Fig. 3.1
An image of London during the Great Smog of 1952 . The tall buildings in the background are completely masked by the thickly polluted air. Source: Getty Images
Since that time, air pollution has been recognized as a major health concern across the globe, and unfortunately, this has not been purely limited to discrete events in time and space. There has been growing appreciation that chronic or repeated exposures to elevated airborne levels of particles and gases are responsible for short- and long-term adverse health outcomes in populations living in both industrialized and developing areas [2, 3]. In most cases, poor air quality has not been an undiscriminating uniform plague across people as it was in London in 1952. Rather, patterns of exposure to air pollution have evolved into a complex mosaic of disparities across lines of race, gender, age, geography, and socioeconomic strata. Concentrations of gaseous and particulate matter in the airborne microenvironment of defined subpopulations may vary at both local and regional levels, resulting in meaningful differences in health among these groups.
In this chapter, we will explore the unequal effects of air pollution across subpopulations divided by these lines. We will begin by reviewing the key air pollutants that are known to result in adverse respiratory health and their sources, then describe these health effects, and finally survey the evidence supporting disparities among subgroups. While not a comprehensive review, our discussion will reveal the complexities behind the ways in which different populations in the world suffer the varied consequences of airborne environmental hazards.
Air Pollutants
Ambient air pollution is often a toxic mixture of gaseous and particulate components , of which six primary air pollutants—sulfur dioxide (SO2), particulate matter (PM), ozone, nitric oxide (NO2), carbon monoxide (CO), and lead—have been individually demonstrated to bear health impact (Table 3.1 ). Certain pollutants were labeled as criteria pollutants after the 1970 Clean Air Act in the USA set standards to specifically limit each of their outdoor emissions. Another significant source of pollution worldwide is environmental tobacco smoke (ETS ), which is now perhaps the most well known of the air pollutants because of its widely-recognized adverse health effects. The impact of ETS is discussed elsewhere in this book and will not be specifically dealt with in this chapter.
Table 3.1
Major air pollutants and common sources
Pollutant | Source |
---|---|
PM2.5, PM2.5–10 | Natural sources: windblown soil, pollen, spores, sea salt, volcanic ash |
Man-made sources: road/street dust, agricultural and construction activities, particles from industrial and vehicular combustion, tobacco smoke, cooking activities | |
NOx, NO2 | High-temperature combustion activities from stationary sources such as thermal powerplants and incinerators, cooking, and mobile sources such as automobiles |
O3 | Produced in the atmosphere from photochemical reactions induced by ultraviolet light between pollutant nitrogen oxides (NOx) and volatile organic compounds. Levels typically vary throughout the day and across seasons depending on intensity of sunlight and temperature |
SO2 | Combustion by-product of fuels such as coal and petroleum. Sources include industrial emissions from refineries, diesel fumes, paper factories. Natural sources include volcanic eruptions |
CO | Burning of fossil or other organic fuels, vehicular exhaust, forest fires, volcanic eruptions |
Lead | Industrial sources such as exhaust from vehicles using leaded gasoline, smelters |
Among the six criteria pollutants, sulfur dioxide (SO2) is one that most commonly originates from the burning of fossil fuels such as coal and petroleum in power plants and factories, as well as in the burning of fuels for various vehicles and locomotive equipment [4]. In the case of the Great London smog, the severe cold led Londoners to burn more coal that winter, which along with the city’s usual industrial emissions, resulted in levels of SO2 and particles of several thousands of μg per m3. Although the institution of the Clean Air Act in the USA has resulted in an overall decline in SO2 emissions since 1970, elevated airborne concentrations continue to persist in regional portions of North America today and are subject to oxidation into sulfuric acid as the gas disperses across high altitudes and mixes with other particles and liquids [4].
The interaction of sulfur gases with the environment generates a mixture that notably also includes particles. In hindsight, these particles may have been the component of the London black smoke responsible for most of the ensuing health effects [4]. Particulate matter (PM) itself is a complex mixture of solids and/or liquid particles of various sizes and is classified by its aerodynamic equivalent diameter (PM10 (<10 μm), PM2.5 (<2.5 μm), and PM0.1 (<0.1 μm)), which is important in that particle size affects the ability to penetrate and deposit in human airways. Fine particles such as those <2.5 μm and ultrafine particles <0.1 μm have the greatest propensity to deposit further down into the air spaces, in contrast to larger particles which are trapped by the mucociliary apparatus of the nose and the upper airway. Whether from natural sources such as soil/dust, fires, and volcanoes, or from man-made sources such as industry, smoking, and usual human activity, PM has been shown to play a significant role in overall and cardiopulmonary mortality and morbidity with both short- and long-term exposures [5].
Another primary pollutant is ozon e, a highly reactive gas that is synthesized from photochemical reactions when UV light reacts with other pollutants such as nitrous oxides and volatile organic compounds in the atmosphere. The majority of exposure to ozone is in the ambient outdoor environment, with indoor ozone only generated by specific appliances or by penetration of outdoor air into indoor spaces. High levels in the ambient air are noted especially in the summer months in the USA and with acute exposures, can induce noticeable respiratory symptoms (chest pain, cough) as well as underlying airway inflammation and cellular injury [4].
In contrast to ozone, NO2 has gained recent interest as both an ambient and an indoor air pollutant. Indoor NO2 is generated by the burning of fossil fuels and is released from the operation of gas and kerosene stoves, heaters, and furnaces, often not vented to the outdoors. The effects of airborne NO2 may have particular implications for those with underlying respiratory conditions such as asthma and COPD [6, 7]. The last two criteria pollutants, carbon monoxide and lead, are both by-products of industrial and vehicular combustion and tend to be concentrated in urban areas, though levels of lead have diminished since the reduction in the use of vehicular leaded gasoline [4]. Lead has been associated with adverse health effects not wholly limited to the respiratory system, often with particular relevance to fetal, infant, and child neurocognitive development [8].
Impact on Respiratory Health
The evidence that has accumulated over the last half century linking acute and chronic exposures of these major air pollutants to morbidity and mortality is significant, and ongoing studies continue to strengthen such associations [9]. Particulate matter, for example, has been estimated to contribute to 800,000 premature deaths in the world annually [5]. Early studies of mortality from long-term outdoor exposures such as the “Harvard Six Cities” study and related follow-up studies observed that inhabitants of the most polluted cities had increased rates of all-cause and cardiopulmonary mortality compared to less polluted cities [5]. Subsequent studies that focused specifically on respiratory outcomes demonstrated associations between incremental increases in ambient PM and increased respiratory symptoms, lower lung function, and increased healthcare encounters for pulmonary disorders such as asthma and COPD, as well as more frequent lung infections in children and adults. The mechanisms behind such relationships have been proposed to involve the ability of particulates to induce inflammatory responses in human airway epithelial cells, which in turn cause oxidative stress and further airway damage [5].
Similarly, pollutants such as ozone are cytotoxic to cells in the airway and are also associated with local inflammatory responses and injury that may underlie the observed decrements in lung function and increase in bronchial hyper-reactivity. Some studies support the relationship of high-ozone days with increased hospital admissions for respiratory illness such as asthma [4], though it is difficult to separate the effects of ozone from the combined effects of other commonly occurring co-pollutants, thereby warranting further single-pollutant studies.
Ongoing efforts to fully characterize the health effects of air pollution have yielded consistent findings that link airborne exposures to respiratory morbidity but in ways not previously well understood. A recent multi-cohort study in Europe found ambient air pollution, particularly PM, to be linked to lung cancer [10], and new evidence on the relationship of increases in indoor NO2 to more severe asthma and COPD symptoms has led to work aimed at developing interventions to reduce indoor exposures [6, 7, 11]. This and future work will help to elucidate the true breadth of air pollution’s impact on human health .
Sources of Pollutants
As described above, air pollution can originate from both natural and anthropogenic sources , and patterns of dispersion have greatly been affected by global climate change and the spread of industrialization over the last century. Regardless of the source, human exposure to air pollution is unfortunately ubiquitous, and the type and extent of these exposures depend not only on where they are being generated but also on lifestyle factors that shape where individuals spend their time. Meteorological factors also play a major role, since ambient temperatures and weather conditions motivate varying degrees of insulation and ventilation in constructed spaces, which in turn affects the communication of outdoor and indoor environments.
In the USA, Americans spend over 90 % of their time inside [12], and therefore indoor sources of pollution can have a significant impact on health. Moreover, despite the impact of the Clean Air Ac t on ambient pollution, indoor levels of air pollution are not centrally monitored or regulated and therefore may pose unrecognized hazards for those who spend extended periods of time indoors. Indoor environments where people spend most of their time are commonly divided into three realms: the household, the school, and the workplace.
The level of exposure in each of these places relies on such characteristics as the type and frequency of activities being performed, the degree of enclosure and ventilation patterns of the building, and any efforts to filter or shield from the inhalation of airborne gases and particles. For example, common household activities have been known to generate high indoor levels of particulate matter. In one study of urban homes in Baltimore, three major sources of particulate matter in the homes were identified: smoking, sweeping, and stove use, with increasing frequency of these behaviors significantly associated with increasing levels of PM10 measured in the indoor air [13]. Cleaning activities contribute to indoor pollution, not only from the emissions from toxic cleaning agents used themselves, but also due to the re-suspension of particles in the settled dust. Similarly, NO2 concentrations within the home are correlated to both the presence of a gas stove and gas heater, as well as use of a space heater or stove/oven for heat [6], demonstrating the effects of cooking activities upon the generation of indoor NO2. Besides cleaning and cooking, other activities such as the burning of candles or incense, use of fireplaces/wood stoves and certain appliances, and smoking are common indoor sources of air pollution in the developed world.
In contrast, the burden of household air pollution in the developing regions of the world is largely the result of indoor burning of biomass fuels . Almost half of the world, mostly in the poorest of settings, depends on the burning of cheaper, but energy-inefficient, organic materials such as animal dung, charcoal, and crop waste, as well as kerosene and mixed fuels, in open fires in order to cook and heat homes. The indoor levels of smoke and particulate matter generated from this burning are orders of magnitude above those seen in developed regions and have been linked to significant mortality and morbidity from acute respiratory infections/pneumonia, COPD, and airway cancers [14].
Many of the same indoor activities common to personal residences pertain to school environments. Cleaning and heating activities within school buildings may contribute more to indoor levels of pollution. One specific source of indoor pollution relevant to schools that has gained recent interest is school bus exhaust-related pollutant exposure. Due to the common phenomenon of bus “idling” while waiting to pick up and drop off children at schools, as well as the general transport of children within buses, exposure to diesel fumes inside and around buses has been found to be a measurable source of particulate matter, elemental carbon, carbon monoxide, and other gaseous and particulate pollutants [15, 16]. This exposure has led to considerable concern regarding the effects of noxious diesel fumes on young children, and further research on this is underway.
A third portion of indoor time for a large number of the world’s adult population is spent at work. Occupational hazards remain a concern as the cause for a variety of acute and chronic respiratory conditions, including exposures that span from airborne industrial toxins to aerosolized agricultural pollutants to the common indoor exposures from routine human activity discussed above. Given its large scope, work-related exposures and their health impact is specifically discussed in another chapter of this book.
In contrast to the variety of indoor pollutant exposures thus far described, outdoor pollution often affects large populations at once and is not readily amenable to lifestyle modifications by the exposed individual. Pollution sources can be described as “stationary” sources, such as factories with smoke stacks, and “mobile” sources such as motor vehicle tail pipes [4]. Despite the lessons learned during the 1952 London Great Smog and other similar environmental disasters, industrial emissions continue to be a major source of ambient pollution, particularly in urban areas. Combustion of fuels by power plants, factories, and waste incinerators that directly release organic, acidic, and metallic compounds into the air, continue to dominate as sources of ambient pollution. Historically, in an effort to improve local air quality, industrial smoke stacks have been redesigned to be taller, removing their emissions from the mixing layers nearer to the ground and from the breathable air of the people who live around them [4]. However, the emissions from these tall stacks, which are often at higher temperature and linger longer in the suspended air, become more widely dispersed and are capable of blanketing a larger geographical area [17]. In contrast, domestic chimneys and other low-lying emissions disperse less and accordingly, contribute greater to local airborne pollution [17]. Vehicular traffic is another example of low-lying emissions that can rapidly elevate concentrations of particulate and gaseous pollution in the direct breathing space of an individual. Not only do vehicles contribute through by-products of combustion released from tail pipes, but operation of these vehicles over often long pathways of transport generate particulate emissions into the air that are released from tire wear and road abrasion [17].
Lastly, natural sources of outdoor pollution such as soil, dust, volcanic eruptions, and organic material such as pollen and fungi, can contribute significantly to ambient levels of particulate matter and gases and can cause meaningful health effects. For example, volcanic activity is known to release a unique diversity of particles and volatiles such as SO2, CO, CO2, HCL, HF, H2S, and radon in ash [18]. In the case of the Eyjafjallajökull eruption in Iceland, up to 25 % of the ash was found to be less than 10 μm, well within the respirable range. Health effects of such eruptions can extend for great distances, as in the case of Icelandic events that led to fallouts across the European continent, and can manifest with acute respiratory effects including symptoms of airway irritation and exacerbations in people with asthma and chronic bronchitis [18].
Evidence for Disparities
Disparities in the exposure to, and health effects from, air pollution are a uniquely relevant dilemma due to the intimate association between the environment and respiratory system. Thus, the inequalities in the environment that characteristically divide groups often coexist with differences in air quality, which in turn lead to disparities in respiratory health. Experts describe three mechanisms that may translate variations in the air quality of microenvironments into further differences in the health of their inhabitants [19]. The first is “differential exposure” where certain groups are found to be more exposed to air pollution sources, thereby violating the principle of environmental justice, which upholds that no one population should bear the brunt of toxic exposure over another [20]. The second mechanism is that of “differential susceptibility,” such that a given exposure to air pollution may have different effects on an individual due to specific personal and social vulnerabilities of people in a group. This is especially relevant to those individuals with pre-existing lung disease such as asthma and COPD. Lastly, the “social coping” mechanism includes factors that relate to socioeconomic status that may modify the ability of an individual to manage risk imposed by the threats faced, such as adequate access to healthcare [21]. These mechanisms are useful to consider when assessing the true burden of poor air quality for specific groups (Table 3.2).
Table 3.2
Pathways of environmental health disparities
Mechanism | Example |
---|---|
Differential exposure | A family who lives upon a major congested road. The exposure to traffic-related air pollution is higher for these individuals than those who may live on a private street |
Differential susceptibility | A young child in this household who plays in the front yard. He/she, as an active child, has higher minute ventilation and may have a less-developed immune system compared to the adults in the home, rendering him/her more susceptible to respiratory illness |
Social coping | The family has an income below the poverty line, and as a result cannot afford to relocate to avoid the pollution exposure, to enroll their child to a safer play environment, or to obtain private insurance to cover healthcare when the child becomes ill, all options which would minimize their risk of pollution-related health effects |
In the following sections, we will discuss the evidence for disparities in air pollution across various dividing lines—socioeconomic status, race, gender and age, and geography. While many of these divisions overlap, they highlight the ways in which various groups of people around the world disproportionately bear the burden of global air pollution.
Socioeconomic Status
Over the last several decades, inequalities in health across socioeconomic strata have been recognized for a variety of health outcomes. In one study comparing 22 nations across the European continent, those countries with lower socioeconomic status (SES ) (as measured by education, occupation, and income) had higher mortality and poorer health assessments [22]. Inequalities in overall mortality across Europe were found to be attributable to differences in rates of cardiovascular, smoking-, and alcohol-related mortality between people of different educational backgrounds. Moreover, variations between countries with regard to the relative contribution of these disease-specific mortalities were also found. In respiratory disease, inequalities between the rich and the poor have been recognized, with asthma morbidity known to be higher in areas in the USA with greater poverty [23]. While the exact causes of this phenomenon are incompletely understood, the link between poverty and lung disease illustrates the interdependence of socioeconomically driven environmental factors and respiratory health and reinforces the notion that pollution may have differential effects upon people who are disadvantaged.
To further understand this relationship, we can consider the conclusions of the WHO Commission on Social Determinants of Health , which attributed physical environments, among other factors pertaining to an individual’s living circumstances, to observed disparities in health [24]. As poverty itself may predispose disadvantaged groups to spend more time in poor-quality housing, work in toxic environments, and engage in hazardous behaviors (e.g., smoking) that directly affect surrounding air quality, greater exposure to a physically-damaging environment in disadvantaged circumstances would allow for further downstream inequalities of health [25].
As the Great London smog event illustrated, among the most pervasive of poor quality environments is that of polluted air. Subsequent work from other European studies has supported that chronic levels of air pollution are associated with disadvantaged populations, potentially more so than other environmental threats. Evidence for social inequalities being linked to higher concentrations of PM, NO2, and SO2 can be found across European nations, though there are a few studies demonstrating conflicting data with regard to the strength and direction of these relationships [19]. Despite such variations in findings regarding differential exposure highlighted by one set of reviewers, the authors conclude that as a whole, individuals from disadvantaged socioeconomic brackets suffer greater effects from air pollution and speculate that this is mediated perhaps by additional mechanisms such as comorbid conditions or social coping which may impact individual vulnerability [19].
In the United States as well, several studies support that persons of lower socioeconomic status are preferentially exposed to greater air pollution. An analysis of counties across the USA revealed that those areas with the worst air quality, as measured by annual and daily PM2.5, were also the poorest, compared with those counties with the best air quality [26]. In another report from North Carolina, predicted estimates of PM2.5 concentrations were 0.10 μg/m3 lower with each increase in interquartile range of median household income [27], further demonstrating that the burden of air pollution may disproportionately fall upon socioeconomically disadvantaged communities. More specific work by Bell and colleagues found that estimated exposure to the specific components of PM2.5 was higher for those who had less than a high school education and for unemployed persons, with larger disparities between the groups in some of the individual particle components than in PM2.5 overall [28]. Such exposures may have long-term health consequences , with several studies demonstrating that there is an increased risk of mortality related to PM2.5 exposure among groups of lower education and income [25, 29].
Perhaps the largest socioeconomic inequality which greatly impacts exposure to air pollution is one which affects three billion people worldwide, primarily in developing regions. Biomass fuel combustion is one of the worst polluters of the indoor environment and preferentially affects the world’s poor due to the fact that biomass fuels are among the cheapest of fuels. Its low rung on the “energy ladder,” whereby fuels low in cost also are least efficient and produce more pollution [14], results in a higher burden of pollution-related adverse respiratory effects among individuals living in parts of the world that cannot afford cleaner fuels. Since women and children are the most affected by domestic activities that require solid fuel, biomass burning will be discussed in greater detail in a later section of this chapter .
< div class='tao-gold-member'>
Only gold members can continue reading. Log In or Register a > to continue
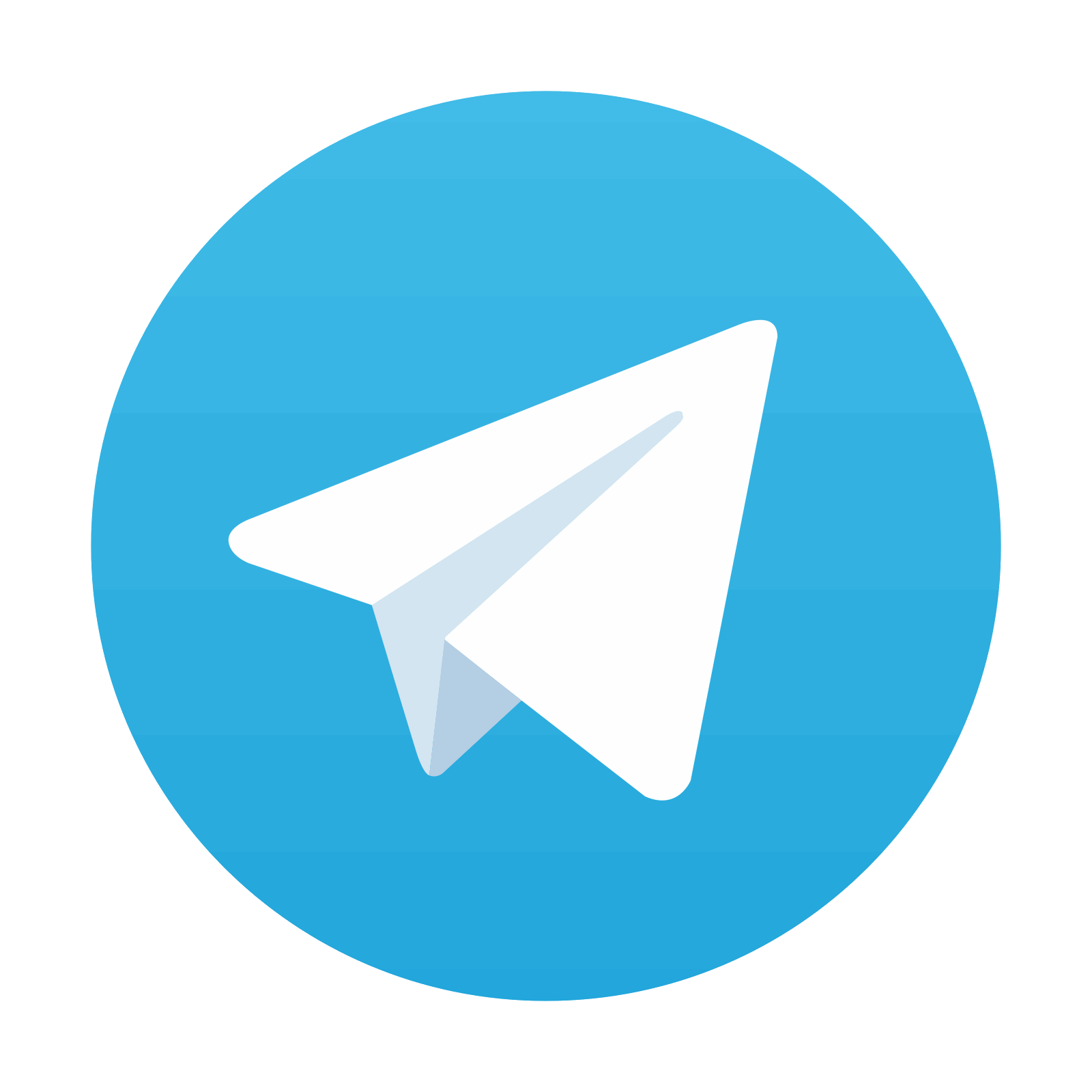
Stay updated, free articles. Join our Telegram channel

Full access? Get Clinical Tree
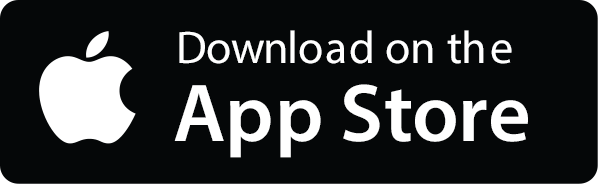
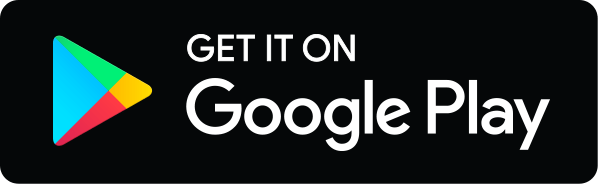