 5) [75]. Larger-scale RCTs are required before this procedure can be applied in daily practice.
4.11 Transposition of the Great Arteries with RV as a Systemic Ventricle
Dextro-TGA (d-TGA) is the most common cyanotic CHD followed by TOF. It is managed by atrial switch operation (Mustard/Senning procedure: venous switch operation [VSO]) or arterial switch operation (Jatene procedure). VSO contributed to the dramatically improved survival rate of d-TGA in the 1960s. However, long-term postoperative pathophysiology has emerged that includes RV dysfunction as well as TR and rhythm disturbance [26, 76, 77]. Congenitally corrected TGA (c-TGA) is a rare form of CHD with pathophysiology resembling that of d-TGA after VSO in which RV acts as a systemic ventricle and features RV dysfunction, TR, and arrhythmias [78]. Congenitally c-TGA patients frequently have combined lesions, such as pulmonary stenosis, ventricular septal defect, Ebstein anomaly, and AV block, all of which have significant negative impacts on prognosis.
The overall 40-year post-VSO survival rate is approximately 50%; however, the survival rate is approximately 70% if the patient survives the immediate postoperative period [76]. Although the impact of atrial switch procedure type, either Mustard or Senning, on mortality is still unclear, combined lesion(s), pulmonary stenosis, and ventricular septal defects increase mortality [76]. In adult c-TGA patients, the prevalence of clinical HF and moderate to severe RV dysfunction at 45 years of age is 25–30% for those without combined lesions and 60–70% for those with substantial combined lesions [78]. The possible etiologies of RV dysfunction include myocardial ischemia and infarction [79, 80] associated with reduced coronary flow reserve and the resulting limitation on exercise capacity as well as the single coronary physiology of RV [81, 82]. Systemic RV fibrosis is associated with ventricular dysfunction and poor prognosis [77], although the cause of RV fibrosis remains unclear. However, a causal relationship between RV dysfunction and TR has not been established.
4.11.1 Assessment of Systemic RV function
The QRS duration and QTc reflect RV volume and RVEF, respectively, whereas QT dispersion is associated with clinical arrhythmia [27]. During follow-up, these ECG parameters worsen in ACHD patients with RV pressure load [83]. The Tei index and RV longitudinal strain are useful echocardiographic variables to evaluate RV function and predict prognosis [84, 85]. Plasma BNP levels are also useful to assess RV dysfunction and TR severity [86, 87]. Plasma BNP ≥ 85 pg/mL may predict cardiac events in repaired d-TGA after VSO [88]. Intriguingly, moderate pulmonary outflow stenosis protects against HF in c-TGA patients, probably because of a shift of the interventricular septum, which prevents the TV orifice from dilating and promotes positive RV–LV interactions [85], leading to better prognosis [89].
4.11.2 Exercise Pathophysiology
Exercise capacity and related systemic blood pressure responses are impaired in d-TGA and c-TGA patients [90–92], and these impairments are associated with poor prognosis [92]. RVEF and Tei index predict exercise capacity [93]. Rigid (less compliant) atrium may be responsible for the blunted increase in stroke volume during exercise in adults after VSO, resulting in lower exercise capacity, whereas this mechanism may not be applicable to adult c-TGA patients [94]. Interestingly, the rate of cardiac events, including AV block, is lower in c-TGA patients with dextrocardia (situs inversus) than those with levocardia (situs solitus) [95].
4.11.3 Management of Adults with d-TGA or c-TGA
The ARB, valsartan, may prevent exercise capacity from declining during follow-up in d-TGA and c-TGA patients, although no benefits of valsartan on RV remodeling or cardiac events have been demonstrated [96]. On the other hand, exercise training is effective for improving exercise capacity [97], and CRT may be effective in selected patients [22, 23]. For severe TR, TV replacement surgery rather than TV plasty is recommended before RVEF falls below 40% [98, 99].
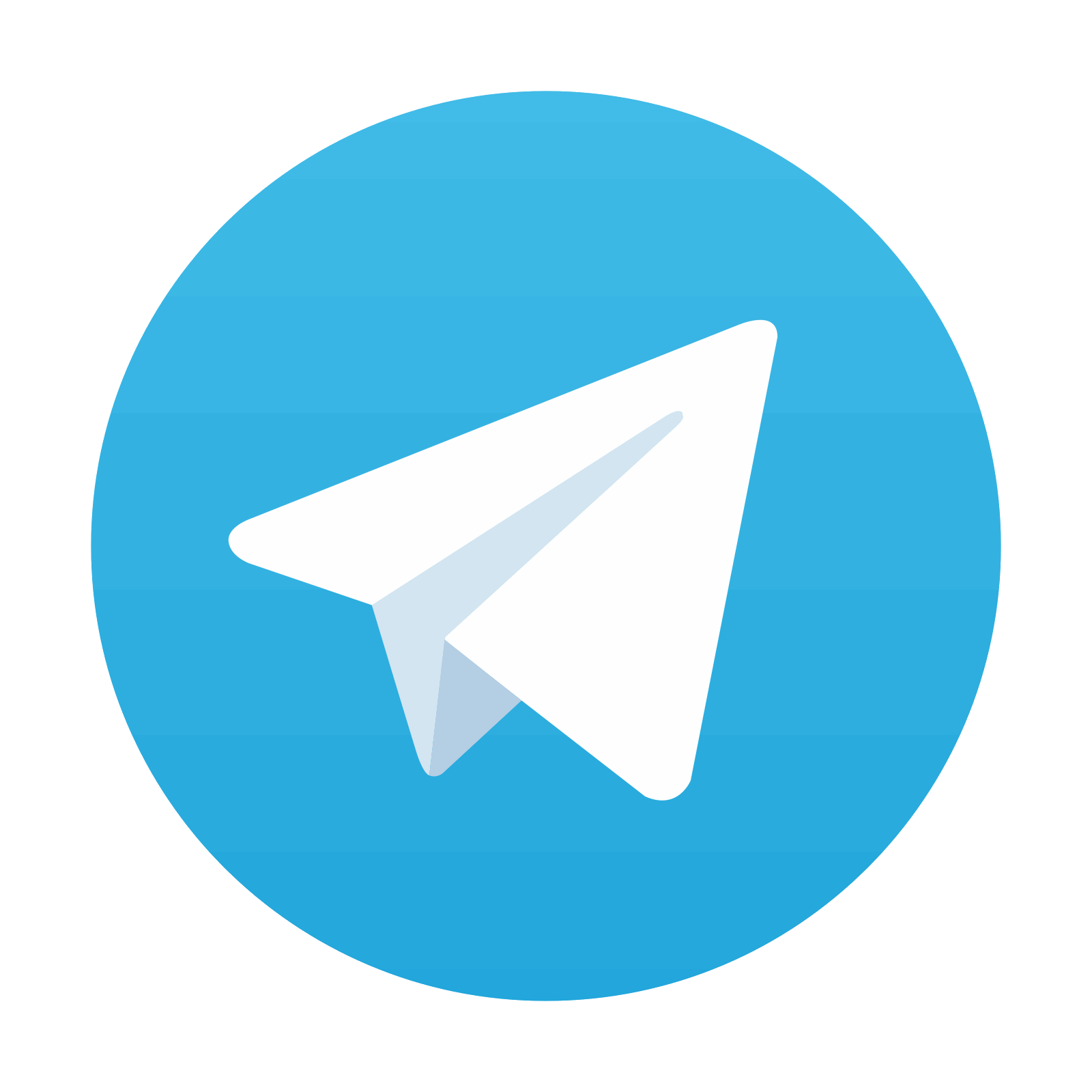
Stay updated, free articles. Join our Telegram channel

Full access? Get Clinical Tree
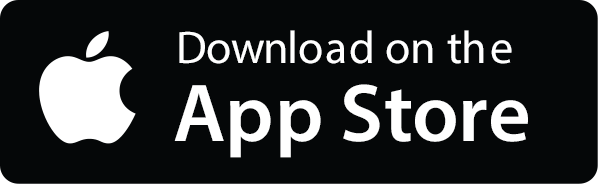
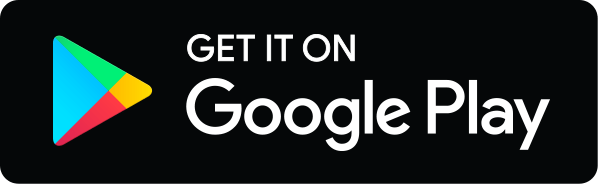