Outline
Overview, 311
Definition, 312
Epidemiology, 312
Molecular Genetic Basis of Hypertrophic Cardiomyopathy, 313
Pathogenesis, 314
Effects of the Mutations on Transcription and Translation, 314
Effects of Mutations on Sarcomere Assembly and Function, 315
Molecular Pathways Liking the Initial Defects to the Phenotype, 315
Determinants of the Clinical Phenotype of Hypertrophic Cardiomyopathy, 315
Phenotypic Features of Hypertrophic Cardiomyopathy, 316
Clinical Manifestations, 318
Chest Pain, 318
Heart Failure, 318
Ventricular Arrhythmias and Sudden Cardiac Death, 319
Supraventricular Arrhythmias, 319
Prognosis, 319
Management of Patients With Hypertrophic Cardiomyopathy, 319
Genetic Testing, 319
Management of Risk of Sudden Cardiac Death in Hypertrophic Cardiomyopathy, 320
Management of Patients With Left Ventricular Outflow Tract Obstruction, 320
Management of Cardiac Arrhythmias, 320
Experimental Therapies, 321
Summary and Future Directions, 321
Overview
Henri Liouville, a French pathologist, described the first case of hypertrophic cardiomyopathy (HCM) in 1869. The patient was an elderly woman with symptoms of heart failure, a ventricular wall thickness of 3.5 to 4 cm and left ventricular outflow tract (LVOT) obstruction. Dr. Liouville described the LVOT obstruction as “Rétrécissement cardiaque sous aortique,” which literally translates to subaortic cardiac. Since this initial description, there has been considerable interest in the unusual clinical and pathological manifestations of HCM.
Alexander Schmincke described diffuse muscular “hyperplasia” at the LVOT and pointed out the vicious cycle of left ventricular hypertrophy and outflow tract obstruction, perpetuating each other. Robert L. Levy and William C. Von Glahn described HCM as “Cardiac Hypertrophy of Unknown Etiology in Young Adults” in 1933. William Evans reported the familial nature of “idiopathic cardiomegaly” in 1949 and constructed the first documented pedigree of a family with HCM. Donald Teare also noted the familial nature of HCM in a young adult patient with severe cardiac hypertrophy. J.A.P. Paré and colleagues described the autosomal dominant mode of inheritance of HCM in a large French-Canadian family, whose genetic mutation was identified by Christine Seidman and colleagues three decades later.
Lord Russell Brock in England and Andrew Glenn Morrow and Eugene Braunwald in the United States recognized the dynamic nature of LVOT obstruction in HCM and concluded that the obstruction was due to “systolic narrowing of the LVOT resulting from massive muscular hypertrophy.” Dr. Braunwald and colleagues coined the term “idiopathic hypertrophic subaortic stenosis.” They also described the unique hemodynamic feature known as the Brockenbrough phenomenon and characterized left ventricular diastolic dysfunction as a major feature of HCM, independent of LVOT obstruction. These observations led Dr. Morrow and colleagues to introduce trans-aortic surgical myectomy in the 1960s, which is known as the Morrow operation.
Advances in echocardiographic imaging led to the next phase of discoveries in HCM and the description of asymmetric septal hypertrophy (ASH) and the assessment of LVOT obstruction by Doppler imaging. Echocardiography soon emerged as the most commonly used imaging tool in diagnosing HCM, the assessment of its severity, the detection of diastolic dysfunction, and the estimation of the LVOT gradient. Likewise, tissue Doppler and spectral imaging modalities provided valuable information on regional myocardial contraction and relaxation abnormalities as well as diastolic function. These imaging modalities also offer utilities in the preclinical and early identification of family members who carry the causal mutations for HCM.
The seminal discovery of the first causal mutation in familial HCM by Drs. Christine and Jon Seidman in 1990 ushered in the era of molecular genetics. The discovery, which was made in the original French-Canadian family described by Paré 30 years earlier, shifted the paradigm of HCM from being an “obscure” disease to primarily a genetic disease of sarcomere and sarcomere-associated proteins. The genetic discoveries brought forth the routine applications of genetic testing in the diagnosis and management of patients with HCM.
Ulrich Sigwart introduced alcohol septal ablation for the reduction of LVOT obstruction in three patients who reported marked and rapid improvement in their symptoms. The technique commonly known as percutaneous alcohol septal ablation has become an alternative modality to Morrow’s procedure for the treatment of selected symptomatic HCM patients with LVOT obstruction who are refractory to medical therapy.
Progress in diagnosis and management of patients with HCM has continued unabated. Notable among the recent progresses is the use of defibrillators (implantable cardioverter-defibrillator, [ICD]) in prevention of sudden cardiac death (SCD) in HCM as an effective intervention, particularly in the secondary prevention of SCD. In recent years a number of randomized clinical trials, albeit small-scale initial phase II and III studies, with new pharmacological agents have been initiated and are expected to pave the way for larger-scale efficacy studies. The findings could expand our pharmacopeia beyond the β blockers, which were established by Dr. Braunwald and colleagues, as the cornerstone of medical therapy for HCM more than half a century ago. Finally, development of new patient-specific and genetic-based therapies are being developed, which might fulfill the goal of tailoring the treatment to the individual patient.
Definition
HCM could be defined as a genetic disease of cardiac myocytes that grossly manifests with cardiac hypertrophy; often asymmetric, and a nondilated left ventricle with a preserved or increased ejection fraction ( Fig. 23.1 ). Thus, by definition, cardiac hypertrophy caused by loading conditions is excluded. Cardiac hypertrophy is commonly concentric but occasionally involves a specific left ventricular wall or apex. It is asymmetric with a predominant involvement of the interventricular septum in about quarter of the cases.

Epidemiology
HCM is a relatively common disease with an estimated prevalence of 1:500 (0.16% to 0.29%) in the world population. Population frequency of HCM might be higher than that estimated based on the expression of cardiac hypertrophy with a wall thickness of 15 mm or greater, as expression of hypertrophy is age-dependent and often absent in the young individuals. It is estimated that approximately 50% of those with the underlying causal HCM mutations develop HCM by the third decade of life, ∼75% by the fifth decade of life, and 95% by 50 years of age. Because of the age-dependent penetrance of HCM mutations, HCM is more prevalent in the older populations, estimated at 0.29% (44/15,137) in the 60-year-old individuals who have had an echocardiogram.
In the clinical diagnosis of HCM, phenocopy conditions should be considered, which might be present in 5% to 10% of those with the clinical diagnosis of HCM, particularly the children. In addition, the presence of concomitant conditions leading to increased load, such systemic arterial hypertension, could complicate the clinical diagnosis, hence estimating the true prevalence of HCM. A genetic-based diagnosis might facilitate the accurate diagnosis of HCM in such cases ( see also Chapter 24 ).
Molecular Genetic Basis of Hypertrophic Cardiomyopathy
HCM, a classic single gene disorder, is a familial disease in approximately two-thirds of cases and with an autosomal dominant pattern of inheritance. HCM with an autosomal recessive or an X-linked mode of inheritance is rare. The latter typically raises the possibility of a phenocopy condition, such as Fabry disease.
Pioneering studies by Christine and Jon Seidman have led to the discovery of the first causal gene for HCM, namely the MYH7 gene, which encodes sarcomere protein β-myosin heavy chain (MYH7), in the French-Canadian family that was originally described by Paré. Subsequently, additional causal genes, including MYBPC3 , TNNT2 , TPM1 , and TNNI3 , which encode myosin-binding protein C proteins, cardiac troponin T, α-tropomyosin, and cardiac troponin I, respectively ,were mapped and identified. Given that the well-established causal gene for HCM encodes sarcomere proteins, HCM is considered primarily a disease of sarcomere protein ( Fig. 23.2 ). Since the initial discoveries, over a dozen causal genes encoding sarcomere proteins and sarcomere-associated proteins, and thousands of mutations for HCM have been identified ( Table 23.1 ).

Gene | Protein | Function |
---|---|---|
Causal Genes for HCM (strong evidence for causality) | ||
MYH7 | β-Myosin heavy chain | ATPase activity, force generation |
MYBPC3 | Myosin binding protein-C | Cardiac contraction |
TNNT2 | Cardiac troponin T | Regulator of actomyosin interaction |
TNNI3 | Cardiac troponin I | Inhibitor of actomyosin interaction |
TPM1 | α-tropomyosin | Places the troponin complex on cardiac actin |
ACTC1 | Cardiac α -actin | Actomyosin interaction |
MYL2 | Regulatory myosin light chain | MYH7 binding protein |
MYL3 | Essential myosin light chain | MYH7 binding protein |
CSRP3 | Cysteine and glycine-rich protein 3 | Muscle LIM protein (MLP), a Z disk protein |
Causal Genes for HCM (moderate level evidence for causality) | ||
FHL1 | Four-and-a-half LIM domains 1 | Muscle development and hypertrophy |
MYOZ2 | Myozenin 2 (calsarcin 1) | Z disk protein |
PLN | Phospholamban | Regulator of sarcoplasmic reticulum calcium |
TCAP | Tcap (Telethonin) | Titin capping protein |
TRIM63 | Muscle ring finger protein 1 | E3 ligase of proteasome ubiquitin system |
TTN | Titin | Sarcomere function |
Genes Associated with HCM (causality not definitive) | ||
ACTN2 | Actinin, α-2 | Z disk protein |
ANKRD1 | Ankyrin repeat domain 1 | A negative regulator of cardiac genes |
CASQ2 | Calsequestrin 2 | Calcium binding protein |
CAV3 | Caveolin 3 | A caveolae protein |
JPH2 | Junctophilin2 | Intracellular calcium signaling |
LDB3 | Lim domain binding 3 | Z disk protein |
MYH6 | Myosin heavy chain α | Sarcomere protein expressed at low levels in the adult human heart |
MYLK2 | Myosin light chain kinase 2 | Phosphorylate MYL2 |
NEXN | Nexilin | Z disc protein |
TNNC1 | Cardiac troponin C | Calcium sensitive regulator of myofilament function |
VCL | Vinculin | Z disk protein |
The list of established causal genes for HCM includes ACTC1 (cardiac α-actin), MYL2 (myosin light chain 2), MYL3 (myosin light chain 3), and CSRP3 (muscle LIM protein). In addition, TTN (titin), TCAP (telethonin), MYOZ2 (myozenin 2), TRIM63 (ubiquitin E3 ligase tripartite motif protein 63 or MuRF1), and FHL1 (four and a half LIM domain 1) also are likely causal genes for HCM. Finally, mutations in TNNC1 (cardiac troponin C), MYH6 (myosin heavy chain or α-myosin heavy chain), PLN (phospholamban), CAV3 (caveolin 3) , ALPK3 (α kinase 3) , and JPH2 (junctophilin 2) have been associated with HCM.
It is evident that HCM is a genetically heterogeneous disease. MYH7 and MYBPC3 are the two most common causal genes and together are responsible for approximately half of the familial HCM. TNNT2 , TNNI3 , and TPM1 are relatively uncommon causes of HCM and collectively are responsible for less than 10% of the HCM cases. The remainder of the causal genes are even less common. Overall, the known causal genes account for about two-thirds of the HCM cases.
The majority of the causal mutations are missense mutations. Premature truncating mutations are rare with the exception of MYBPC3 mutations, which are often insertion/deletion and frameshift mutations, leading to premature truncation of the encoded protein. The frameshift mutations are often transcribed into unstable mRNAs, which are targeted for degradation by the nonsense-mediated decay (NMD). Similarly, whenever a premature truncated protein is expressed it is typically degraded by the ubiquitin proteasome system (UPS), leading to haploinsufficiency. Rare deletion mutations in MYH7 , TNNT2, and others also have been reported.
Population frequency of a specific HCM mutation is low and most mutations are either rare or private mutations. A notable exception is the p.Arg502Trp mutation in MYBPC3 , which has been reported to occur in ∼2.4% of HCM patients. Likewise, the p.Val762Asp mutation in the MYBPC3 gene has been reported in 3.9% of the Japanese population. A few hot spots for mutation also have been reported. Moreover, mutations do not exhibit an aggregation in a specific domain of the encoded protein, with the exception of mutations in MYH7 , which show a predilection toward the globular head and hinge region of the protein, albeit mutations in the rod domain of MYH7 , which have also have been described. Finally, MYH7 and MYBPC3 are the two most common causal genes in apical HCM.
The causal gene in approximately one-third of HCM remains unknown. The so-called missing causal genes typically pertain to the sporadic cases, where establishing the causality of the genetic variants unambiguously is exceedingly challenging if not impossible. This is in contrast to large families where the causal genes and mutations could be unambiguously identified through cosegregation and linkage analysis. The difficulty in identifying the “missing causal genes” in the sporadic cases, is in part intrinsic to genetic diversity of the humans, as each genome comprises about 4 million genetic variants, including about 11,000 nonsynonymous, and several thousand pathogenic variants. In addition, the majority of the genetic variants is rare and population-specific, which makes it challenging to identify the responsible causal variant in a sporadic case. In addition, genetic variants exert a gradient of effect sizes, ranging from large and disease causing to clinically negligible. Those with large effect sizes are expected to exhibit a high penetrance, cause familial disease where the variant cosegregates with inheritance of the phenotype. In contrast, variants with moderate effect sizes exhibit incomplete or low penetrance in a familial setting and are often found in sporadic cases. Therefore, unambiguous ascertainment of genetic causality in such situations is difficult.
Finally, a subset of HCM, estimated to be about 5%, is caused by compound mutations in the same gene or two different genes. Double mutations seem to be associated with a more pronounced phenotype. Likewise, a small subset of HCM might be caused by multiple pathogenic variants, and hence, might be oligogenic in nature.
Pathogenesis
The mechanistic molecular pathways connecting the causal mutations to the ensuing clinical and phenotypic features of HCM are diverse ( Fig. 23.3 ). The mechanistic events could be sequentially categorized into three groups, based on the proximity of the genetic mutations, as follows:
- •
Proximal effects of the causal mutations, namely transcription and translation of the mutant proteins;
- •
Effects of the mutations on sarcomere structure and function, such as assembly and ATPase activity;
- •
Molecular pathways that are activated in response to structural and functional defects in the myofilaments/sarcomeres and link the mutations to the phenotypic features, such as trophic and mitotic factors.

Each set of the mechanistic categories entail a diverse array of events, likely reflecting the underpinning biological effects of the involved codon and protein. The mechanisms are likely to differ for HCM caused by mutations in genes other than those coding for the sarcomere proteins, including phenocopy conditions.
Effects of the Mutations on Transcription and Translation
Proximal defects are defined as the direct and most proximal effect of the mutations on gene transcription, translation, integrity, and stability of the encoded transcripts and proteins, and incorporation of the encoded proteins into sarcomeres. In keeping with the diversity of the genes and mutations involved, the proximal phenotypes are also diverse.
HCM mutations are mostly heterozygous missense mutations, which are typically translated into proteins, each encompassing a change in a single amino acid. However, a change in the nucleotide sequence could affect the efficiency of transcription as well as the translation of the encoded mRNA into the corresponding protein. The suboptimal codon usage often leads to an allelic imbalance, which is often compensated for by the wild type allele. Thus, the majority of the missense mutations do not significantly affect, or only modestly affect, the expression levels of the corresponding proteins. However, it seems that there is considerable variability in the transcript levels of mutant alleles among myocytes and in different regions of the myocardium, which might also influence the phenotypic expression of HCM.
A fraction of HCM mutation is stop codon or insertion/deletion mutations that lead to premature truncation of the transcripts and the encoded proteins. The truncated transcripts and proteins are subject to several transcriptional and translational surveillance and quality control programs that target the prematurely truncated transcripts and proteins for degradation. Notable among them is the NMD pathway, which targets transcripts containing premature termination codon by releasing the elongation factors from the template and recruitment of the decay-inducing complex. Similarly, the prematurely truncated proteins are unstable and subjected to unfolded protein response and decay by UPS.
In scenarios where the causal mutation leads to a gain- or loss-of-stop codon or results in a frame shift, expression of the mutant protein is reduced, and the net effect is reduced expression level of the involved protein and even haplo-insufficiency. This seems to be the predominant mechanism for mutations in the MYBPC3 , which predominantly lead to premature truncation of the protein.
Effects of Mutations on Sarcomere Assembly and Function
The majority of the mutant proteins carrying the causal mutations are stably expressed and incorporate into sarcomeres and myofibrils. However, the mutation by altering the three-dimensional (3-D) structure of corresponding protein could affect protein-protein interactions and reduce incorporation of the mutant protein into sarcomeres. There is variability in the incorporation of the mutant proteins into sarcomere, which is expected to affect function of the assembled sarcomeres in cardiac myocytes.
In interpreting the results of functional data, it is important to consider the differences in the sarcomere protein composition between mice and humans. While the mouse predominantly expresses α-myosin heavy chain or MYH6 (>95%) in the heart, the β-myosin heavy chain or MYH7 is the predominant myosin isoform in the human heart, comprising >90% of the total myosin heavy chain. Differences in the MYH protein composition is relevant to functional studies of HCM mutations, as MYH6 and MYH7 show four- to six-fold differences in ATPase activities and actomyosin kinetics.
Mutations impart a diverse array of functional defects by affecting various components of the actomyosin complex, including calcium sensitivity of the troponin complex, ATPase activity of myosin heavy chain, and generation of the force of contraction upon displacement of the actin filament by myosin heavy chain globular head. Under physiological conditions, the thin actin filament is released from the actomyosin complex upon binding of ATP to the globular head of the myosin. In the presence of HCM mutations, the sensitivity of this complex in releasing actin, upon binding of ATP to myosin globular head, is reduced. Consequently, in any given moment during a cardiac cycle, a higher number of myosin and actin are in a bound state than in a dissociated state, compared to the physiological condition. This is expected to affect both the systolic and diastolic phases of cardiac cycle. Consequently, the maximal tension development per each unit of the ATP is hydrolyzed, and hence, myocardial efficiency of force generation, is lower in HCM compared to the physiological state. The effects of the mutations on the dissociation of actin and myosin upon ATP binding, and hence, myocardial energetic efficiency, are variable leading to different degrees of functional impairment. For example, mutations in MYH7 reduce myofibrillar ATPase activity more than those in the MYBPC3, resulting in a higher energy cost of tension generation for the MYH7 rather than the MYBPC3 mutations. These functional alterations, such as reduced myocardial energetic efficiency, precede cardiac hypertrophy, as the impairment is also observed in the prehypertrophic stage in individuals who carry HCM mutations. The increased energy cost of tension generation also correlates with the reduced ratio of cardiac phosphocreatine to ATP in the human hearts in HCM. Experimental data in a transgenic rabbit model of HCM, which has a cardiac myosin isoform composition closely resembling that in the human heart, show reduced calcium sensitivity of the myofibrils ATPase activity early and prior to the development of cardiac hypertrophy. Calcium sensitivity of maximum force generation also seems to vary among myocytes, which might be secondary to a variation in the expression levels of the mutant transcript. Finally, different regions of the myocardium show differences in the expression levels and functions, suggesting a mosaic molecular and functional effect in HCM.
The effects of mutations in thin filament proteins such as TNNT2 on sarcomere function seem to differ from mutations in the thick filaments, such as MYH7. Accordingly, mutations in the thick filament proteins enhance calcium sensitivity of force generation and ATPase activity of the myofilaments, as opposed to the effects of mutations in the thick filaments, which were discussed earlier. The effects also precede the development of cardiac hypertrophy in the murine models.
Molecular Pathways Liking the Initial Defects to the Phenotype
Defects in gene expression, sarcomere structure, and myocyte function, imparted by the HCM mutations, lead to activation of expression of large cadre of molecules, including the stress-responsive signaling pathways in the heart (see Fig. 23.3 ). These dysregulated pathways mediate induction of cardiac hypertrophy, fibrosis, morphological, and functional phenotypes in HCM. As would be expected, the nature of the dysregulated transcriptional and signaling pathways are diverse and vary according to the biological functions of the proteins involved and the nature of the mutations. Among the dysregulated pathways in HCM are the calcineurin, mitogen activated protein kinases, and the transforming growth factor β pathways. In addition, the noncoding RNA and epigenetic factors are also implicated. The dysregulated pathways collectively lead to the induction of cardiac hypertrophy, interstitial fibrosis, and other morphological, histological, and functional features of HCM (see Fig. 23.3 ).
Determinants of the Clinical Phenotype of Hypertrophic Cardiomyopathy
The clinical phenotypes of HCM are secondary events resulting from complex and stochastic interactions of a number of dysregulated pathways and the environmental factors ( Fig. 23.4 ). Consequently, a large number of factors contribute to the pathogenesis of clinical manifestations of HCM. Naturally, the causal mutations have the largest impact on the expression of the clinical phenotype; however, they are not the sole determinants, as the genetic background or the modifier genes and the additional pathogenic variants, the epigenetic factors, the noncoding RNAs, the posttranslational protein modifications, and the environmental factors all contribute to phenotypic expression of the disease (see Fig. 23.4 ). Nevertheless, despite the large effect size of the causal mutations, there is no clear genotype-phenotype correlation in HCM, and phenotypic expression of the disease is largely similar between MYH7 and MYBPC3, which are the two most common causal genes in HCM. The multiplicity of the phenotypic determinants along with their complex and stochastic interactions contribute to variability in the phenotypic expression of HCM ( Fig. 23.5 ).


The classic HCM is a single gene disorder with an autosomal dominant mode of inheritance. However, small families and probands have been described where multiple pathogenic variants seem to contribute to the pathogenesis of HCM. Thus, in a sense, a subset of HCM might be digenic and oligogenic in etiology. The presence of multiple pathogenic variants is associated with a more severe phenotype, including severe cardiac hypertrophy and outflow tract obstruction.
Several modifier genetic variants and loci have been implicated as determinants of severity of the phenotype. Notable among them is an insertion/deletion variant in the angiotensin-1-converting enzyme 1 gene ( ACE ), which is associated with variation in the plasma levels of ACE. The variant has been associated with the severity of cardiac hypertrophy as well as the risk of SCD in patients with HCM. In a mouse model of HCM the Fhl1 gene, encoding 4.5 LIM domains protein 1 is implicated as a possible modifier of cardiac hypertrophy and function.
Among the external factors, loading conditions, including systemic arterial hypertension, are expected to influence phenotypic expression of HCM, including severity of cardiac hypertrophy. The so-called hypertensive hypertrophic cardiomyopathy of the elderly might represent a condition wherein the concomitant presence of systemic arterial hypertension influences expression of sarcomere protein mutations or pathogenic variants in the sarcomere genes. Likewise, isometric exercises would be expected to enhance expression of cardiac hypertrophy in those who carry the HCM mutations or pathogenic variants in genes encoding sarcomere proteins.
There is considerable phenotypic overlap for the phenotypic expression of mutations in genes known to cause cardiomyopathies. The causal gene for HCM might also cause other forms of cardiomyopathies, particularly dilated cardiomyopathy (DCM), which phenotypically contrasts HCM. This is well illustrated for TNNT2 and MYH7 , which are known to cause either HCM or DCM. Differences in the phenotypic expression of the mutations in a given gene might reflect the effects of the mutations on interactions of the mutant protein with other proteins, as well as calcium sensitivity of the myofilaments. Mutations enhancing Ca +2 sensitivity of the myofibrillar force generation and ATPase activity could lead to HCM, whereas those reducing these functions lead to DCM.
Phenotypic Features of Hypertrophic Cardiomyopathy
Cardiac hypertrophy, the quintessential phenotypic feature of clinical HCM, develops primarily because of myocyte hypertrophy, and to some extent because of increased extracellular matrix volume. HCM is clinically diagnosed based on the detection of cardiac hypertrophy by a diagnostic modality, such as echocardiography. Cardiac hypertrophy is commonly defined as an end diastolic wall thickness of 13 mm or greater on an echocardiogram. In HCM, cardiac hypertrophy occurs in the absence of external loading conditions or is disproportionate to the external factors, and in the absence of other secondary causes, such as storage diseases. A left ventricular wall thickness of 15 mm or greater has been advocated as a more accurate indicator of hypertrophy in HCM, and it confers a greater specificity at the expense of reduced sensitivity in detecting HCM. Given that body size is a major determinant of cardiac size and wall thickness, a Z-score, reflecting deviation from an age- and sex-matched population average, is used in children to define cardiac hypertrophy.
Cardiac hypertrophy is commonly concentric, and in about a quarter of the cases it is asymmetric, predominantly involving the interventricular septum. Cardiac apex is occasionally the predominant site of involvement, which leads to the diagnosis of apical HCM. Such patients exhibit the characteristic deep T wave inversion in the precordial leads. Rarely, the other left ventricular wall or only the right ventricle is involved—the latter manifesting with right ventricular outflow tract obstruction.
Cardiac hypertrophy, in the absence of a secondary cause, is the quintessential clinical diagnostic criterion for HCM. However, cardiac hypertrophy is the common response of the heart to a variety of stress, including increased loading conditions, such as systemic arterial hypertension. HCM is diagnosed when cardiac hypertrophy is judged to be disproportionate to the increased load. HCM might also be present concomitantly with systemic arterial hypertension, rendering the clinical diagnosis challenging. Phenotypic features, including ASH, increased left ventricular ejection fraction, a small left ventricular cavity size, and the presence of LVOT obstruction, would suggest the diagnosis of HCM in those with hypertension.
The distinction between physiological hypertrophy observed in professional athletes and pathological hypertrophy of HCM also could be challenging. Again, characteristics described above, including the presence of a small left ventricular cavity size and LVOT obstruction, would indicate HCM, as athletes typically exhibit cardiac hypertrophy with an enlarged left ventricle. Other features, including pathological Q waves, conduction defects, reduced tissue Doppler velocities, and reduced strain, would favor the diagnosis of HCM.
Phenocopy conditions, such as glycogen storage diseases, lysosomal storage diseases, mitochondrial diseases, triplet repeat syndromes, and others, mimic HCM. The distinction is typically difficult, despite differences in the pathogenesis of HCM and its phenocopy conditions, such as Fabry disease. Typically, a very high or low QRS voltage and presence of a delta wave on an electrocardiography (ECG) in a male patient with severe cardiac hypertrophy, as well as involvement of other organs, would hint at the possibility of a phenocopy condition. Genetic testing could lead to differentiation of HCM and its phenocopy conditions, although the yield of genetic testing is not high.
The pathological hallmark of HCM is myocyte disarray, which is defined as loss of normal parallel alignment of cardiac myocytes because of haphazardly oriented deformed myocytes that are enlarged and have pleiotropic nuclei ( Fig. 23.6 ). Disarray typically involves more than 10% of the myocardium and is typically present in conjunctions with hypertrophy and fibrosis. Severe myocyte disarray has been associated with increased risk of SCD in patients with HCM.

Another important histological feature of HCM is interstitial fibrosis, which is associated with an increased risk of cardiac arrhythmias, heart failure, and SCD (see Fig. 23.6 ). Modern imaging techniques have enabled the detection and quantification of myocyte disarray and interstitial fibrosis without the need for an endomyocardial biopsy. Late gadolinium enhancement (LGE), detected and quantified by cardiac magnetic resonance (CMR) imaging, as a marker for extracellular volume fraction and interstitial fibrosis, is associated with the occurrence of cardiac arrhythmias and the risk of SCD in HCM. Likewise, collagen cleavage products are useful biomarkers for the presence of interstitial fibrosis in HCM. Other potential biomarkers for cardiac hypertrophy, cell death, and fibrosis, are cytokines, cardiac troponin T, and microRNAs.
Clinical Manifestations
The majority of patients with HCM are asymptomatic or minimally symptomatic, even though they typically exhibit reduced exercise tolerance. The common symptoms include palpitations, chest pain, and shortness of breath. The major clinical issues in patients with HCM include the management of cardiac arrhythmias and the risk of SCD; the management of heart failure, typically due to diastolic dysfunction; and the management of LVOT obstruction.
Chest Pain
Patients with HCM frequently experience chest pain, particularly the young individuals. It is commonly exercise induced, but otherwise does not follow the characteristics of angina pectoris of coronary origin. Chest pain presumably occurs because of a mismatch between the oxygen demand of hypertrophic myocardium and an inadequate supply partly because of increased intramural coronary vasculopathy, marked by thickening of arterial media, and a relatively reduced capillary density, leading to myocardial ischemia and even necrosis ( see Fig. 23.6 ). However, in older patients, concomitant coronary artery disease might be present. For those with no obstructive coronary artery disease, treatment with β-blockers and nondihydropyridine calcium channel blockers is effective in alleviating symptoms.
Heart Failure
Heart failure symptoms in patients with HCM are commonly due to diastolic dysfunction ( see also Chapter 11 ) with an elevated left ventricular end diastolic pressure but a normal or increased left ventricular ejection fraction. Therefore, it is heart failure with preserved ejection fraction (HFpEF). Although the left ventricular ejection fraction is preserved, myocardial function, both systolic and more markedly diastolic, is impaired, as detected by modern imaging techniques such as tissue Doppler imaging, 3-D speckle tracking echocardiography, and CMR ( Fig. 23.7 ). Consequently, diastolic dysfunction is the major cause of heart failure in patients with HCM, and is a major prognosticator. Symptom-wise, exercise intolerance and reduced cardiopulmonary capacity are the common early symptoms. Often, patients modify their lifestyle to cope with the symptoms. Therefore, detailed history taking is necessary to uncover the exercise intolerance. Exercise intolerance is often associated with findings of fluid retention such as peripheral edema. Exertional dyspnea is often progressive, and if untreated leads to the classic symptoms of fluid retention, such as orthopnea and paroxysmal nocturnal dyspnea, as well as peripheral edema. The symptoms are first responsive to medical treatment including the judicious use of diuretics. The use of diuretics is particularly concerning in those with an LVOT obstruction, as excess diuresis could induce hypovolemia, hypotension, and syncope.

The main risk factors for heart failure in patients with HCM include LVOT obstruction, severe cardiac hypertrophy, extensive interstitial fibrosis, and atrial fibrillation. LVOT in approximately one-third of the patients, and is a major contributor to elevated left ventricular end diastolic pressure and HFpEF. LVOT obstruction results from cardiac hypertrophy, particularly asymmetric septal hypertrophy, which narrows the outflow tract, and a small left ventricle cavity size, collectively leading to a Venturi effect and displacement of the anterior leaflet of the mitral valve toward septum during systole (systolic anterior motion) and obstruction of blood flow at the LVOT (see Fig. 23.6 ). Resting LVOT obstruction, defined a pressure gradient of >30 mm Hg, is present in approximately one-quarter of HCM patients, but is inducible with exercise in more than half of the patients. As initially described by Sir Russell Brock and Dr. Braunwald, the obstruction is dynamic and varies with changes in contractility, stroke volume, and afterload. It is an important contributor to the progression of cardiac hypertrophy and the risk of heart failure.
Atrial fibrillation is also common and found in about one-quarter of patients with HCM. A new onset of atrial fibrillation is not well tolerated and often leads to heart failure. Diastolic dysfunction infrequently progresses to refractory heart failure requiring heart transplantation. In addition, a small subset of patients with HCM, estimated to be about 5%, develop global systolic dysfunction with chamber dilatation and evolve into DCM, leading to heart failure with reduced ejection fraction.
Ventricular Arrhythmias and Sudden Cardiac Death
SCD is the often the first manifestation of HCM, particularly in the young, but is fortunately infrequent, with an incidence of 0.5% to 2% per year in the overall HCM population. Nonetheless, HCM is the most common cause of SCD in young competitive athletes, which is often tragic and occurs in the absence of prior symptoms. However, minor symptoms might be present, which often are not pursued. Syncope is a major risk factor for SCD in patients with HCM. It commonly occurs because of ventricular tachycardia and less commonly because of orthostatic hypotension resulting from hypovolemia, LVOT obstruction, and autonomic dysfunction. SCD in HCM is typically because of ventricular tachycardia/fibrillation. Therefore, symptoms of palpitations, presyncope, and syncope necessitate additional investigation for cardiac arrhythmias, typically by long periods of telemetric rhythm monitoring.
Sustained ventricular tachycardia and repetitive nonsustained ventricular tachycardia, the latter particularly when associated with lightheadedness or presyncope, are major risk factors for SCD and necessitate implantation of an ICD. A family history of SCD, reflective of the underpinning causal mutation and the genetic background, is also an important risk factor for SCD. However, given the presence of considerable variability in the phenotypic expression of HCM, additional findings would be needed, besides a family history of SCD, to implant an ICD. However, there is no uniform opinion on the indications for an ICD implantation. Severe cardiac hypertrophy and extensive interstitial fibrosis are also associated with an increased risk of SCD in HCM.
Supraventricular Arrhythmias
Atrial fibrillation/flutter and paroxysmal atrial tachycardia are the common forms of supraventricular tachycardias in HCM. The annual incidence of atrial fibrillation is about 3% per year. Overall, about one-quarter of patients with HCM experience atrial fibrillation, which is often not well tolerated and is associated with adverse clinical outcomes. The rapid heart rate, leading to a shortened diastolic phase, and the loss of atrial contraction in the presence of impaired left ventricular relaxation and compliance, lead to elevated left ventricular filling pressure and symptoms of heart failure. Likewise, reduced stroke volume could lead to hypotension and symptoms of lightheadedness, presyncope, and syncope. A preexcitation pattern on an electrocardiogram is detected in approximately 5% of patients with HCM, which might lead to AV nodal reciprocating arrhythmias. The preexcitation pattern is more common in patients with a phenocopy condition, such as glycogen storage diseases.
Cardioversion is the treatment of choice for symptomatic new onset atrial fibrillation, followed by medical therapy to prevent recurrence. Recurrent and paroxysmal atrial fibrillation are treated with antiarrhythmic drugs. Atrial fibrillation increases the risk of thromboembolism. The annual incidence of thromboembolism in HCM is estimated to be about 4%. Given the increased risk, stroke patients with atrial fibrillation require long-term anticoagulation.
Prognosis
Patients with HCM exhibit a spectrum of clinical course, ranging from SCD at a young age to a normal life expectancy. All things considered, HCM is a relatively benign disease, particularly in those without an LVOT obstruction who have a very low rate of complications. The presence of LVOT obstruction increases the risk of heart failure, and in its absence, only about 10% of patients experience heart failure symptoms over the course of 6.5 years. Overall, approximately two-thirds of patients with HCM do not have increased morbidity and experience a normal life expectancy.
As mentioned, HCM is a relatively benign disease despite the risk of SCD. The annual mortality of garden variety adult patients with HCM is estimated at less than 1%. This is also the case for patients with apical HCM, who exhibit a 15-year survival probability of approximately 95%.
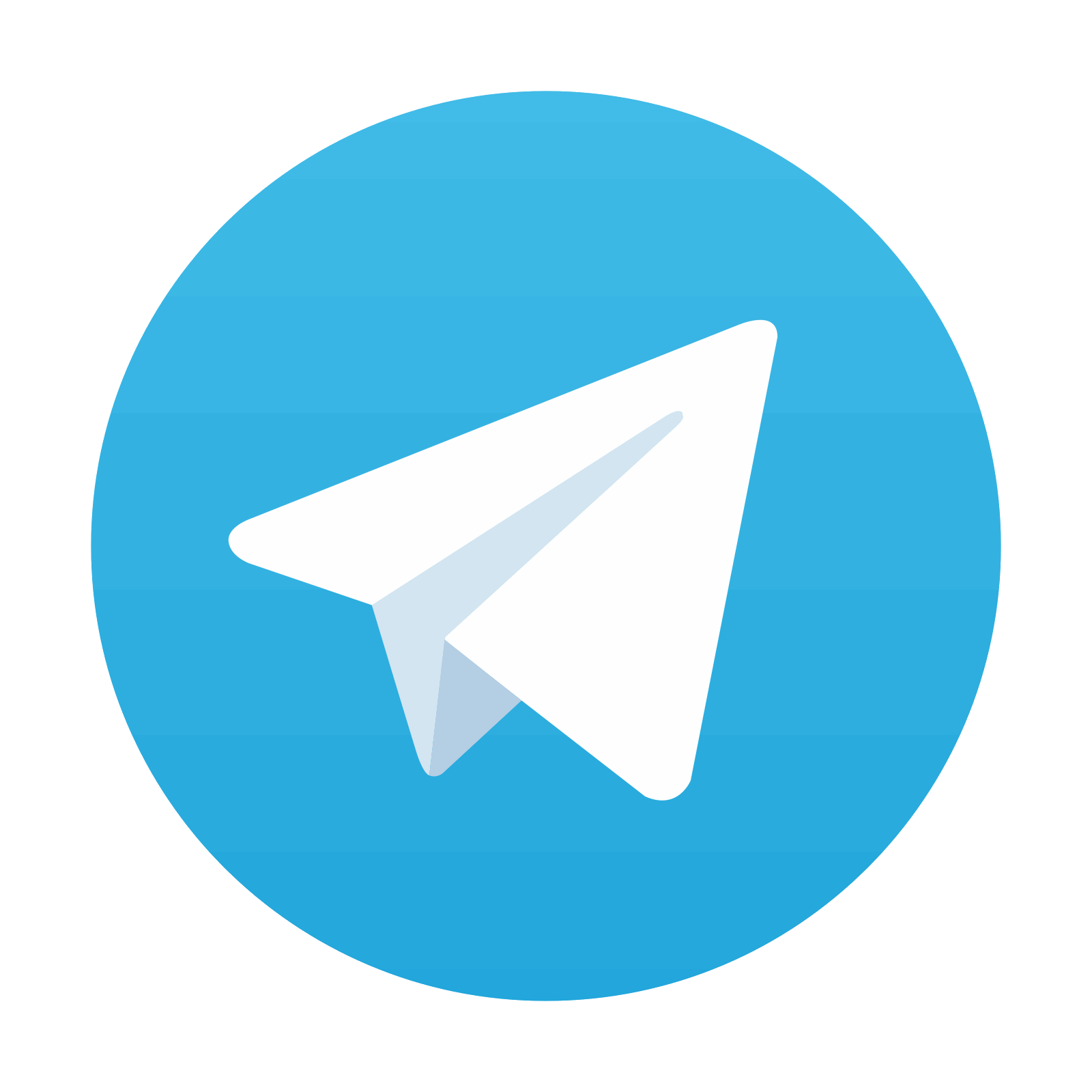
Stay updated, free articles. Join our Telegram channel

Full access? Get Clinical Tree
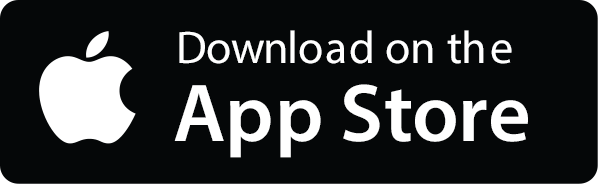
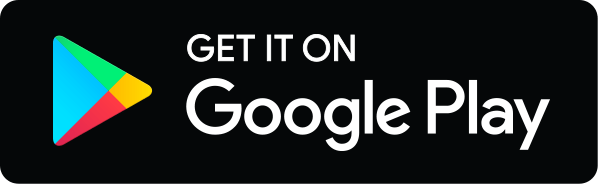