Attention ASE Members:
The ASE has gone green! Visit www.aseuniversity.org to earn free continuing medical education credit through an online activity related to this article. Certificates are available for immediate access upon successful completion of the activity. Nonmembers will need to join the ASE to access this great member benefit!
I
Update on Knowledge of Ultrasound Physics and Instrumentation
Since the 2001 document, considerable progress has been made in the area of improving the visualization of a commercially available ultrasound contrast agent (UCA) for left ventricular (LV) opacification (LVO) and perfusion. With regard to details on the composition of commercially available microbubbles and microbubble physics, please refer to the “Contrast Agents” and “Contrast-Specific Ultrasound Imaging” sections in the 2008 ASE consensus statement. Contrast enhancement for LVO using low–mechanical index (MI) harmonic imaging has been available on all ultrasound systems marketed within the past decade, and real-time very low MI techniques are available on nearly all commercially available systems. By definition, very low MI represents values < 0.2, low MI represents values < 0.3, intermediate MI represents values of 0.3 to 0.5, and high MI is any MI that exceeds 0.5. The real-time very low MI techniques permit the enhanced detection of microbubbles within the LV cavity and myocardium. Although myocardial perfusion imaging is not an approved indication for UCAs, these very low MI imaging techniques have been used in multiple clinical studies to examine perfusion and improve the detection of coronary artery disease in the emergency department, improve the detection of coronary artery disease during stress testing, and improve the diagnostic evaluation of cardiac masses. Therefore, sonographers should be familiar with the advantages and drawbacks of each contrast imaging method ( Table 1 ) and the physics related to each technique ( Figure 1 ).
Descriptor | Company Manufacturer(s) | Tissue cancelation technique | Advantage(s) | Disadvantage(s) |
---|---|---|---|---|
Pulse-inversion Doppler and very low MI ∗ | Philips Sonos/iE33 Toshiba Aplio/Xario GE 1.5-, 1.6-, and 1.7-MHz transducers | Alternating polarity | High resolution | Attenuation and dynamic range |
Power modulation and very low MI ∗ | Philips Sonos/iE33 GE 2.1- and 2.4-MHz transducers | Alternating amplitude | High sensitivity | Resolution, image quality, and dynamic range |
Contrast pulse sequencing and very low MI ∗ | Siemens Acuson | Both alternating polarity and alternating amplitude | Image quality and high sensitivity | Attenuation and dynamic range |
Low-MI † harmonic (LVO) | All vendors | B-mode; no cancelation | Image quality | Decreased contrast sensitivity, apical swirling, and no perfusion |

Pulse-inversion Doppler (originally developed by Advanced Technology Laboratories, now used by GE Healthcare, Little Chalfont, United Kingdom) is a tissue cancelation technique that overcomes motion artifacts by sending multiple pulses of alternating polarity into the cavity and myocardium. Although pulse-inversion Doppler provides excellent tissue suppression and high resolution by receiving only even-order harmonics, there is significant attenuation, especially in the basal myocardial segments of apical windows. Power modulation (originally developed by Philips Medical Systems, Andover, MA) is a technique that improves the signal-to-noise ratio at very low MIs (0.05–0.20). This technique is also a multipulse cancelation technique, only here, the power, or amplitude, of each pulse is varied. The low-power pulses create a linear response, whereas the slightly higher power pulse results in a linear response from tissue but a nonlinear response from microbubbles. The linear responses from the two different pulses (the amplified low-power pulse and the slightly higher power pulse) can be subtracted from each other. The transducer then only detects the nonlinear behavior, which emanates exclusively from the microbubbles. Power modulation also detects fundamental nonlinear behavior but does not have the resolution and image quality that pulse inversion offers. Contrast pulse sequencing (originally developed by Siemens Medical Solutions USA, Mountain View, CA) combines these multipulse techniques by interpulse phase and amplitude modulation, which although more complex has the purpose of enhancing nonlinear activity from microbubbles at a low MI and canceling out the linear responses from tissue. Contrast imaging with each specific pulse-sequence scheme can be used at very low MIs (<0.2) to assess LVO and myocardial contrast perfusion in real time with excellent spatial resolution. Sonographers should be aware of the variations in pulse-sequence schemes and use them if available whenever contrast is required ( Table 1 ). The advantage, compared with B-mode low-MI harmonic imaging (LVO), is that there is better tissue cancelation and enhanced contrast from microbubbles. However, not all vendors have real-time very low MI imaging software available, and in these settings, low-MI (<0.3) harmonic imaging should be used.
This document provides instructions on how to set up very low MI real-time imaging, and the video examples provide specific examples as well as potential artifacts. The writing group recommends that sonographers who are just beginning to use UCAs, or who do not have very low MI imaging software available, start with the low-MI harmonic imaging methods described in Table 1 . We recognize that experience is a critical factor in performing any aspect of ultrasound imaging, and we recommend to all sites that they work with their local contrast agent representatives to optimize contrast with low-MI imaging techniques and with their specific ultrasound vendors on how to effectively use real-time very low MI imaging software.
I
Update on Knowledge of Ultrasound Physics and Instrumentation
Since the 2001 document, considerable progress has been made in the area of improving the visualization of a commercially available ultrasound contrast agent (UCA) for left ventricular (LV) opacification (LVO) and perfusion. With regard to details on the composition of commercially available microbubbles and microbubble physics, please refer to the “Contrast Agents” and “Contrast-Specific Ultrasound Imaging” sections in the 2008 ASE consensus statement. Contrast enhancement for LVO using low–mechanical index (MI) harmonic imaging has been available on all ultrasound systems marketed within the past decade, and real-time very low MI techniques are available on nearly all commercially available systems. By definition, very low MI represents values < 0.2, low MI represents values < 0.3, intermediate MI represents values of 0.3 to 0.5, and high MI is any MI that exceeds 0.5. The real-time very low MI techniques permit the enhanced detection of microbubbles within the LV cavity and myocardium. Although myocardial perfusion imaging is not an approved indication for UCAs, these very low MI imaging techniques have been used in multiple clinical studies to examine perfusion and improve the detection of coronary artery disease in the emergency department, improve the detection of coronary artery disease during stress testing, and improve the diagnostic evaluation of cardiac masses. Therefore, sonographers should be familiar with the advantages and drawbacks of each contrast imaging method ( Table 1 ) and the physics related to each technique ( Figure 1 ).
Descriptor | Company Manufacturer(s) | Tissue cancelation technique | Advantage(s) | Disadvantage(s) |
---|---|---|---|---|
Pulse-inversion Doppler and very low MI ∗ | Philips Sonos/iE33 Toshiba Aplio/Xario GE 1.5-, 1.6-, and 1.7-MHz transducers | Alternating polarity | High resolution | Attenuation and dynamic range |
Power modulation and very low MI ∗ | Philips Sonos/iE33 GE 2.1- and 2.4-MHz transducers | Alternating amplitude | High sensitivity | Resolution, image quality, and dynamic range |
Contrast pulse sequencing and very low MI ∗ | Siemens Acuson | Both alternating polarity and alternating amplitude | Image quality and high sensitivity | Attenuation and dynamic range |
Low-MI † harmonic (LVO) | All vendors | B-mode; no cancelation | Image quality | Decreased contrast sensitivity, apical swirling, and no perfusion |
Pulse-inversion Doppler (originally developed by Advanced Technology Laboratories, now used by GE Healthcare, Little Chalfont, United Kingdom) is a tissue cancelation technique that overcomes motion artifacts by sending multiple pulses of alternating polarity into the cavity and myocardium. Although pulse-inversion Doppler provides excellent tissue suppression and high resolution by receiving only even-order harmonics, there is significant attenuation, especially in the basal myocardial segments of apical windows. Power modulation (originally developed by Philips Medical Systems, Andover, MA) is a technique that improves the signal-to-noise ratio at very low MIs (0.05–0.20). This technique is also a multipulse cancelation technique, only here, the power, or amplitude, of each pulse is varied. The low-power pulses create a linear response, whereas the slightly higher power pulse results in a linear response from tissue but a nonlinear response from microbubbles. The linear responses from the two different pulses (the amplified low-power pulse and the slightly higher power pulse) can be subtracted from each other. The transducer then only detects the nonlinear behavior, which emanates exclusively from the microbubbles. Power modulation also detects fundamental nonlinear behavior but does not have the resolution and image quality that pulse inversion offers. Contrast pulse sequencing (originally developed by Siemens Medical Solutions USA, Mountain View, CA) combines these multipulse techniques by interpulse phase and amplitude modulation, which although more complex has the purpose of enhancing nonlinear activity from microbubbles at a low MI and canceling out the linear responses from tissue. Contrast imaging with each specific pulse-sequence scheme can be used at very low MIs (<0.2) to assess LVO and myocardial contrast perfusion in real time with excellent spatial resolution. Sonographers should be aware of the variations in pulse-sequence schemes and use them if available whenever contrast is required ( Table 1 ). The advantage, compared with B-mode low-MI harmonic imaging (LVO), is that there is better tissue cancelation and enhanced contrast from microbubbles. However, not all vendors have real-time very low MI imaging software available, and in these settings, low-MI (<0.3) harmonic imaging should be used.
This document provides instructions on how to set up very low MI real-time imaging, and the video examples provide specific examples as well as potential artifacts. The writing group recommends that sonographers who are just beginning to use UCAs, or who do not have very low MI imaging software available, start with the low-MI harmonic imaging methods described in Table 1 . We recognize that experience is a critical factor in performing any aspect of ultrasound imaging, and we recommend to all sites that they work with their local contrast agent representatives to optimize contrast with low-MI imaging techniques and with their specific ultrasound vendors on how to effectively use real-time very low MI imaging software.
II
Update on Contrast Administration Policy and Establishing Intravenous Access
It is recognized that the establishment of IV access remains one of the biggest obstacles to administering UCAs in clinical echocardiography laboratories. Because UCAs are critical to improving the detection of regional wall motion abnormalities and improving the detection of Doppler signals, it is essential that sonographers work with hospital administrations to adopt a contrast program that promotes their use in technically difficult studies. In August 2012, the Intersocietal Accreditation Commission (IAC) officially released the new IAC standards and guidelines for adult echocardiography accreditation. The guidelines require all cardiac ultrasound systems to have instrument settings to enable the optimization of UCAs. The IAC guidelines recommend using UCAs for all studies with suboptimal image quality and require a policy or process to enable alternative imaging for suboptimal studies. Several large clinically active cardiology programs have put in place policies for UCA use that assist sonographers in complying with current IAC guidelines. This update reemphasizes the 2001 statement that the ASE supports IV training for sonographers in hospital and clinic settings. This training requires knowledge of aseptic technique, venous anatomy, appropriate sites of access, risks to patients, and hospital approval to perform the technique. To optimize echocardiographic quality and improve patient care by reducing unnecessary additional procedures, UCAs should be used when indicated, and sonographers deserve full hospital administrative support in achieving this IAC mandate. The Appendix describes the different methods by which hospitals have developed contrast protocols that permit the streamlined use of UCAs while minimally affecting hospital throughput.
III
Update on How and When to Perform Contrast Echocardiography
UCAs should be used whenever suboptimal images exist for the quantification of chamber volumes and ejection fraction and the assessment of regional wall motion (see Table 3 of the ASE consensus statement ). Suboptimal images are defined as the inability to detect two or more contiguous segments in any three of the apical windows. Doppler flow evaluations with UCAs should be performed on rest or stress studies if spectral signals to quantify velocities and pressure gradients were inadequate. Doppler enhancement with UCAs can be done in the same studies in which UCAs were used to improve LVO.
Typical location of artifact | Artifact/problem | Sonographer correction method | Key additional points |
---|---|---|---|
Apex-endocardial border | Swirling | Use real-time very low MI imaging Increase contrast infusion rate ( Video 7 ; available at www.onlinejase.com ) | Lower frame rate prevents apical destruction; also can move focus to near field. |
Apex-myocardium | Reduced contrast | Increase near-field TGC under resting conditions; move focus temporarily to near field ( Video 8 ; available at www.onlinejase.com ) | If resting wall motion is normal, perfusion should be normal, so a defect in this setting is an artifact. |
Basal segment–myocardium | Reduced myocardial contrast | Additional foreshortened apical windows to get basal segments in the near field ( Video 9 ; available at www.onlinejase.com ) | If resting wall motion is normal, perfusion is normal, and therefore there should be no resting contrast defects in the absence of wall motion abnormalities. Use this concept in setting up receiver gain during resting images, because during stress, perfusion alone can be abnormal. |
LV cavity contrast | Inadequate using a continuous infusion | Check IV site to ensure not obstructed; increase infusion rate; ensure contrast is not too dilute and is staying adequately mixed | Could switch to a small bolus. |
LV cavity contrast | Shadowing of basal/mid segments | Slow down infusion or reduce bolus size and flush rate | Infusion (compared with bolus) reduces shadowing problems and allows more rapid correction of the problem. |
Maneuver(s) | Specific intervention/timing | Mechanism |
---|---|---|
Add blood | 10% blood added to 10% air and 80% saline | Produces smaller, more concentrated microbubbles |
Cough, Valsalva maneuver, and abdominal compression | Performed during full RA opacification | Transiently increases RA pressure, creating RA-to-LA pressure gradient |
Femoral vein injection | Performed instead of arm injection | IVC flow is directed to the IAS; SVC flow is directed to the TV |
The writing group recommends that users become familiar with both the bolus techniques and continuous infusion methods described in the Appendix . Bolus injections can result in severe attenuation of the LV cavity, which takes time to resolve. If a bolus is to be used, it is recommended to give it as a 0.5-mL dose of a dilution of Definity (Lantheus Medical Imaging, North Billerica, MA) (one vial in 8.5 mL of saline) or as a 0.3-mL dose of undiluted Optison (GE Healthcare, Little Chalfont, United Kingdom). In each case, the saline flush should be approximately 3 mL over 10 sec. Depending on the indication for contrast agent use, the sonographer should optimize the MI to improve detection of contrast agent in specific areas. Contrast agent administration should be done with either harmonic low-MI imaging or with the very low MI real-time software described in Table 1 , not with fundamental imaging. The Appendix describes the administration techniques used by the different members of the writing group for both bolus injections and continuous infusions. In general, low-MI harmonic imaging requires lowering the MI to <0.3 while in a harmonic imaging mode and the administration of small boluses (as described above) followed by slow saline flushes (3–5 mL over 5–10 sec). The very low MI real-time imaging techniques are inherently tissue cancelation techniques that eliminate or reduce myocardial and valvular signals in the absence of contrast. Brief (three to 10 frames) high-MI “flashes” can be used to clear contrast from the myocardium or from intracardiac masses, to analyze the rate at which contrast replenishes these areas. Normal resting myocardial contrast replenishment should occur within 4 sec, while during stress imaging, the replenishment should occur within 2 sec (see Section V ). The time gain compensation settings should be adjusted under resting conditions so that myocardial and LVO appears even from the base to the apex, which typically requires a slight adjustment upward of the near-field potentiometers (or near-field time gain compensation settings). Specific setups for sonographers in different clinical settings are described below. The ASE’s ContrastZone Web site ( http://www.contrastzone.com ) has additional tips and instructions for using contrast to improve LVO.
LV Ejection Fraction and Regional Wall Motion Assessment
This clinical setting includes both situations in which accurate serial assessments of ejection fraction are required (e.g., chemotherapy) and when visualization of the endocardium is critical (evaluation of chest pain [CP] or during stress echocardiography). In this context, a contrast infusion or slow bolus/flush technique should be used to ensure optimal LVO without shadowing of basal segments or swirling of contrast agent in the apical portion of the LV cavity. Swirling can be avoided by using the real-time very low MI techniques or using a lower scan-line density in the near field with low-MI harmonic imaging. Multicenter studies have emphasized the use of a low MI (<0.3) to achieve full opacification of the apex to optimize the detection of regional wall motion abnormalities and quantify ejection fraction. It is recommended that both regional wall motion analysis and quantification of volumes not be made until full opacification of the left ventricle is achieved without apical swirling or basal segment attenuation. Although perfusion imaging is not an approved indication, the detection of myocardial contrast enhancement after brief high-MI impulses does correlate with myocardial blood flow abnormalities and may improve the detection of subendocardial wall motion abnormalities using the same infusion or slow bolus/flush techniques used to optimize LVO.
Hypertrophic Cardiomyopathy, Apical Variant
The apical variant of hypertrophy associated with hypertrophic cardiomyopathy is present in about 7% of affected patients but may not be detected by routine surface echocardiography, because of incomplete visualization of the apical endocardial border. When apical hypertrophic cardiomyopathy is suspected but not clearly documented or excluded, contrast studies should be performed. If apical hypertrophic cardiomyopathy is present, the characteristic spadelike appearance of the LV cavity, with marked apical myocardial wall thickening, is clearly evident on contrast-enhanced images.
LV Noncompaction
Noncompaction of the myocardium is an uncommon but increasingly recognized abnormality that can lead to heart failure and death. It is due to alterations of myocardial structure with thickened, hypokinetic segments that consist of two layers: a thin, compacted subepicardial myocardium and a thicker, noncompacted subendocardial myocardium. When LV noncompaction is suspected but inadequately visualized with conventional two-dimensional imaging, the characteristic deep intertrabecular recesses of the noncompacted layer may be identified by showing contrast medium–filled intracavitary blood between prominent LV trabeculations. In this setting, it is recommended that one use a harmonic MI setting that is intermediate (i.e., 0.3–0.5) rather than the usual low-MI imaging, to more clearly delineate the trabeculations ( Video 1 ; available at www.onlinejase.com ). Although several different diagnostic criteria are used to diagnose isolated noncompaction, the writing group recommends a noncompacted to compacted ratio of >2:1 when using contrast.
LV Thrombus and Intracardiac Mass Evaluation
LV thrombi are most commonly located in the LV apex. An apical thrombus may be difficult to define clearly, or to exclude, especially if the apex is foreshortened or there is near-field ring-down artifact. The use of UCAs reduces the likelihood of foreshortening of the left ventricle, enabling full visualization of the apex and detection of the telltale “filling defect” sign of a thrombus, which might otherwise be missed. In a recent study of patients at high risk for thrombus because of myocardial infarction or heart failure, contrast echocardiography nearly doubled the sensitivity (61% vs 33%, P < .05) and yielded improved accuracy (92% vs 82%, P < .01) compared with noncontrast echocardiography for the detection of LV thrombi, of which 75% were apical. In that study, contrast echocardiography and cine magnetic resonance imaging closely agreed on the diagnosis of thrombus (κ = 0.79, P < .001), although thrombus prevalence was lower by contrast echocardiography than delayed-enhancement cardiac magnetic resonance ( P < .05). Those thrombi that were detected by delayed-enhancement cardiac magnetic resonance, but not by contrast echocardiography, were more likely to be mural in shape or small in volume ( P < .05). The writing group recommends that ultrasound contrast agent be used to assess for cavitary thrombi whenever the LV apex is not clearly visualized on a noncontrast examination of a patient with severely depressed systolic function.
To differentiate a thrombus from an intracardiac tumor, real-time very low MI perfusion imaging with high-MI flash should be used if available. Thrombi are avascular and show no contrast enhancement after a high-MI flash impulse, as opposed to tumors, which may be either poorly (benign stromal tumors, such as myxoma) or highly (malignant tumors) vascularized and will demonstrate proportional degrees of perfusion by flash replenishment real-time very low MI imaging ( Videos 2-4 ; available at www.onlinejase.com ). If real-time very low MI software is not available, low-MI (<0.3) harmonic imaging can be deployed to visualize whether contrast enhancement is occurring within the mass and aid in the differentiation of cardiac masses.
LV Aneurysm versus Pseudoaneurysm
LV aneurysm, an often asymptomatic complication of a prior myocardial infarction, is the most common LV apical abnormality. It is characterized by thin walls and a dilated apex, which may be akinetic or dyskinetic. These findings are usually easily visualized with echocardiographic imaging. However, if the apex is foreshortened and not completely visualized, an apical aneurysm may go undetected. UCAs can aid in visualizing apical wall motion abnormalities, but care must still be taken to minimize foreshortening. In addition, associated abnormalities (such as LV apical thrombus) may not be visible until a UCA is used. The use of UCAs by a sonographer to detect an apical aneurysm or a thrombus also requires the use of both the parasternal and apical windows to delineate the extent of the abnormality and avoid foreshortening. Similarly, a pseudoaneurysm can be distinguished from an aneurysm using contrast to demonstrate a narrow neck and systolic filling of the pseudoaneurysm sac. ( Video 5 ; available at www.onlinejase.com ) demonstrates examples of contrast filling an apical and inferior pseudoaneurysms in systole. Both cases were confirmed at surgery.
Other Less Common Apical Abnormalities
The characteristic layering of thrombus and necrotic material at one or both of the left and right ventricular apices, without underlying wall motion abnormality and preserved perfusion with contrast, can be seen in endomyocardial fibrosis ( Figure 2 ).
Stress-induced (takotsubo) cardiomyopathy most frequently affects the mid to apical region of the left ventricle, in a distribution that is not characteristic of coronary artery disease ( Figure 3 ). The classic appearance of the left ventricle in this disorder is more fully characterized and appreciated when the left ventricle is opacified with contrast agent. Moreover, delayed, but present, myocardial perfusion in the affected segments may help differentiate stress-induced cardiomyopathy from epicardial coronary disease. This is a critical diagnostic finding, especially in postmenopausal women presenting with acute coronary syndromes, who not infrequently have associated renal dysfunction or other relative contraindications for angiography.
Emergency Department CP Evaluation
Acute CP is one of the most common presenting symptoms to an emergency department. The causes of this symptom may range from benign musculoskeletal problems to life-threatening conditions such as acute myocardial infarction (AMI), aortic dissection, or acute pulmonary embolism. Of these “serious” causes of CP, AMI occurs the most frequently. Making an early diagnosis of AMI, however, can be difficult, because the medical history, physical examination, electrocardiography, and chest radiography all have poor sensitivity. Serum cardiac biomarkers are currently the main methods used to determine if a patient is presenting with an AMI, but they are not released into the serum until hours after the onset of symptoms. Echocardiography can be used to detect a patient with an acute coronary syndrome because even after a brief coronary occlusion (5–15 min), regional systolic function is reduced. More important, the absence of a segmental wall thickening abnormality can exclude an ischemic cause of CP. Normal regional function identifies a low-risk population with a 24-hour adverse event rate of only 0.4%. Patients with abnormal regional function are sixfold more likely to have early events compared with those with normal function.
Therefore, for sonographers, the use of UCAs in patients with CP is critical to attain optimal sensitivity for detecting even small focal wall thickening abnormalities. The use of UCAs has been shown to significantly improve the detection of a new segmental abnormality. It is the sonographer’s role to quickly evaluate all LV wall segments in patients with suspected acute coronary syndromes; however, this must be done with the mind-set that if two contiguous wall segments cannot be visualized, wall motion must be further evaluated with the use of UCAs. An example of a lateral wall motion abnormality detected only with very low MI contrast imaging is demonstrated in ( Video 6 ; available at www.onlinejase.com ). The use of real-time very low MI imaging in patients with CP adds additional diagnostic and prognostic information by simultaneously providing perfusion information. If complete replenishment of contrast is observed within 4 sec in a segment with abnormal regional wall motion (i.e., normal perfusion), this identifies a patient at intermediate risk for cardiac events compared with a high-risk situation in which both a regional perfusion abnormality (delayed replenishment of contrast) and a wall motion abnormality exist.
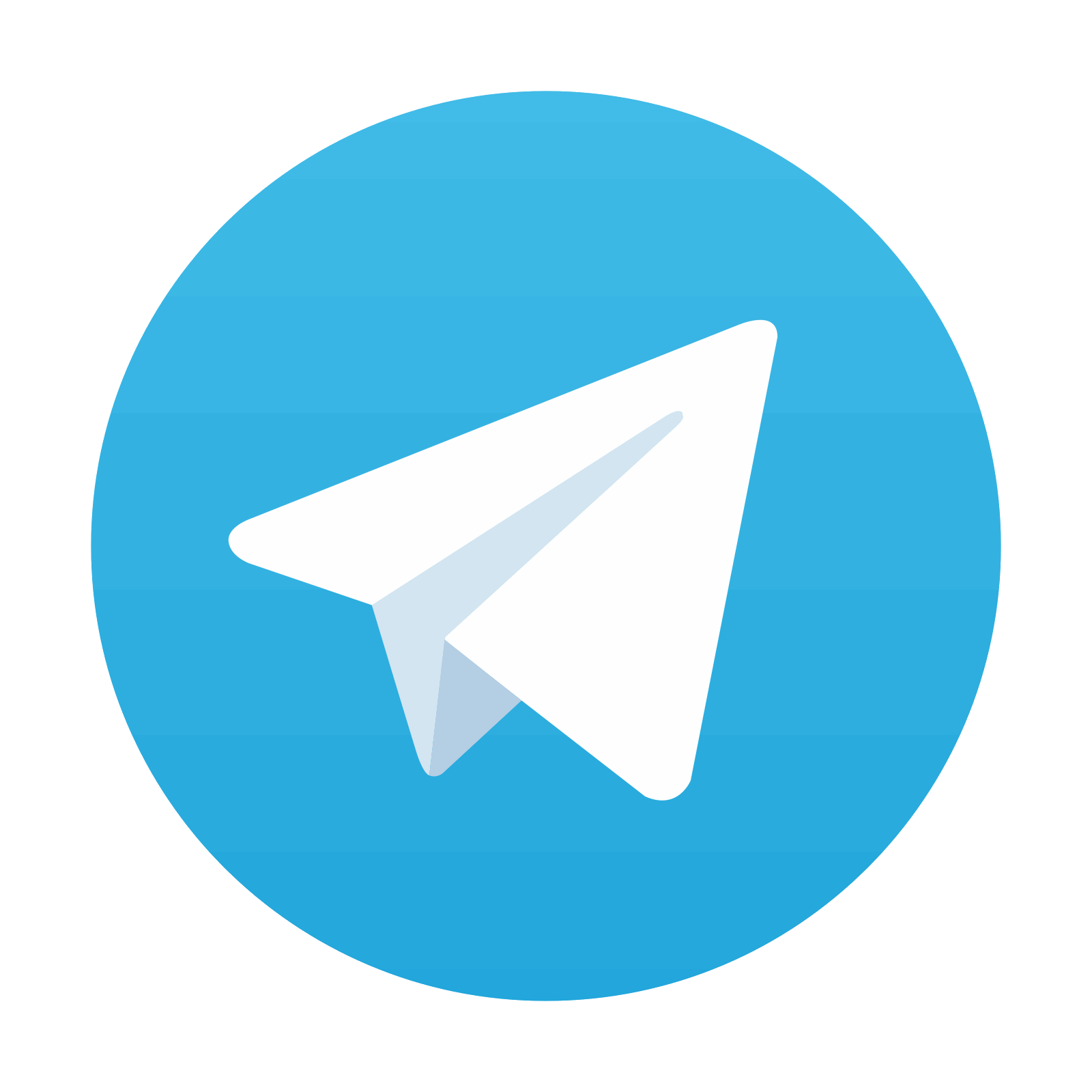
Stay updated, free articles. Join our Telegram channel

Full access? Get Clinical Tree
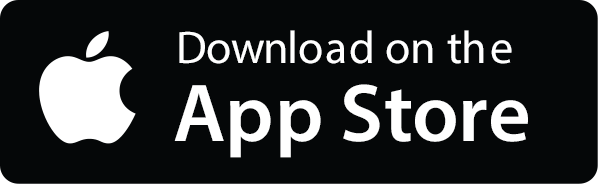
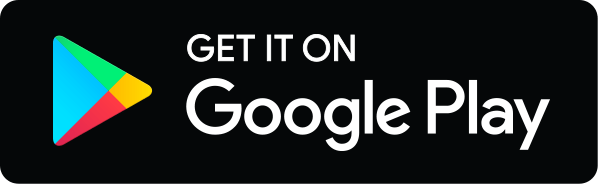