Outline
Clinical Presentations, 323
Findings That Indicate a Genetic Form of Cardiomyopathy, 323
Fabry Disease, 324
Danon Disease, 324
Familial Cardiac Amyloidosis, 325
Arrhythmogenic Right Ventricular Cardiomyopathy, 325
Left Ventricular Noncompaction Cardiomyopathy, 325
Genetic Causes of Cardiomyopathy, 327
Sarcomere Mutations, 327
Clinical Implications, 327
Cytoskeletal Mutations, 328
Clinical Implications, 328
Mutations Altering Calcium Handling, 328
Clinical Implications, 328
Nuclear Envelope Mutations, 329
Clinical Implications, 329
Ion Channel Mutations, 329
Clinical Implications, 330
Transcription and Splicing Factor Mutations, 330
Clinical Implications, 330
Desmosome Mutations, 330
Clinical Implications, 331
Mitochondrial Mutations, 331
Clinical Implications, 331
Genetic Testing, 331
The genetic contributions to heart failure are numerous and remarkably heterogeneous, extending from the monogenic forms of cardiomyopathy to more common polygenic diseases, such as hypertension, coronary atherosclerosis, and myocardial infarction. For monogenic diseases, the responsible mutations are rare but carry a very high pathogenic burden. Polygenic conditions typically include DNA variants that are common in healthy populations, imparting only a small pathogenic effect. For all of these conditions, both genetic factors and environmental stimuli likely work in synergy to cause cardiovascular disease. This chapter will focus on the monogenic disorders, such as familial dilated, hypertrophic, restrictive, and arrhythmogenic forms of cardiomyopathy, and their progression to heart failure.
In considering the genetic aspects of heart failure, one should contemplate not only the underlying cause of a familial form of cardiomyopathy, but also the pattern of familial segregation, or, more specifically, consider who else in the family is at risk of developing heart failure. One should also recognize syndromic features of disorders that include familial cardiomyopathy. Genetic testing is an important aspect of the evaluation for familial cardiomyopathy, and it should be preceded by genetic counseling by a trained counselor. From a research perspective, improved understanding of the genetic basis for familial forms of cardiomyopathy should help to develop better treatments.
The following clinical scenario highlights many of these aspects. A 22-year-old man was referred for genetic evaluation due to his family history of dilated cardiomyopathy (DCM) and sudden cardiac death. He was healthy as a child and young adult, training for a triathlon without cardiac limitations or symptoms. His family history is shown in the pedigree in Fig. 24.1 . His paternal grandfather died suddenly in his early 50s. No autopsy was performed, and his death was attributed to a “heart attack.” Later, a paternal uncle had sudden cardiac death in his early 40s. This time an autopsy was performed, showing nonspecific features of DCM, without significant coronary atherosclerosis or inflammatory heart disease. Echocardiograms were performed for the surviving three siblings of this deceased individual, and two had asymptomatic DCM. The youngest in this generation progressed to end-stage heart failure, and he underwent cardiac transplantation. The father of the proband received an implanted cardioverter-defibrillator (ICD), and had multiple appropriate shocks for ventricular tachycardia despite his ejection fraction (EF) of 45%. Three years ago, the proband’s echocardiogram showed left ventricular (LV) diameter 5.4 cm, EF 55%, and now his echocardiogram shows his LV diameter is 5.7 cm, and his EF is 50%. His electrocardiogram (ECG) is normal.

Several questions arise at this point: (1) Are the LV enlargement and mildly reduced EF due to his exercise or to early features of a genetic cardiomyopathy? (2) What is his risk of sudden cardiac death? (3) Should he continue to exercise? (4) Which asymptomatic family members carry the same genetic predisposition to DCM and sudden cardiac death? Recognition of a clear genetic cause will help to answer these questions. The male-to-male transmission excludes X-linked disorders, and the pattern is most consistent with autosomal dominant inheritance. This means that each child of an affected individual carries a 50% chance of inheriting the genetic predisposition. Notably, a mutation in LMNA encoding the nuclear envelope protein lamin A/C, could cause this condition. LMNA mutations are associated with high rates of sudden death and systemic manifestations. Such a mutation may influence decisions about ICD placement and arrhythmia risk in the proband and family members.
After genetic counseling, clinical genetic testing was performed in the proband’s father using a panel of genes in which mutations cause DCM. His has a heterozygous substitution of a highly conserved arginine with a histidine at codon 636 in exon 9 of RBM20 , encoding RNA Binding Motif Protein 20 ( Fig. 24.2 ). This mutation was also present in the proband’s affected paternal uncle, and absent in his paternal grandmother (probably present in his affected paternal grandfather). It has been published as a pathogenic mutation. His targeted genetic testing shows that he also has this mutation. As such, his mild LV dilation and dysfunction are likely early features of genetic DCM. His sister and each of his paternal first cousins in Fig. 24.1 also have this mutation, establishing their predisposition to DCM and sudden cardiac death.

Clinical Presentations
People with familial forms of cardiomyopathy typically do not have overt symptoms until they have advanced dysfunction with very low cardiac output fairly late in the course of their disease. When cardiac function abruptly declines, as with acute ischemic or inflammatory injury to the heart, the sudden elevation of ventricular filling pressures usually causes prominent symptoms. With gradual loss of ventricular systolic function, physiological compensation due to neurohormonal activation causes progressive dilation of the heart and initial preservation of the stroke volume. Yet this same process of neurohormonal activation eventually becomes pathological, contributing to greater LV dilation and loss of systolic function.
The prevalence of familial DCM is often underestimated. Though sometimes considered rare, careful assessment of the first-degree relatives of individuals with otherwise unexplained DCM for the same condition has repeatedly shown 20% to 35% with familial DCM. One study considered family members with LV enlargement greater than 112% of the size predicted on the basis of body surface area and normal systolic function as affected, and they reported a prevalence of familial DCM of 48% in a cohort that was previously considered to be idiopathic. With this in mind, the American College of Cardiology/American Heart Association/American Society of Echocardiography (ACC/AHA/ASE) guidelines for the use of echocardiography provide a Class I recommendation for evaluation of first-degree relatives (parents, siblings, and children) of people with unexplained DCM.
The genetic forms of cardiomyopathy are typically categorized based on the appearance of the heart. Because of pioneering research identifying its genetic basis, hypertrophic cardiomyopathy (HCM) is now usually well recognized as a familial condition ( see also Chapter 23 ). In contrast, nonischemic DCM can be caused by numerous other factors ( see also Chapter 20 ). Accordingly, familial DCM is less well recognized when it occurs. Incomplete pedigrees, age-dependent onset of symptoms, de novo mutations, recessive inheritance, and low penetrance are all factors contributing to failed recognition of familial forms of cardiomyopathy. The phrase “heart attack” is a commonly used lay term that infers coronary thrombosis or occlusion, but it may otherwise refer to arrhythmic sudden death in the context of familial cardiomyopathy. Thus when discussing family history with individuals with idiopathic cardiomyopathy, it is important to clarify whether “heart attack” or sudden death occurred in the context of known coronary disease or extensive coronary atherosclerotic risk factors. If an autopsy was performed, it should be able to discern ischemic and nonischemic forms of heart failure. Familial cardiomyopathy also includes restrictive cardiomyopathy (RCM), which may overlap with DCM or HCM in some families and may be missed without careful imaging ( see also Chapter 21 ).
The most easily recognized pattern of inheritance is autosomal dominant, but autosomal recessive, X-linked, and matrilinear (mitochondrial) patterns also occur. In X-linked forms of cardiomyopathy, female carriers may manifest the condition (X-linked dominant), though typically later and less severely than affected men. A familial trait like cardiomyopathy does not need to affect a large number of people in the family. In fact, recessive, X-linked, or de novo dominant forms of cardiomyopathy can appear without clear antecedent family history to alert healthcare providers to its underlying basis. Simply the presence of a single similarly affected family member allows for the diagnosis of familial cardiomyopathy. A family history of early and unexplained sudden death, typically below the age of 35 years, may also be considered as a criterion for the diagnosis of familial cardiomyopathy. In families with a single affected individual, the term “genetic cardiomyopathy” may be preferable to familial cardiomyopathy.
Findings that Indicate a Genetic Form of Cardiomyopathy
The main diagnostic tool in establishing the diagnosis of familial cardiomyopathy is ascertainment of a comprehensive family history. American College of Cardiology Foundation (ACCF)/AHA guidelines recommend review of at least three generations of family history. This is best determined with a pedigree, in which each individual is represented by a circle (female) or square (male), with designation of phenotypic features by shading part or all of the circle or square. Rather than open-ended questions inquiring whether a family history of cardiomyopathy exists, health details for each person in the family should be reviewed, with particular attention to cardiovascular manifestations.
Although assessment of the family history and phenotypic characterization of family members who are at risk of inheriting the same genetic disorder is the best way to recognize inherited forms of cardiomyopathy, some additional features may help to make this diagnosis on initial presentation ( Table 24.1 ). Because the heart and skeletal muscles both contain several of the same protein components, an individual with concomitant heart failure and skeletal myopathy usually has a monogenic condition to explain both findings, with rare systemic inflammatory exceptions. The manifestations of skeletal myopathy may be subtle, consisting of asymptomatic elevation of the serum creatine kinase level or abnormalities in muscle bulk (pseudohypertrophy or muscle hypotrophy). There is poor correlation between the severity of cardiac dysfunction and skeletal muscle weakness.
Organ | Manifestation | Consider |
---|---|---|
HEENT | Dysmorphic appearance | Chromosomal abnormality, LMNA mutation |
Blindness or unexplained loss of vision | Mitochondrial disorder | |
Deafness or premature presbycusis | Mitochondrial disorder, EYA4 mutation | |
Unexplained corneal keratopathy | Fabry disease | |
Retinopathy | Danon disease | |
Adipose | Lipodystrophy | LMNA mutation |
Skin | Angiokeratomas | Fabry disease |
Palmoplantar keratodermas | Naxos, Carvajal, ARVC | |
Hypohidrosis | Fabry disease | |
Central nervous system | Developmental delay | Chromosomal disorder |
Danon disease | ||
Mitochondrial disorder | ||
Presenilin mutation | ||
Peripheral nervous system | Peripheral neuropathy | Fabry disease |
TTR amyloidosis | ||
Skeletal muscle | Reduced strength, tone, or bulk | Cardio-skeletal myopathy |
Pseudohypertrophy | Cardio-skeletal myopathy | |
Unexplained elevation of serum creatine kinase | Cardio-skeletal myopathy |
Mitochondrial disorders typically include cardiomyopathy, in addition to skeletal myopathy, blindness or loss of vision, early hearing loss, developmental delay, seizures, and/or short stature. Elevation of the resting lactic acid level in blood without hypoperfusion also suggests a mitochondrial disorder, and should prompt further evaluation for systemic manifestations. There are several forms of glycogen- and lysosomal-storage diseases with cardiac manifestations. These are usually recessive or X-linked disorders with loss-of-function mutations in enzymes, and they result in excessive production and deposition of intermediate metabolites.
Fabry Disease
Mutations in GLA , encoding alpha-galactosidase A, cause X-linked Fabry disease (or Anderson-Fabry disease). Johannes Fabry in Germany and William Anderson in England simultaneously first reported this condition in 1898. The constellation of HCM, renal dysfunction, peripheral neuropathy, hypohydrosis, and angiokeratomas of the skin should raise concern for Fabry disease, but it can also occur with isolated cardiac involvement. Corneal opacity (keratopathy) also occurs in Fabry disease, and it may be indistinguishable from amiodarone-induced corneal disease.
The age of onset for Fabry disease depends on gender and the type of mutation. Males with complete absence of alpha-galactosidase A enzyme activity typically have their initial symptoms during late childhood. Males who have partial enzyme activity often present later in life with HCM, and often without systemic features. Clinical manifestations in women with a heterozygous GLA mutation range from asymptomatic to the full spectrum seen in affected men. Since the responsible gene is on the X chromosome, male-to-male transmission of HCM in a family makes Fabry-associated HCM unlikely, unless Klinefelter syndrome (chromosome XXY) is present.
A clinical suspicion of Fabry disease can be confirmed by measurement of serum alpha-galactosidase A enzyme activity or by GLA genetic testing. Because women may have skewed inactivation of the X chromosome in different tissues, their serum alpha-galactosidase A enzyme activity may be normal in blood but deficient in the heart. Accordingly, blood enzyme activity is less reliable in women than in men, who have only a single copy of this gene. In one study, 90 probands with HCM underwent genetic analysis; 31/90 (34%) had sarcomere mutations, and 3/90 (3%) had GLA mutations. None of these individuals had systemic features of Fabry disease. The inclusion of this gene in large panels for genetic evaluation of cardiomyopathies should help to increase recognition of Fabry disease, and particularly with isolated cardiac involvement.
Perhaps because of its position on the X chromosome and correlation with serum enzyme activity analysis in men, careful studies have identified less obvious mutations occurring deep in the introns of GLA. Although complete loss of function of the enzyme usually causes the full spectrum of Fabry disease, partial loss may have cardiac- or renal-limited manifestations. For instance, the IVS4+919G>A was found to cause greater than 90% decrease in enzyme activity with later onset, cardiac-limited Fabry due to creation of abnormally spliced mRNA. Although it was previously considered to be a very rare condition, newborn screening for Fabry has expanded in Asia, demonstrating that 0.13% of infants in Taiwan carry this mutation, presumably half of whom are male.
The availability of replacement enzyme infusions for Fabry disease should lead to consideration of this condition in anyone with unexplained HCM. Most clinical panels for genetic testing for HCM include GLA . Alternatively, if an X-linked pattern of inheritance is not excluded with familial segregation of HCM, then a serum alpha-galactosidase A activity level may be useful for screening, particularly in men as compared to women with HCM. One study showed that treatment of affected individuals with recombinant human alpha-galactosidase A slowed progression to a composite endpoint, including cardiac, renal, and cerebrovascular complications or death, when compared to placebo. The development of recombinant alpha-galactosidase A for the treatment of Fabry disease highlights the possibility of similar targeted therapy for related enzyme deficiencies.
Danon Disease
Danon and colleagues reported another X-linked glycogen storage disease with cardiomyopathy. Phenotypic manifestations include cardiac hypertrophy with loss of systolic function, skeletal myopathy, developmental delay, and retinopathy. Loss-of-function mutations in LAMP2 , encoding a lysosome-associated membrane protein, cause this condition, although enzyme replacement therapy is not currently available.
One report highlighted the rapid progression and early mortality in a very small cohort of children (6 males and 1 female, age 7–17 years) with LAMP2 mutations. Four died from heart failure, one died from ventricular fibrillation refractory to defibrillator shocks, and one underwent cardiac transplantation with mean age of 21 years for these endpoints. In a much larger cohort of 82 individuals with Danon disease from 36 separate families, in addition to 63 previously reported cases (145 people with this condition), another group more comprehensively characterized the natural history of this rare disorder. In this report, the average ages of first symptom, cardiac transplantation, and death were 12.1, 17.9, and 19.0 years in men, and 27.9, 33.7, and 34.6 years in women, respectively. Cardiac transplantation appropriately addresses heart failure when it progresses to advanced stages, but progressive disease in other organ systems will occur in that context.
Familial Cardiac Amyloidosis
Cardiac amyloidosis may be suspected when there is discordance between imaging studies showing concentric ventricular hypertrophy and electrocardiograms demonstrating low ECG voltage ( see also Chapter 22 ). Mutations in TTR , encoding transthyretin, are the most common cause of inherited cardiac amyloidosis. Transthyretin is a carrier protein in the blood, binding to thyroid hormone and retinol binding protein. Mutations occur throughout the encoded protein, after cleavage of a 20-amino acid N-terminal signal peptide. In contrast with Human Genome Organisation (HUGO) nomenclature rules, the traditional numbering of mutations in this gene corresponds to the mature protein rather than the pro-protein. Accordingly, a clinical laboratory report may indicate that a mutation is p.Val50Met, then designating it by its more commonly recognized enumeration, Val30Met. Approximately 3.4% of African Americans carry one mutation (Val122Ile or p.Val142Ile by HUGO nomenclature) in heterozygosity or homozygosity, and its presence is associated with a higher frequency of heart failure after age 70 years. The high prevalence of this allele among individuals with black African ancestry and the concomitantly high prevalence of heart failure with preserved EF (HFpEF) suggest that many older patients with black African ancestry and HFpEF may have unrecognized transthyretin cardiac amyloidosis. In one intriguing study looking at 650 self-identified black participants in the BEST (Beta-Blocker Evaluation in Survival) trial with NYHA (New York Heart Association) class III-IV heart failure, the prevalence of the Val122Ile mutation for those less than age 60 was 3.5%, whereas for those 60 years and older, the prevalence of this TTR mutation was 10%. For other less common mutations in TTR, the likelihood of developing cardiac amyloid and the age at which it may develop should be tailored to the specific mutation.
Although far less common, other familial forms of amyloid can occur due to mutations in genes encoding apolipoprotein A-I (APOA1) , apolipoprotein A-II (APOA2) , gelsolin (GSN) , fibrinogen A (FGA) , and lysozyme (LYZ) . Although these forms of familial amyloidosis do not typically involve the heart, systemic deposition of amyloid occurs in later stages and sometimes leads to cardiac dysfunction, which is similar to other forms of cardiac amyloidosis.
Arrhythmogenic Right Ventricular Cardiomyopathy
Arrhythmogenic right ventricular cardiomyopathy (ARVC) is genetic disorder characterized by right ventricular fibrofatty scar and prominent ventricular arrhythmias ( see also Chapter 21 ). Because of the uncommon presence of fat in the mid-wall of the RV, this condition was initially called arrhythmogenic right ventricular dysplasia (ARVD). However, “dysplasia” typically refers to malignancy or abnormal proliferation, which is not present in patients with this condition. Accordingly, the preferred term evolved to ARVC or ARVD/C. Similar pathological changes and arrhythmia also occur in the left ventricle, leading to the eponym arrhythmogenic left ventricular cardiomyopathy (ALVC) and the broader category for both conditions—arrhythmogenic cardiomyopathy (ACM). Although the term ACM encompasses both conditions, detailed criteria have been used for the diagnosis of ARVC, and emerging recommendations classify ARVC as a subtype of ACM.
In one US study of 100 affected individuals, the median age at presentation was 26 years, with the initial diagnosis occurring postmortem in 31%. The original description of this condition and subsequent diagnostic criteria focused on cardiomyopathies that predominantly (or exclusively) involved the RV. Now, overlap with LV involvement is expanding the diagnosis to include all forms of ACM. Naxos disease and Carvajal syndrome are two similar recessive disorders that also involve palmoplantar keratoderma and wooly hair. Noncardiac involvement in ARVC is quite rare, though subtle keratoderma or wooly hair may be present if carefully investigated.
The most frequent manifestation of ARVC is ventricular tachyarrhythmia, and once the condition is recognized with institution of appropriate antiarrhythmic therapies, the next priority for management becomes recognition and treatment of heart failure. One study identified at least one sign or symptom of heart failure in nearly half of all patients with ARVC. Many individuals with ARVC have a long history of prominent aerobic exertion. In retrospective investigation, the amount of exercise, particularly after the diagnosis of ACM, directly correlates with the likelihood of further arrhythmia and development of heart failure.
Approximately 50% of individuals with ARVC have a mutation in a component of the cardiac desmosome. Immunohistochemical analysis for plakoglobin is consistently reduced and mislocalized in ARVC hearts, in contrast with HCM, DCM, ischemic cardiomyopathy, and control hearts. Notably, cardiac sarcoid is commonly on the differential diagnosis for ARVC, and reduced plakoglobin immunoreactivity is quite similar in sarcoid as in ARVC. Extending beyond the desmosome, mutations contributing to ARVC also occur in several other genes ( Table 24.2 ).
Encoded Protein’s Cellular Location/Function | Gene | DCM | HCM | RCM | ARVC |
---|---|---|---|---|---|
Sarcomere/myofilament or Z-disc | ACTC | + | + | − | − |
ACTN2 | + | + | − | − | |
ANKRD1 | + | + | − | − | |
CSRP3 | + | + | + | − | |
MYBPC3 | + | + | − | − | |
MYH6 | + | + | − | − | |
MYH7 | + | + | + | − | |
MYL2 | − | + | − | − | |
MYL3 | − | + | − | − | |
MYLK2 | − | + | − | − | |
MYOZ2 | + | + | − | − | |
MYPN | + | + | + | − | |
NEBL | + | − | − | − | |
NEXN | + | + | − | − | |
TCAP | + | + | − | − | |
TNNC1 | + | + | − | − | |
TNNT2 | + | + | + | − | |
TNNI3 | + | + | + | − | |
TPM1 | + | + | − | − | |
TTN | + | + | − | + | |
Cytoskeleton | DES | + | − | + | + |
DMD | + | − | − | − | |
DTNA | + | − | − | − | |
FKTN | + | − | − | − | |
FLNC | + | + | + | + | |
LDB3 | + | + | − | − | |
OBSCN | + | + | − | − | |
PDLIM3 | + | − | − | − | |
SGCD | + | − | − | − | |
VCL | + | + | − | − | |
Calcium regulation | CAV3 | + | − | − | − |
CALR3 | − | + | − | − | |
JPH2 | − | + | − | − | |
PLN | + | − | − | + | |
PSEN1 | + | − | − | − | |
PSEN2 | + | − | − | − | |
RYR2 | − | − | − | + | |
Nuclear envelope | EMD | + | − | − | − |
ILK | + | − | − | − | |
LAMA4 | + | − | − | − | |
LAP2 | + | − | − | − | |
LMNA | + | + | + | + | |
TMEM43 | + | − | − | + | |
Ion channel | ABCC9 | + | − | − | − |
SCN5A | + | − | − | + | |
Transcription factor or regulator of transcription | EYA4 | + | − | − | − |
GATA4 | + | − | − | − | |
GATAD1 | + | − | − | − | |
NKX2-5 | + | − | − | − | |
PRDM16 | − | − | − | − | |
RAF1 | + | + | − | − | |
RBM20 | + | − | − | − | |
Desmosome and associated cell junction | CDH2 | − | − | − | + |
CTNNA3 | − | − | − | + | |
DSC2 | − | − | − | + | |
DSG2 | + | − | − | + | |
DSP | + | − | − | + | |
JUP | − | − | − | + | |
PKP2 | − | − | − | + | |
Mitochondria | ANT1 | + | + | − | − |
FKRP | + | − | − | − | |
FXN | − | + | + | − | |
ND1 | + | + | − | − | |
tRNA | + | + | + | − | |
TAZ | − | + | − | − | |
Storage disease | GAA | − | + | − | − |
GLA | − | + | − | − | |
LAMP2 | − | + | − | − | |
PRKAG2 | − | + | − | − | |
TTR | − | + | + | − | |
Chaperone | BAG3 | + | − | + | − |
CRYAB | + | − | + | − |
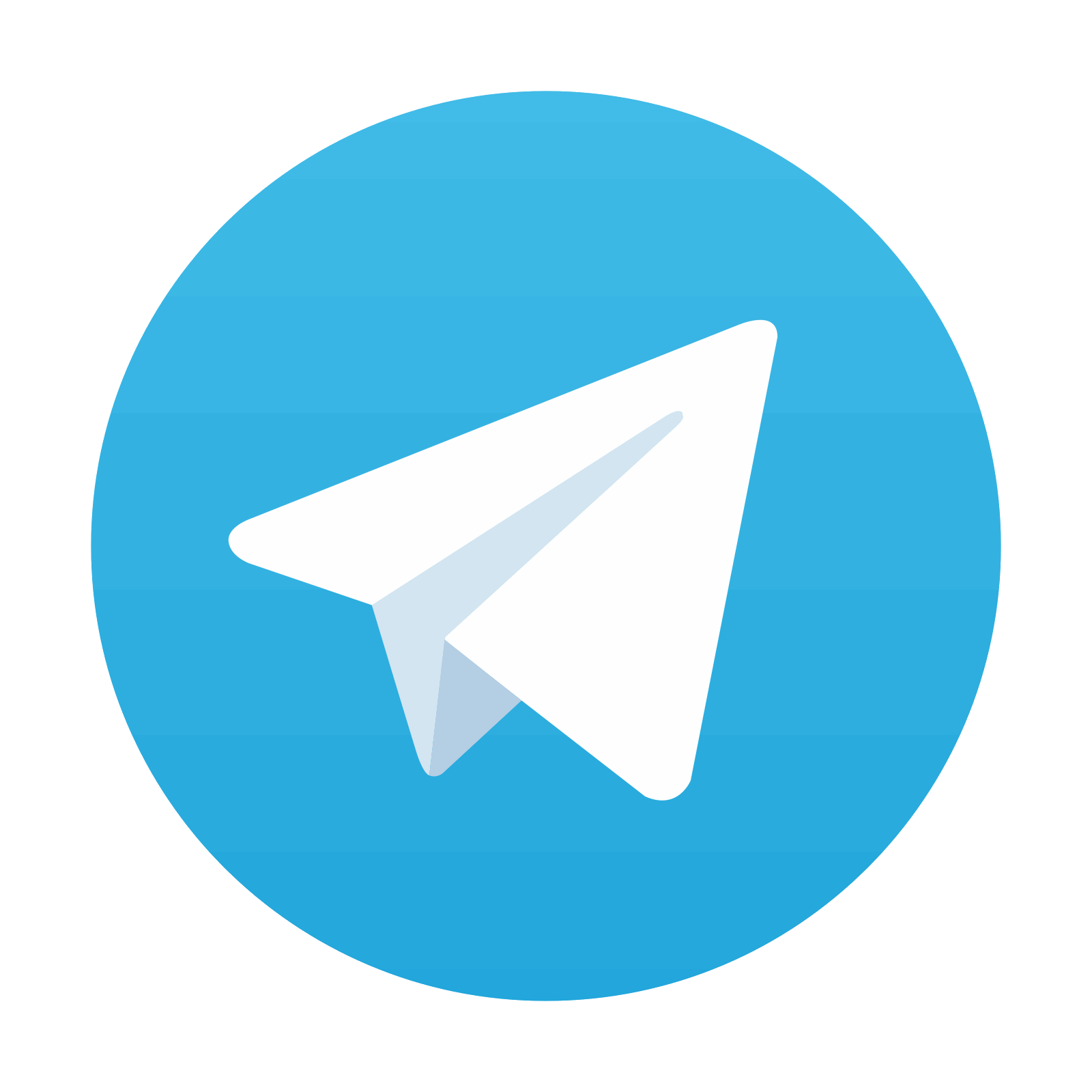
Stay updated, free articles. Join our Telegram channel

Full access? Get Clinical Tree
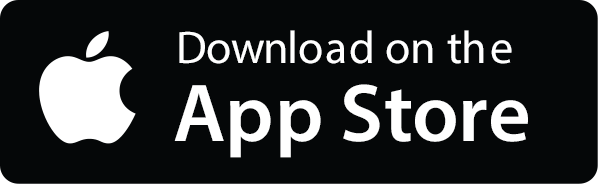
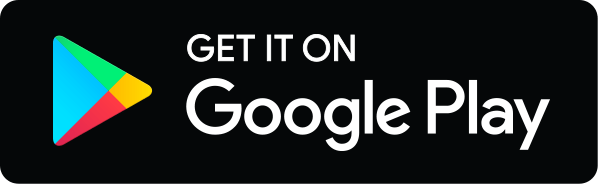
