Attention ASE Members:
The ASE has gone green! Visit www.aseuniversity.org to earn free continuing medical education credit through an online activity related to this article. Certificates are available for immediate access upon successful completion of the activity. Nonmembers will need to join the ASE to access this great member benefit!
General Considerations
Recent guidelines have been published providing detailed guidance on specific echocardiographic diagnostic criteria for measurements of diastolic function, chamber dimensions, right ventricular (RV) function, and Doppler measurements. Also, guidelines have been published with respect to requirements for competence in basic and advanced perioperative transesophageal echocardiography (TEE), as well as focused cardiac ultrasound examinations. Increasingly, however, transthoracic echocardiography (TTE) and TEE are being used to monitor hemodynamics and direct therapy in critically ill patients. Case reports, observational studies, and state-of-the-art literature reviews have demonstrated the potential role of echocardiography in care and decision making for medical and surgical patients. Intensivists, trauma physicians, cardiologists, and anesthesiologists are now using TTE and TEE to provide hemodynamic assessments in patients with life-threatening illnesses such as sepsis, respiratory failure, congestive heart failure (CHF), shock, and traumatic injuries, as well as patients with significant respiratory and cardiac diseases undergoing noncardiac surgery and high-risk noncardiac procedures. Ramp tests and weaning protocols using echocardiographic monitoring are used in left ventricular (LV) assist device (LVAD) optimization and to guide the removal of assist devices. The clinical impact on diagnosis, decision making, and management has led governing bodies to address the potential value of echocardiography in unstable medical and noncardiac surgical patients. The recent consensus statement by the American Society of Echocardiography (ASE) on focused echocardiography and the standardization of the basic perioperative transesophageal echocardiographic examination have led to the need for guidelines regarding when, and how, to use echocardiography as a quantitative monitoring tool. By definition, we propose that echocardiography is being used as a monitoring tool if, after a diagnostic assessment, repetitive hemodynamic or anatomic assessments are being made over a period of minutes, hours, or days in the same patient to guide management. In this context, this covers all areas in which echocardiography is monitoring a therapeutic cardiac or noncardiac intervention, whether it is fluid resuscitation, pericardial effusion monitoring, ramp or weaning protocols in LVAD cases, or during perioperative care.
General Considerations
Recent guidelines have been published providing detailed guidance on specific echocardiographic diagnostic criteria for measurements of diastolic function, chamber dimensions, right ventricular (RV) function, and Doppler measurements. Also, guidelines have been published with respect to requirements for competence in basic and advanced perioperative transesophageal echocardiography (TEE), as well as focused cardiac ultrasound examinations. Increasingly, however, transthoracic echocardiography (TTE) and TEE are being used to monitor hemodynamics and direct therapy in critically ill patients. Case reports, observational studies, and state-of-the-art literature reviews have demonstrated the potential role of echocardiography in care and decision making for medical and surgical patients. Intensivists, trauma physicians, cardiologists, and anesthesiologists are now using TTE and TEE to provide hemodynamic assessments in patients with life-threatening illnesses such as sepsis, respiratory failure, congestive heart failure (CHF), shock, and traumatic injuries, as well as patients with significant respiratory and cardiac diseases undergoing noncardiac surgery and high-risk noncardiac procedures. Ramp tests and weaning protocols using echocardiographic monitoring are used in left ventricular (LV) assist device (LVAD) optimization and to guide the removal of assist devices. The clinical impact on diagnosis, decision making, and management has led governing bodies to address the potential value of echocardiography in unstable medical and noncardiac surgical patients. The recent consensus statement by the American Society of Echocardiography (ASE) on focused echocardiography and the standardization of the basic perioperative transesophageal echocardiographic examination have led to the need for guidelines regarding when, and how, to use echocardiography as a quantitative monitoring tool. By definition, we propose that echocardiography is being used as a monitoring tool if, after a diagnostic assessment, repetitive hemodynamic or anatomic assessments are being made over a period of minutes, hours, or days in the same patient to guide management. In this context, this covers all areas in which echocardiography is monitoring a therapeutic cardiac or noncardiac intervention, whether it is fluid resuscitation, pericardial effusion monitoring, ramp or weaning protocols in LVAD cases, or during perioperative care.
Scope of Work
Multidisciplinary guidelines published by the American Society of Anesthesiologists and the Society of Cardiovascular Anesthesiologists in 2010 recommend the use of TEE in patients who are undergoing noncardiac surgery and exhibit persistent hypotension or hypoxia despite intervention (category B2 and B3 evidence). Clinical data exist on the usefulness of TEE and TTE in adult patients in critical care units or emergency departments who are hemodynamically unstable or who need noninvasive hemodynamic monitoring. However, prospective, randomized clinical trials are lacking on the morbidity, mortality, and cost-effectiveness of echocardiography in this population. Because of the ethical and logistic challenges in conducting randomized clinical trials on patients who are hemodynamically compromised, expert opinion is heavily relied on for criteria and guidelines. Although expert opinion and a significant body of literature support the use of echocardiography as a tool to guide therapy in patients who are critically ill, standard guidelines that define when and how echocardiography can be used to guide medical and surgical therapy have not been published. This document summarizes the literature that supports the use of echocardiography as a monitoring tool in specific clinical settings. The specific parameters that are used are discussed first, followed by guidelines for their use in specific clinical scenarios.
I
Echocardiographic Monitoring Tools
Echocardiography has the ability to noninvasively evaluate and track both RV and LV hemodynamic status. In the following section, we discuss echocardiography-based hemodynamic measurements that can be used to serially measure the response to medical interventions such as fluid and drug therapy.
Echocardiography can be used to manage the response to fluid resuscitation in critically ill patients who are at risk for heart failure or tissue hypoperfusion. Traditional monitors, such as central venous catheters or pulmonary artery (PA) catheters, have not been found to improve survival or decrease length of stay in hospitalized patients. PA catheters, when used to estimate left atrial (LA) pressure (LAP), can cause PA rupture. They are typically calibrated with saline-filled transducers at the bedside and therefore can be inaccurate in the assessment of LV filling pressures because of waveform artifacts, damping, and airway pressure, especially in ventilated patients. Furthermore, PA catheters and central venous catheters do not accurately measure LV diastolic dysfunction, which is more predictive of mortality in hospitalized patients. Echocardiography has the potential to noninvasively measure left-sided filling pressures and guide volume assessments in hospitalized patients who may be at risk for both systolic and diastolic heart failure. Serial examination of two-dimensional (2D) and Doppler indices can be used to monitor stroke volume (SV) and overall volume status. Several studies have recently shown the benefits of goal-directed fluid therapy in surgical patients. In this setting, 2D echocardiography with Doppler can measure changes in SV in response to either a fluid bolus or the administration of a diuretic, while monitoring LAP with transmitral and tissue Doppler imaging (TDI) as well as right atrial pressure (RAP) using vena cava respiratory dynamics. The limitation of echocardiography in this setting is that it cannot perform continuous monitoring, and it requires meticulous attention to sample volume placement. Current guidelines suggest that specific parameters be used to detect pressures that are elevated or normal, and not for exact values. In the setting of advanced decompensated systolic heart failure, using serial TDI measurements may be inaccurate in monitoring filling pressures. Therefore, different recommendations exist for monitoring LAPs in patients with systolic or diastolic heart failure ( Table 1 ).
Monitoring parameter Role Reference | System requirements | Important technical features | Specific values to use while guiding interventions |
---|---|---|---|
Transmitral E/e′ for LAP Nagueh et al . | Pulsed Doppler Tissue Doppler | Doppler alignment End-expiratory acquisition | E/e′ < 8; normal LVEF = normal LAP E/e′ ≥ 13; normal LVEF = increased LAP E/A > 2; DT < 150 msec; depressed LVEF = increased LAP E/A < 1 and E < 50 cm/sec; depressed LVEF = normal LAP |
IVC size /collapsibility, for RAP Rudski et al . , Brennan et al . | 2D harmonic | Visualization throughout the respiratory cycle | Size ≤ 2.1 cm; collapses >50% during sniff = RAP 0–5 mm Hg Size > 2.1 cm; collapses >50% during sniff = 5–10 mm Hg Size > 2.1; collapses <50% during sniff = 10–20 mm Hg |
LV and RV chamber size, areas, and volumes for intravascular volume status and function Lang et al . | 2D harmonic | Optimal alignment; endocardial border visualization ∗ ; avoiding foreshortening | Normal ranges: LVIDD men 4.2–5.9 cm ∗ LVIDD women 3.9–5.3 cm ∗ LVEDV 46–106 mL women LVEDV 62–150 mL men LVESV 14–42 mL women LVESV 21–61 mL men RV FAC ≥ 35% |
LVOT stroke distance for intravascular volume status Ristow et al . | 2D harmonic; pulsed Doppler | Optimal Doppler alignment; visualization of aortic valve leaflet opening | Normal values VTI > 18 cm |
PASP for right-sided hemodynamics Lahm et al . | Pulsed Doppler Continuous-wave Doppler | Optimal Doppler alignment | Normal value: PASP < 35 mm Hg |
TAPSE RV s for RV function during fluid administration Rudski et al . | M-mode (TAPSE) Tissue Doppler (RV s′) | Optimal standard 4C view and alignment with TV annulus and right ventricle | Normal value: TAPSE ≥ 16 mm RV s′ ≥ 10 cm/sec |
∗ LV and transmitral Doppler measurements are at the plane of the MV leaflet tips. Please refer to Figure 7 in Lang et al . for example images of biplane LVEDV and LVESV measurements and Figure 9 in Rudski et al . for RV FAC image measurements.
Two-Dimensional Echocardiographic Monitoring Parameters
LV Chamber Dimensions
Cardiac chambers can be measured serially to look for ventricular filling during focused examination of volume status. A small LV internal diameter at end-diastole (LVIDD) can be indicative of hypovolemia; care should be taken to not mistake a low LV internal diameter at end-systole (LVIDS) with hypovolemia. Hypovolemia is best monitored using end-diastolic measurements, because a low LVIDS could also depict decreased systemic vascular resistance (SVR), increased inotropic state, or decreased ventricular filling. In hypovolemia, both LVIDD and LVIDS are decreased, while in the setting of decreased SVR, LVIDD is normal and LVIDS is decreased. Both RV and LV internal diameters can be measured serially to monitor response to fluids. Measurements should be made in the same echocardiographic view and serially compared. LV dimensions (LVIDD and LVIDS) can be measured in the transthoracic echocardiographic parasternal short-axis (SAX) or long-axis (LAX) view using either 2D linear measurements or M-mode imaging at the LV minor axis, 1 cm distal to the mitral valve (MV) annulus at the MV valve leaflet tips. The same measurements can also be obtained with 2D TEE in the midesophageal (ME) two-chamber view at the MV leaflet tips or using M-mode imaging in the transgastric LV SAX view at the midpapillary level. The SAX or LAX view can be used for LVIDD and LVIDS, and the transgastric midpapillary SAX view provides a critical view in monitoring for the development of regional wall motion abnormalities with any of the three major epicardial vessels. However, the LAX is preferred because it may be less prone to improper alignment and thus likely to detect interval changes in dimension size and fractional shortening. Reference ranges for LVIDD are 3.9 to 5.3 cm in women and 4.2 to 5.9 cm in men.
Inferior Vena Cava (IVC) Size and Collapsibility
Hypovolemic patients can be identified using measurement of both size and collapsibility of the IVC for estimation of RAP. Fluid responsiveness of patients can be measured using 2D or M-mode assessment of IVC parameters. Inspiration in normovolemic, spontaneously breathing patients causes negative intrathoracic pressure and a decrease in IVC size. An exaggerated response in IVC collapse occurs in patients in the hypovolemic state during inspiration. Routine measurements in size of the IVC and collapsibility with respiration have been used in patients with shock to reliably guide fluid management decisions. The transthoracic echocardiographic subcostal window can be used to view the IVC in the sagittal plane by angling and rotating the transducer to the left from the subcostal four-chamber (4C) view. M-mode imaging allows high–frame rate measurements of size changes throughout the respiratory cycle ( Figure 1 ). Care must be taken to ensure that the IVC does not translate out of the imaging plane during portions of the respiratory cycle, leading to “pseudocollapse.” Because IVC collapse will not occur in patients on positive pressure ventilation due to inspiration-induced reductions in venous return, it should not be used to monitor RAP in this setting. Although isolated measurements of IVC collapsibility have been used to predict response to fluid management, there are fewer data to support serial measurements of IVC collapsibility to guide fluid management. Changes in the IVC collapsibility index of >10% have been observed with 2-kg weight reductions after hemodialysis. In this setting, collapsibility index was better than dry-weight assessments in predicting adverse outcomes associated with hemodialysis. Values for estimation of RAP using the IVC collapsibility index are referenced in Table 1 from the guidelines for the echocardiographic assessment of the right heart in adults.
Doppler Monitoring Parameters
Mitral Inflow
Mitral inflow velocities, both peak early diastolic velocity (E) and late diastolic velocity (A), are commonly used to determine patterns of diastolic dysfunction and can also be used to serially monitor LAP. The mitral E wave represents the LA-LV gradient during early diastole and thus is preload dependent. The mitral A wave is the LA-LV gradient during late diastole and is affected by changes in LV diastolic function and LA compliance. Mitral inflow velocities (E wave, A wave, DT, and E/A ratio) are measured in the apical 4C view with TTE and the ME 4C view with TEE using PW Doppler ( Figure 2 ). The sampling volume should be 1 cm distal to the MV annulus or at the leaflet tips during diastole, with a sampling gate of 1 to 3 mm. Comprehensive explanations of mitral inflow indices for classification of diastolic function are described in the ASE recommendations for the evaluation of LV diastolic function. It is the recommendation of the writing group that the E- and A-wave velocities be used in conjunction with the annular velocities when monitoring for changes in filling pressures or diastolic function. Figure 2 displays the recommended sample volume positions for monitoring the tissue Doppler measurements of e′ and transmitral measurements of the E and A waves. Although pulmonary venous assessments of systolic filling fractions have proved feasible for monitoring LAP with TEE in an elective setting, specific cutoffs for normal and abnormal filling pressures have not been provided, and their feasibility for monitoring LV filling pressures by TTE has not been demonstrated.
TDI
PW TDI is a sensitive indicator of LV diastolic function. TDI measures mitral annular velocities during both systole and diastole at end-expiration. TDI is used to measure e′, the peak early velocity of the mitral annulus. Studies have found e′ to be less load dependent than other measures of diastolic function, such as mitral inflow and pulmonary vein flow velocities. The measurement of E/e′, where E is the mitral inflow peak early diastolic velocity, is a reliable estimate of LAP when systolic function is normal ( Table 1 ). Therefore, serial E/e′ measurements are practical and reliable measurements that can be performed as a serial assessment of LAP to guide fluid therapy in ambulatory and hospitalized subjects at risk for heart failure. Measurement of e′ is best performed in the ME 4C view on TEE or the apical 4C view on TTE, where Doppler angles are well aligned with the lateral and septal (or medial) MV annulus ( Figure 2 ). Septal e′ measurement by TEE may not be equivalent with that by TTE because of potential misalignment of the Doppler beam with the direction of tissue motion in the ME 4C view. Care should be taken to measure this within 20° of angulation of mitral annular motion. The velocity scale should be set to 20 cm/sec below and above the baseline. Both septal and lateral TDI velocities should be taken and the two averaged for the measurement of E/e′. Although averaging may be used for overall assessments of LAP, use of medial e′ alone may be better for serial assessments of LAP. On the other hand, septal mitral annular e′ measurements may not accurately reflect LV diastolic function in the setting of septal wall motion abnormalities or RV dysfunction.
Calculated Monitoring Parameters
SV, Cardiac Output (CO), and SVR Calculations
Measurement of SV of both the right and left ventricles can be performed readily using PW Doppler. These measurements can be reliably obtained using TTE and TEE. Assessment of CO is important in determining responses to medical and surgical therapies, such as administration of inotropic agents for the treatment of right and left heart failure. Using PW Doppler, SV through a site (such as the RV outflow tract [RVOT] or LV outflow tract [LVOT]) can be calculated using two variables: (1) the velocity-time integral (VTI), or stroke distance, and (2) the cross-sectional area of the site (using the diameter of the RVOT or LVOT). Thus,
Stroke volume (or flow) = Cross sectional area (cm 2 ) × VTI (cm).
Because CO = SV × heart rate, both right- and left-sided CO can be serially measured noninvasively before and after medical therapies. In clinical practice, RV SV is calculated by using the parasternal SAX view. PW Doppler can be used to acquire the RVOT VTI (in centimeters) in this view. Because of difficulties in measuring the RVOT diameter, it is recommended that the RVOT VTI be used as a monitor of RV SV. LV SV is calculated on TTE using the apical five-chamber or LAX view. The deep transgastric LAX view is used in TEE, whereby the PW Doppler sample volume is placed in LVOT. Gradients across the aortic valve (in the setting of prosthetic valve thrombolysis monitoring) should be acquired with continuous-wave Doppler monitoring in this location. Measurement of the baseline LVOT diameter is best accomplished in the ME LAX view. The LVOT diameter can be used to calculate area, which when combined with the LVOT VTI and heart rate can be used to calculate SV and CO. Using IVC collapsibility indices to estimate RAP, and arm blood pressure measurements to calculate mean arterial pressure, SVR (in Wood units) can be calculated as
To convert this to conventional SVR units (dynes · sec/cm 5 ), this value should be multiplied by 80. The limitations of echocardiographic measurements of SV, CO, and time-velocity integrals in the LVOT are that all measurements require accurate alignment with the LVOT, and consistent sampling should occur just beneath the aortic valve. The use of an LVOT diameter adds a second potentially more significant error measurement, and it was the recommendation of the committee that stroke distance (i.e., LVOT and RVOT time-velocity integrals) alone be used for serial measurements, with the assumption that LVOT diameter remains constant.
RV Systolic Function
Echocardiographic evaluation of right heart function at the bedside is critical in the management of right heart failure, a common and serious diagnosis in intensive care unit patients. Because of a lower systolic elastance, the right ventricle is more sensitive to afterload then the left ventricle. Simple, noninvasive measurements of RV function can be completed using several indices ( Table 1 ). Tricuspid annular plane systolic excursion (TAPSE) is less preload dependent than other markers of RV function and is performed in patients using both TTE and TEE. TAPSE and RV s′ can be measured with TTE in the apical 4C view and with TEE using the ME 4C view or transgastric view. For TAPSE, the M-mode cursor is directed through the lateral annulus of the tricuspid valve, and the distance of annular motion during systole is measured longitudinally. The view that provides optimal longitudinal alignment should be used. A TAPSE measurement of <16 mm, or s′ < 10 cm/sec, is highly specific for RV dysfunction, and both can be used to serially monitor RV systolic function. RV internal diameter in diastole (RVIDD) and fractional area change (FAC) measurements can be measured routinely in the apical 4C view on TTE and in the ME 4C view with TEE. RVIDD and the RVIDD/LVIDD ratio should be measured at the widest point of the right ventricle in a standardized 4C plane. Although normal and abnormal values for longitudinal strain are still to be determined, this parameter has been used to monitor RV systolic function during therapeutic interventions in pulmonary hypertension.
PA Systolic Pressure
Besides serial quantification of RV function, pulmonary pressures can also be evaluated by calculating the RV-RA gradient using the modified Bernoulli equation (4 V 2 ). Using the peak tricuspid regurgitant jet velocity as V , the RV-RA gradient can be calculated. Because RAP can be determined by assessing IVC size and collapsibility, PA systolic pressure can be estimated as RAP + RV-RA gradient, where RV-RA gradient is 4 × (peak tricuspid regurgitant jet velocity). The peak tricuspid regurgitant jet velocity is measured using continuous-wave Doppler parallel to the tricuspid regurgitant jet. This can be performed with TTE in the apical 4C view, parasternal SAX view, or RV inflow view. Doppler through the tricuspid valve in TEE is best performed in either the ME 4C view or the RV inflow view obtained from transducer angles that align the Doppler cursor parallel to the color Doppler jet. An additional transesophageal view that is useful for Doppler alignment is obtained by rotating the viewing angle to 130° to 145° to obtain an apical LAX plane and then rotating clockwise to visualize the tricuspid valve regurgitant jet using current guidelines. Although measurements are taken in multiple views with both TTE and TEE, the highest velocity signal should be used for serial measurements (as this represents the most parallel alignment).
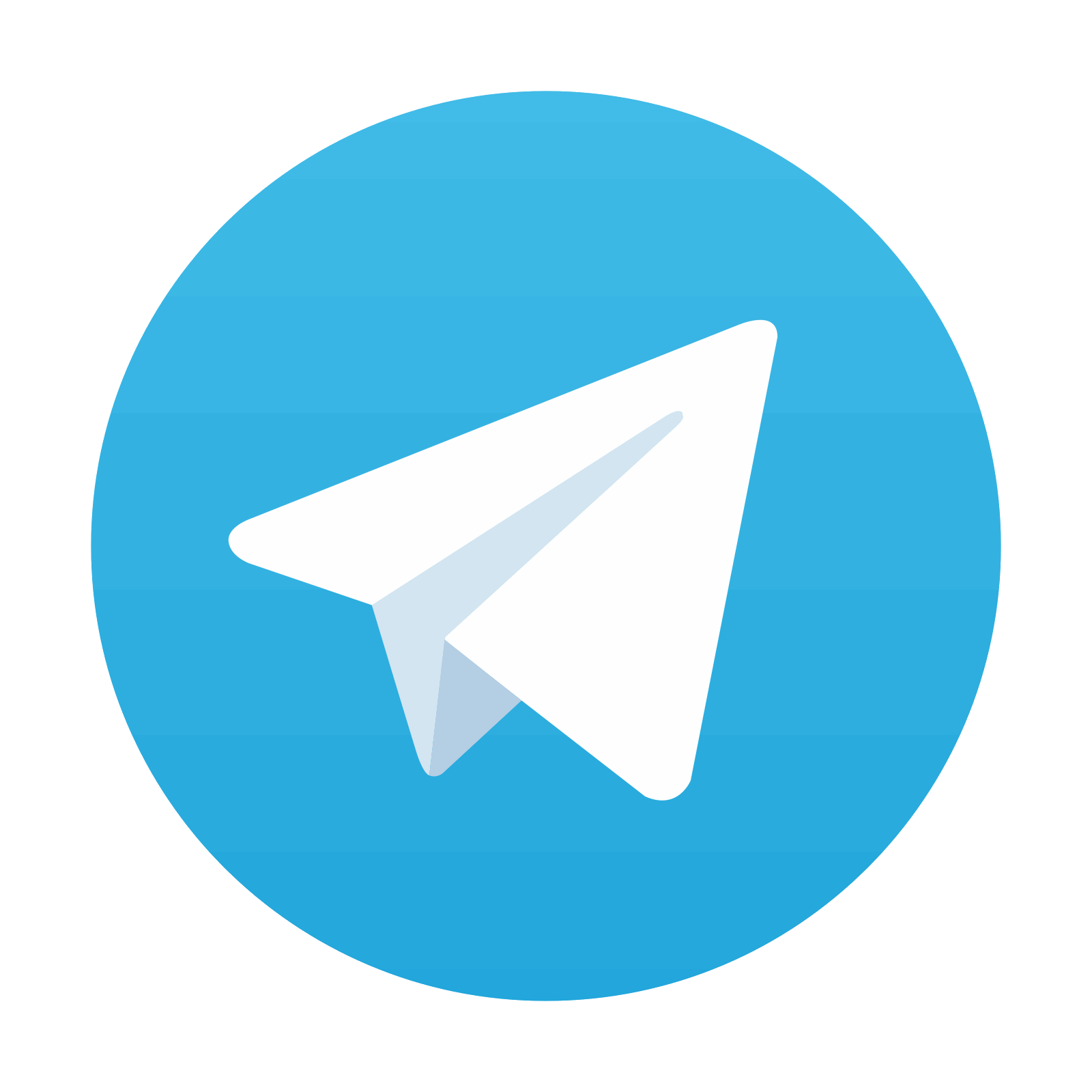
Stay updated, free articles. Join our Telegram channel

Full access? Get Clinical Tree
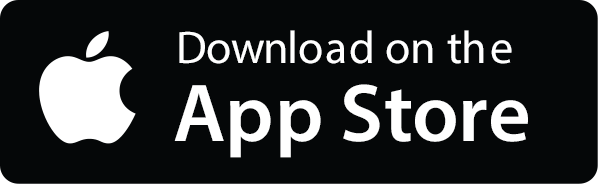
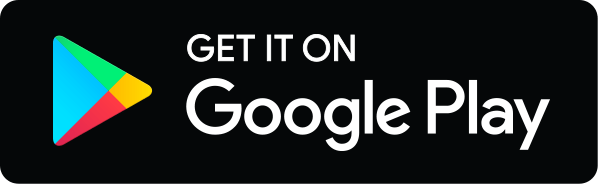