10
Health effects of environmental exposures while in automobiles
10.1 Environmental exposures in automobiles
Cars and trucks are leading producers of air pollution worldwide. The number of cars in the world is estimated at 625 million and has been steadily increasing. The US Federal Highway Administration statistics estimate that 3.7 million cars have been added to roads each year since 1960, which is a 212% increase, putting the US total over 250 million. The time that people spend in cars is also increasing, with recent studies showing that most Americans spend an average of 90 minutes a day in cars. There are many occupations that require many hours in a vehicle, including truck drivers, bus drivers and police officers. Most people are unaware of the potential exposure to environmental pollution while they are driving and may even feel protected from the outside environment while inside a car. In the last 20 years, researchers have begun looking at the indoor environment of cars as a significant source of pollution exposure. Many studies have shown that driving in cars provides the highest level of exposure to particulate matter, volatile organic compounds, nitrogen compounds and carbon monoxide for most people. Several studies have shown that walking or biking in heavy traffic provides less exposure than driving a car in the same conditions. This exposure can adversely impact health and especially cause problems with the respiratory tract. Other exposures unique to the indoor environment of cars include smoking while driving, chemical out-gassing of volatile organic compounds from new cars and air bag deployment, which can all affect respiratory health.
10.2 Air pollution exposure while driving in cars
The pollution inside cars is a mix of gasoline and diesel exhaust and contains multiple noxious chemical substances in gaseous, aerosol and particulate form. Exposure is highest in heavy traffic, when driving behind vehicles that generate more exhaust, and in highly congested urban areas. Unfortunately, a car’s exterior and ventilation system do not protect passengers from exposure.
Particulate matter (PM) is probably the most dangerous component of car exhaust that drivers and passengers are exposed to. PM is made up of liquids and solids suspended in the air and can range in size from 10 μm, identified as ‘coarse’ or PM10, to less than 2.5 μm, identified as ‘fine’ or PM2.5. PM is often the product of incomplete combustion and includes smoke, dust and soot. PM is breathed into the nasal, sinus and bronchial passages and can accumulate and calcify. Very fine PM can penetrate to the alveoli and can even be absorbed into the bloodstream. PM acts locally in the nose, throat and lungs as an irritant causing nasal congestion, sinusitis, throat irritation, coughing, wheezing, shortness of breath and chest discomfort.
Several studies have shown that increased exposure to PM10 causes exacerbation of existing respiratory conditions, including asthma. A study done at Kaiser Permanente in Southern California showed that for every 10 μg/m3 increase in PM exposure, hospital admissions rose by 7% for patients with respiratory disease, 3.5% for patients with acute respiratory illnesses and 3% for patients with cardiovascular disease. A study by the California Environmental Protection Agency demonstrated an association of a 2.5% increase in emergency room visits and a 1% increase in mortality for people with pneumonia with every 10 μg/m3 increase in PM exposure level. The ‘Six Cities Study’ by the Harvard School of Public Health found that test subjects exposed to higher PM concentrations were 26% more likely to die prematurely than subjects exposed to lower concentrations.
Air conditioning systems can remove between 40 and 75% of the largest PM, but remove only 2-15% of the dangerous particles less than 1 μm in diameter. Commercial air filters can decrease coarse PM by 90%, but only reduce the fine particles by 5%. A study from the University of Southern California and the California Air Resources Board collected data in Los Angeles in 2003 and determined that drivers were receiving 35-45% of diesel and ultrafine particle exposure during their commute (average 1.5 hours). Diesel trucks and hard accelerations were the main sources of this pollution.
Volatile organic compounds (VOCs) are another significant component of air pollution emitted by cars. VOCs are produced by the combustion or evaporation of solvents, paints, glues and fossil fuels. VOCs are components of chemical reactions that create ozone/smog. The EPA has designated many VOCs hazardous air pollutants. Gasoline and diesel exhaust contains significant concentrations of dozens of VOCs. The most significant are benzene, 1,3-butadiene, xylenes, ethylbenzene, toluene and formaldehyde. Benzene and 1,3 butadiene are known carcinogens and formaldehyde is a suspected carcinogen. Inhaled benzene has been linked to leukemia and blood disorders in many studies, children being more susceptible. The World Health Organization has designated the acceptable level of benzene to be zero. There are no current links to the development of cancer secondary to exposure to benzene in vehicles, but it is felt that it contributes to the overall carcinogenic exposure. Short-term exposure to benzene may result in drowsiness, dizziness or headaches. Toluene acts on the central nervous system and can cause short-term fatigue, sleepiness, headaches and nausea. Long-term exposure to inhaled benzene can decrease white blood cell numbers and immunoglobulin levels, causing susceptibility to infections, including influenza and the common cold.
A 1991 study from the Harvard School of Public Health compared in-car VOC exposure, exposure on the roadside and exposure at a station away from the roadside. It found that exposures to the most significant VOCs (listed above) were the same in cars and in the air next to the car, but significantly higher than away from the roadside. Having the windows rolled up did not make any difference, although having the air conditioning on had a small impact on decreasing exposure. Another Harvard study found that exposure in cars was higher when compared with the exposure on the subway, while bicycling or walking. It also found that subjects’ commutes (average time 76 minutes) accounted for 21% of their benzene exposure.
Carbon monoxide (CO) is a gas generated from incomplete combustion of hydrocarbons, such as gasoline and diesel fuel. Cars produce 60% of the CO in the USA. In high-density urban areas cars and trucks are the source of 95% of CO production. CO production is increased when engines are cold vs warm or if they are not properly maintained. CO rapidly dissipates in the air and is only detected in enclosed spaces or areas of very high traffic volume. CO is a poisonous gas that is odorless, colorless and tasteless. CO strongly binds to hemoglobin, thus reducing oxygen binding to hemoglobin and oxygen delivery to tissues. Symptoms of headaches, dizziness, disorientation, nausea and fatigue are common at low levels and are often mistaken for the flu. High-level exposure can result in death. Patients with underlying respiratory disease are at risk from CO exposure if they have an increased oxygen demand. This concept holds true for people with cardiac disease, children and the elderly.
Many studies have shown that elevated CO levels are noted in cars while driving as compared with the ambient environment, even in heavy traffic areas. The California Air Resources Board conducted an extensive study of in-car CO exposure comparing different driving conditions, i.e. rush hour vs non-rush hour, freeway vs arterial and rural roads, in Los Angeles and Sacramento. The highest exposures were during rush hour on freeways in Los Angeles while the test vehicle was driving behind an out-of-tune truck or an older model sedan. Similar studies have been replicated internationally, specifically in Paris, the UK and Mexico City, which boasts the highest commuter CO exposure. Higher levels in general were found during evening commutes. In a Washington, DC study, this was felt to be secondary to many commuters receiving increased exposure when starting their cars in enclosed parking lots.
Nitrogen oxides are also produced during combustion of fuel and contribute to ground-level ozone, acid rain and particulate matter. Nitrogen dioxide is the best studied nitrogen oxide species. Direct exposure to nitrogen oxide causes mucosal irritation of the eyes, nose, throat and lungs and can exacerbate respiratory conditions such as asthma and chronic obstructive pulmonary disease (COPD) secondary to penetration of fine particulate matter. Exposure to nitrous oxide compounds is also associated with decreased ability of the lungs to combat bacteria and viruses, leading to lung infections. Many studies show that nitrogen dioxide exposure is higher inside cars as compared with ambient or roadside monitoring.
Ozone is not emitted by cars, but is a product of nitrogen oxide and VOCs reacting with sunlight. Relatively few studies have been done with regards to in-car exposure to ozone, but most show that ozone levels are lower in cars vs the ambient environment.
There is no question that cigarette smoke exposure is harmful to smokers and those around them. This is backed up by the most recent US Surgeon General’s Report from 2006. Components of tobacco smoke can be readily measured in the air where someone is smoking, including small particles that can penetrate into the lung, carbon monoxide, nicotine and benzene. The concentrations of the smoke components depend upon the number of smokers and how much they are smoking, the size of the space where the smoking is taking place, the rate of exchange of air in the smokers’ environment with outdoor air, and the presence and efficiency of air cleaning devices. The exposure of nonsmokers depends on the proximity to smokers while smoking. Obviously, smoking in an enclosed space such as a car intensifies smoke exposure. For the smoker, the inherent risks of lung and cardiovascular disease are increased by the extra exposure to smoke confined in a vehicle.
Mainstream smoke is the smoke that is inhaled through a burning cigarette/cigar by the smoker. Exhaled mainstream smoke is exhaled by the smoker and sidestream smoke is produced by the smoldering cigarette, both of which make up environmental tobacco exposure (ETS) or second-hand smoke (SHS). Recent studies have shown that SHS exposure while driving in cars is very high. Passengers in the back seat, who are often children, have higher exposure as the smoke tends to circulate and stay there. A study performed by the Harvard School of Public Health during 2005-2006 to evaluate SHS exposure and air quality in cars found unsafe levels of respired particulate matter and carbon monoxide for children. In 1992 the EPA classified SHS as a Class A carcinogen, characterized by strong epidemiologic evidence that it causes cancer.
In children, SHS increases the risk of respiratory diseases including lower respiratory infections such as bronchitis and pneumonia, asthma and decreases overall lung function. There is also a link to the long-term risk of lung cancer that increases in a dose-response relationship. In a population-based, case-control study, household exposure to 25 or more smoker-years during childhood and adolescence doubled the risk of lung cancer, whereas exposure to fewer than 25 smoker-years did not increase the risk. An estimated 17% of lung cancer in nonsmokers is attributable to high levels of environmental smoke exposure during childhood and adolescence. There is a growing body of evidence that SHS exposure increases the risk of a child developing cardiovascular disease in adulthood, but requires further study.
Adults exposed to SHS with underlying respiratory disease are more prone to exacerbations. There is evidence in adults as well, that the risk for lung cancer is increased with SHS exposure. The 2006 Surgeon General’s report included a meta-analysis including 52 studies, which showed that the relative risk of lung cancer among male and female nonsmokers who were ever exposed to SHS from a spouse was 1.21 (95% CI 1.13-1.30). The same report contained a meta-analysis of 25 studies of lung cancer and exposure to SHS in the workplace, which estimated a pooled relative risk of 1.22 (95% CI 1.13-1.33). The risk of cardiovascular disease is also increased by SHS exposure.
Some patients may be sensitive to the phenomenon of ‘out-gassing’ from new cars, which is the release of VOCs from plastics, glues and solvents from the interior of cars. This is the ‘new car smell’. In several studies 40-60 VOCs were identified. The most significant were ethylbenzene, benzene, acetone, cyclohexanone, n-hexane, styrene, toluene, xylene and undecane. The total VOCs were twice as high as ambient VOCs levels. The level of VOCs decreases significantly with time and one study showed a 90% reduction in by 3 weeks, whereas another study showed a decrease of 60% by one month after manufacturing. If patients are sensitive to out-gassing, they will probably complain of headaches, fatigue, drowsiness and eye, nose and throat irritation. They should limit their exposure to new cars, but with time should be able to tolerate driving or riding in the car that caused problems.
Airbag deployment and/or airbag rupture rarely cause serious lung injury, but there are case reports in the literature of reactive airway dysfunction syndrome (RADS) and asthmatic reactions related to sodium azide inhalation. Sodium azide reacts with potassium nitrite to produce a blast of nitrogen gas, which inflates the airbag. The gas then dissipates through tiny holes in the nylon fabric, deflating the bag. Sodium hydroxide and sodium carbonate are by-products of sodium azide combustion and released into the car. Sodium azide becomes hydroizoic acid, a volatile irritant, when mixed with water. RADS is characterized as chronic asthma-like symptoms developing within 24 h of exposure to an irritant. Patients often complain of cough, dyspnea and wheezing. A study by Gross et al. showed that asthma attacks could be precipitated by normal airbag deployment in some asthmatics. Car companies have obviously worked to make airbag deployment as safe as possible.
10.5 Diseases associated with exposures
As mentioned above, local irritation of the upper and lower airways can occur from PM and VOCs. Exacerbation of existing asthma, COPD and other respiratory diseases is effected by exposure to air pollution and smoking exposure in cars. The risk of lung cancer is increased in persons exposed to many years of SHS exposure, as is the risk of cardiovascular disease.
In trying to infer health risks from environmental exposure in vehicles, many studies have been done evaluating professional drivers such as truck, bus and taxi drivers. Many of these studies show increased risk of diseases such as cancers and cardiovascular disease, but it is still difficult to make a causal link to in-vehicle pollution exposure based on these cohort studies, given the many other confounding factors of smoking, job stress and sedentary lifestyle. More studies need to be done to help us evaluate our risks. A large retrospective mortality study of American truck drivers from 1979 to 1990 found elevated proportional mortality rates for cardiovascular disease and lung cancer, especially among younger (less 55 years) long-haul truckers. Information about smoking habits was not available, but even after adjustment based on current rates of smoking, the authors propose that smoking habits are not fully responsible for the differences seen and exposure to diesel exhaust is likely to be a contributing factor. A study of over 2000 taxi drivers in Rome from 1950 to 1988 showed a lower overall mortality than expected, but an increase in diabetes and lung cancer. The risk of lung cancer was only seen in drivers enrolled in the later part of the study (from 1965 to 1975). It is unclear if occupational exposure accounted for this, but these drivers were probably exposed to heavier exposure. Myocardial infarction rates were increased in urban bus drivers in Sweden as compared with their rural counterparts, although no changes in cancer rates were seen. It is again difficult to attribute this strictly to environmental pollution exposure, but is reasonable to infer that the urban drivers have more exposure than the rural drivers. A study of urban bus drivers in New York City from the 1980s found that cardiovascular deaths and malignancy were elevated, especially cancer of the esophagus.
10.6 Diagnosis and management issues
Diagnosing respiratory disease or cardiovascular disease related to in-vehicle exposure is difficult. Epidemiologic studies, as mentioned, show defined trends in increased disease, but there is actually little data that provides a causal link to in-vehicle exposure of pollution. We can infer that the known health hazards of the implicated chemicals and particulate matter also hold true during exposure in vehicles (Table 10.1). More evidence with regards to exposure to smoke while in a car shows it is directly correlated to increased disease.
Table 10.1 Air pollution exposure
Components of air pollution | Symptoms of exposure | Highest level of exposure |
PM 2.5 | Irritant effect upper and lower airways; wheezing, cough | Heavy traffic, urban areas |
Exacerbation of asthma and lung disease | ||
PM 10 | Irritant effect upper and lower airways; wheezing, cough | Heavy traffic, urban areas |
Exacerbation asthma and lung disease, increased respiratory disease | ||
VOCs | Drowsiness, dizziness or headaches | In-car vs ambient environment ‘Out-gassing’ of cars |
CO | Headaches, dizziness, disorientation, nausea and fatigue | Rush hour, evening hours |
High exposure leads to death | ||
Nitrogen dioxide | Irritation of the eyes, nose and throat<br/> Exacerbation of lung disease and increased risk of lung infections | In-car vs ambient environment |
Ozone | Cough; exacerbation of asthma and lung disease | Lower inside cars vs ambient environment |
Cigarette smoke | Irritation of upper and lower airways; cough<br/> Exacerbation of asthma and lung disease, increased ear infections in children | For smoker<br/> SHS: in vehicle with person actively smoking; windows rolled up |
Increased risk of lung cancer and cardiovascular disease |
With any exposure, patients may be presenting with exacerbations of their underlying lung disease or new-onset disease. A medical history inquiring about how many hours they spend in a car may be important, including if they drive a vehicle for work. Discussing trends in their respiratory disease exacerbations with regards to time spent in a vehicle may also lead to clues. Having a patient find alternative transportation and seeing if this improves their symptoms may also help pinpoint vehicular exposure as a culprit.
In general, physicians should counsel patients to reduce the amount of time spent driving in cars. Alternate forms of transportation including subways, trains, buses and even biking and walking diminish environmental exposure. Avoiding peak traffic times, especially evening rush hour, will also decrease exposure. Another key element to decreasing emission exposure is properly maintaining vehicles and driving more efficient cars that produce fewer emissions. Specific attention should be paid to the automobile air conditioning system, including routine maintenance and replacement of filters and hoses as needed. This is especially important as many automobiles now recommend use of the air conditioning year round as a means to control humidity. Additionally, exposure to particulates from the roadway and surrounding traffic may be decreased by keeping windows closed and using the air conditioning system. Exposure to exhaust can be minimized by attention to the muffler and exhaust system. Proper tuning of the vehicle will also increase combustion efficiency and decrease particulate, VOC and pas phase pollutant production. A patient who is a professional driver and is experiencing respiratory problems would need to be counseled about the risks their vocation may be causing to their health.
The findings on SHS and disease have led to legislation for smoke-free indoor environments and education of parents concerning the effects of their smoking on their children’s health. Legislation is currently in place banning smoking in cars with children (usually under 16 or 18 years old) in Maine, Arkansas, Louisiana, California and Puerto Rico. Ten other states currently have bills under review. Nova Scotia became the first Canadian province to ban smoking with children in cars in April 2008 and has been followed by British Columbia and most recently Ontario.
More studies need to be performed in order to definitively link air pollution exposure in vehicles to increased disease, but the current data is certainly concerning. Legislation to decrease vehicle emissions and decrease the risk of SHS are also important avenues to decreasing exposure and safeguarding our health.
Further reading
Alfredsson, L. et al. (1993) Incidence of Myocaridal infarction and mortality from specific causes among bus drivers in Sweden. Int. J. Epidemiol. 22(1): 57-61.
Almeida, F. et al. (2006) Lung injury after airbag deployment: airbag lung. Injury Extra 37: 181-183.
Borgia, P. et al. (1994) Mortality among taxi drivers in Rome: a cohort study. Am. J. Ind. Med. 25(4): 507-517.
Chan, C.C. et al. (1991) Driver exposure to volatile organic compounds, CO, ozone, and NO2 under different driving conditions. Environ. Sci. Technol. 25: 964-972.
Dockery, D. et al. (1993) An association between air pollution and mortality in six cities. New Engl. J. Med. 329: 1753.
Hesterberg, T. et al. (2009) Non-cancer health effects of diesel exhaust: a critical assessment of recent human and animal toxicological literature. Crit. Rev. Toxicol. 39: 195-227.
International Center for Technology Assessment (July (2000)) In-car air pollution, the hidden threat to automobile drivers. Available from: www.nanoaction.org.
Janerich, D.T., Thompson, W.D., Varela, L.R. et al. (1990) Lung cancer and exposure to tobacco smoke in the household. New Engl. J. Med. 1990; 323: 632-636.
Overton, S. et al. Identification of volatile organic compounds in a new automobile. Scientific Information services. Available from: www.sisweb.com.
Rank, J. et al. (2001) Differences in cyclists and car drivers exposure to air pollution from traffic in the city of Copenhagen. Sci. Total Environ. 279: 131-136.
Rees, V.W. et al. (2006) Measuring air quality to protect children from secondhand smoke in cars. Am. J. Prev. Med. 31(5): 363-368.
Robinson, C.F. et al. (2005) Truck drivers and heart disease in the United States, 1979-1990. Am. J. Ind. Med. 47(2): 113-119.
US Department of Health, Human Services (USDHHS) (2006) The Health Consequences of Involuntary Exposure to Tobacco Smoke: a Report of the Surgeon General. Centers for Disease Control and Prevention: Rockville, MD.
Wilson, R. Sengler, J. et al. (1996) Particles in our Air: Concentraions and Health Effects. Harvard School of Public Health, Harvard University Press: Cambridge, MA, 1996.
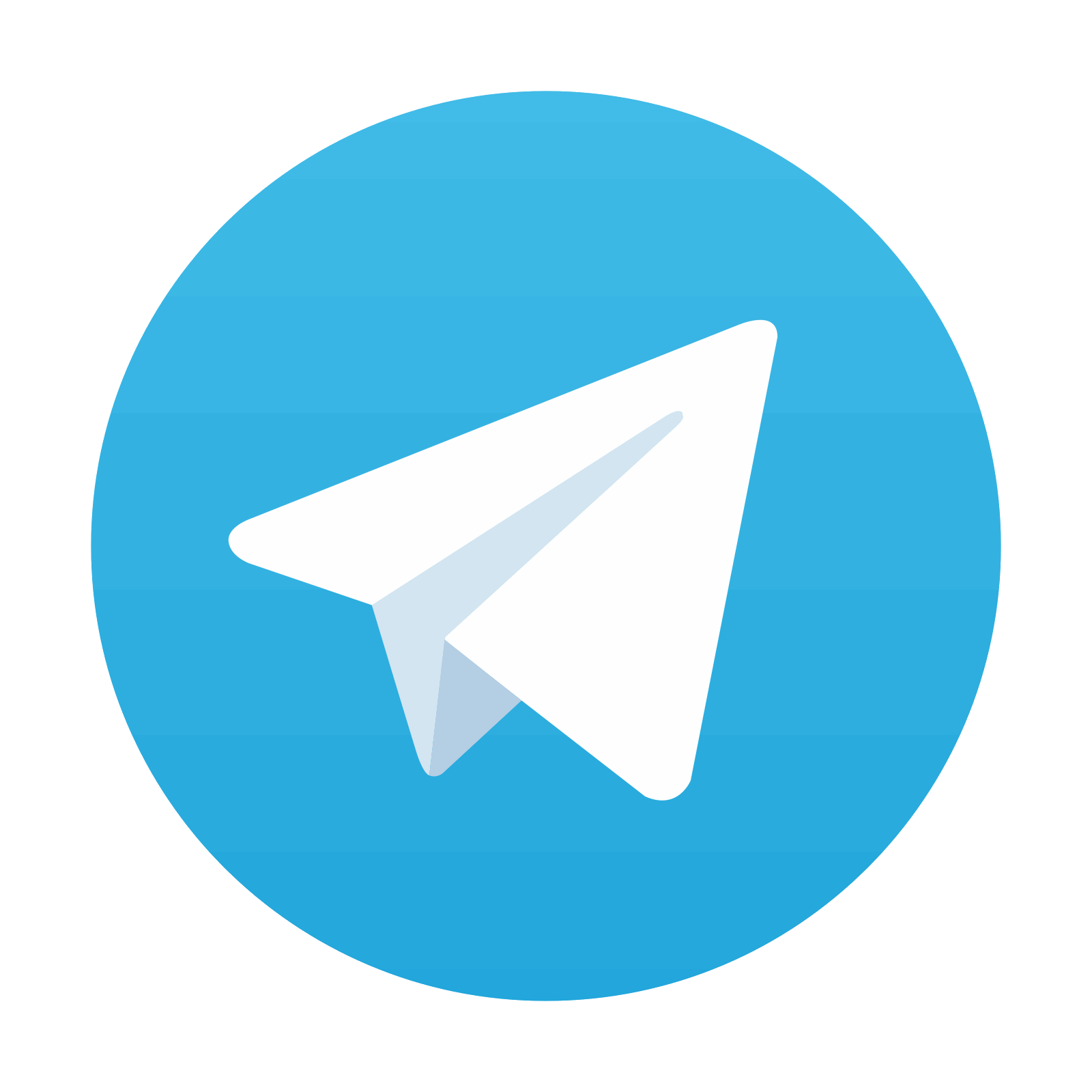
Stay updated, free articles. Join our Telegram channel

Full access? Get Clinical Tree
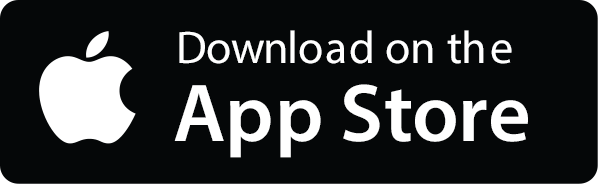
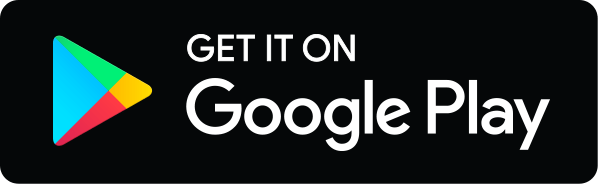