Evidence of the involvement of the cardiovascular system in patients with COVID-19 is increasing. The evaluation of the subclinical cardiac involvement is crucial for risk stratification at admission, and left ventricular global longitudinal strain (LVGLS) may be useful for this purpose. A total of 87 consecutive patients admitted to the COVID Center were enrolled from December 2020 to April 2021. A complete echocardiography examination was performed within 72 hours from admission. The main outcome was the need for mechanical ventilation by way of orotracheal intubation (OTI) and mortality, and the secondary outcome was the worsening of the respiratory function during hospitalization, interpreted as a decrease of the ratio between the partial pressure of oxygen and the fraction of inspired oxygen (P/F) <100. Of 87 patients, 14 had severe disease leading to OTI or death, whereas 24 had a P/F <100. LVGLS was significantly impaired in patients with severe disease. After adjustment for risk factors, by considering LVGLS as continuous variable, the latter remained significantly associated with severe acute respiratory distress syndrome (P/F <100) (hazard ratio [HR] 1.48, 95% confidence interval [CI] 1.18 to 1.88, p = 0.001) and OTI/death (HR 1.63, 95% CI 1.13 to 2.38, p = 0.012). When using an LVGLS cutoff of −16.1%, LVGLS ≥ −16.1% was independently associated with a higher risk of severe acute respiratory distress syndrome (HR 4.0, 95% CI 1.4 to 11.1, p= 0.008) and OTI/death (HR 7.3, 95% CI 1.6 to 34.1, p = 0.024). LVGLS can detect high-risk patients at the admission, which can help to guide in starting early treatment of the admitted patients.
COVID-19 is a pandemic viral infection leading to SARS-CoV-2. Although this respiratory syndrome is the most important cause of death, increasing evidence indicates that the cardiovascular system is affected by this disease. , Recently, the echographic measurement of left ventricle (LV) deformation by global longitudinal strain (GLS) has been introduced as a novel marker of cardiac dysfunction. It evaluates myocardial deformation and is less influenced by loading conditions compared with ejection fraction (EF). Accumulating literature on multimodal cardiac imaging highlights the LV GLS (LVGLS) impact on the prognosis of several clinical conditions. Moreover, LVGLS can detect initial and subclinical ventricle impairment, earlier than conventional echocardiographic parameters. The evaluation of the subclinical cardiac involvement in patients with COVID-19 may be crucial and LVGLS may be useful for this purpose. Our study investigates whether LVGLS was a predictor of the decrease of respiratory function, the need for mechanical ventilation, and mortality in patients admitted to the hospital with COVID-19–related infection.
Methods
A single-center, prospective study was performed at the University Hospital of Verona, Italy. We enrolled a total of 87 consecutive patients admitted to the COVID Center Unit (Internal Medicine and Infectious Disease wards) from December 2020 to April 2021. All admitted patients received bedside echocardiography within 72 hours from admission to the COVID Center Unit. The study was approved by the local Ethics Committee. Inclusion criteria were: age ≥18 years; hospitalization with a confirmed diagnosis of COVID-19; EF ≥50%. Exclusion criteria were: history of ischemic cardiomyopathy; the presence of LV segmental wall-motion abnormalities; history of heart failure; severe valvular disease; atrial fibrillation; pulmonary hypertension, chronic obstructive pulmonary disease. Upon admission, complete bloodwork, including the markers presented in this study, was performed. A database including demographic characteristics, comorbidities, laboratory exams, and outcomes was independently reviewed by a medical team using the electronic database. The respiratory impairment was assessed using the ratio between the partial pressure of oxygen and the fraction of inspired oxygen (P/F) obtained by arterial blood gas analysis.
Parasternal axis, apical views, and subxiphoid view were obtained using a GE Vivid T8 ultrasound system (GE Healthcare, Arlington Heights, Illinois), (GLS normal values −18.2% to −21.2%). A digital loop, electrocardiographic gated, was acquired from 2 to 3, and 4 chamber views. All images were stored and analyzed at a later time. LVGLS was calculated by averaging the values obtained in the apical 2 to 3 and 4 chamber views (18 segments). LV endocardial borders were automatically traced at the end of the diastole, subsequently, the software tracked the endocardial layer throughout the cardiac cycle. When necessary, the operator manually adjusted the endocardial border. A segment could be excluded by the operator if it was judged not well traced ( Figure 1 ). All measurements were made blinded to other laboratory and clinical data, including patient outcomes.
Two different outcomes were considered in our study: the main outcome was the need of orotracheal intubation (OTI) and mortality, whatever came first; the secondary outcome was the development of severe acute respiratory distress syndrome (ARDS) (P/F <100 mm Hg) during hospitalization.
Continuous variables are presented as mean ± SD or median with interquartile range based on data distribution. Categorical variables are expressed as percentages. One-way analysis of Variance or Kruskal–Wallis were used to compare continuous variables according to the data distribution pattern. Logistic and Cox regression analyses were performed to determine if any anamnestic, clinical, or imaging variable (age, gender, hypertension, diabetes, chronic kidney disease, d-dimer, ferritin, ratio between early mitral inflow velocity and mitral annular early diastolic velocity (E/E’), and LVGLS) could be independently associated with the dependent variable (end points). The variable selection was made through sequential replacement (a stepwise method), which consists of a combination of backward and forward techniques. If the p value was <0.05 or >0.1, the covariates were respectively included and excluded from the regression model. The area under the receiver operating characteristic (ROC) curves were built to assess the performance of LVGLS (alone and together with the other dependent variables included in the model) for predicting the study end points. Furthermore, we tried to identify the optimal cut-off point of LVGLS for the prediction of the outcomes, trying to optimize sensibility and specificity in the C-statistics analysis. IBM SPSS Statistics for Windows, Version 22.0. (IBM Corp. Released 2013, Armonk, New York) was used for all data analysis. All tests were 2-sided, and p <0.05 were considered statistically significant.
Results
A total of 87 consecutive patients were enrolled in our study. Baseline anthropometric, clinical, biochemical parameters, and echocardiography data are listed in Tables 1 and 2 . Regarding specific medical therapy for COVID-19, 90% of subjects were treated with steroids, 18.8% with remdesivir, 24.4% received antibiotics, and all patients were treated with low molecular weight heparin. Patients with lower LVGLS had higher N-terminal pro brain natriuretic peptide (r = 0.58, p <0.001), no correlations were found between LVGLS and other biochemical and imaging parameters. When patients were divided into 2 subgroups according to the end points of the study: OTI or death (n = 14; Table 1 ); development of severe ARDS (P/F <100 mm Hg) (n = 24; Table 2 ), LVGLS was statistically significant impaired in both outcomes. ROC curves were elaborated for both end points to obtain the area under the curve, either considering LVGLS alone or on top of the other covariates after the regression models. In patients with the P/F lower than 100, LVGLS alone predicted the outcome with 78% accuracy (95% CI 0.66 to 0.88). The addition of LVGLS into the model with clinical and imaging predictors resulted in a significant increase in the C-statistic (from 0.64 to 0.85, p <0.05) ( Figure 2 ). When considering the OTI/death end point, LVGLS alone predicted the outcome with 74% accuracy (95% CI 0.68 to 0.87). The addition of LVGLS into the model with clinical and imaging predictors resulted in a nonsignificant increase in the C-statistic (from 0.73 to 0.93; p >0.05) ( Figure 2 ). In the Cox regression analyses, considering LVGLS as a continuous variable, this remained independently predictive of severe ARDS (P/F <100) (HR 1.48, 95% CI 1.18 to 1.88, p = 0.001) and OTI/death (HR 1.63, 95% CI 1.13 to 2.38, p = 0.012). When using an LVGLS cutoff of −16.1% (sensibility 86.5%, specificity 58.5% by the ROC curve), LVGLS ≥ −16.1% was independently associated with a higher risk of severe ARDS (HR 4.0, 95% CI 1.4 to 11.1, p = 0.008) and OTI/death (HR 7.3, 95% CI 1.6 to 34.1, p = 0.024) ( Figure 3 ). Ferritin was a significant predictor of both outcomes (HR 1.001, 95% CI 1.000 to 1.002, p = 0.029), whereas the E/E′ ratio was significant only in the OTI/death outcome (HR 1.62, 95% CI 1.12 to 2.36, p = 0.014).
OTI/death | OTI/death | ||
---|---|---|---|
Variable | Yes (n°14) | No (n°73) | p value |
Age (years) | 75.0 [61.5; 83.5] | 63.0 [53.5; 73.5] | 0.070 |
Male | 79% | 70% | 0.068 |
Body mass index (kg/m 2 ) | 24.9 [23.4; 27.7] | 26.5 [24.9; 29.2] | 0.120 |
Hypertension ⁎ | 50% | 40% | 0.480 |
Dyslipidemia † | 10% | 23% | 0.176 |
Chronic kidney disease | 14% | 12% | 0.842 |
Diabetes mellitus | 2% | 16% | 0.105 |
ACE inhibitors/angiotensin receptor blocker | 36% | 35% | 0.994 |
Beta-blockers | 43% | 21% | 0.075 |
Systolic blood pressure (mm Hg) | 130.0 [103.5; 149.5] | 120.0 [115; 140] | 0.629 |
Diastolic blood pressure (mm Hg) | 73.0 [61.2; 87.5] | 75.0 [65; 80] | 0.979 |
Heart rate (bpm) | 88 [75; 90] | 76 [70; 88] | 0.174 |
C-reactive protein (mg/dL) | 147 [55; 160] | 62 [26; 133] | 0.039 |
Procalcitonin (ng/mL) | 0.24 [0.19; 0.42] | 0.08 [0.05; 0.27] | 0.014 |
D-dimer (µg/L) | 1425 [831; 2017] | 1121 [600; 2156] | 0.448 |
Ferritin (µg/L) | 798 [522; 3808] | 872 [508; 1427] | 0.574 |
Lymphocytes (mm 3 ) | 700 [422; 1330] | 815 [600; 1175] | 0.576 |
Prothrombin time | 1.06 [1.01; 1.15] | 1.08 [1.00; 1.19] | 0.924 |
Creatine-kinase (U/L) | 85 [62; 147] | 70 [42; 160] | 0.361 |
Lactic dehydrogenase (U/L) | 394 [341; 545] | 304 [241; 379] | 0.011 |
Troponin (ng/L) | 14.4 [9; 26] | 13 [7; 16] | 0.452 |
N-terminal pro brain natriuretic peptide (pg/ml) | 1246 [91; 6774] | 351 [135; 795] | 0.047 |
Glomerular filtration rate (ml/min) | 61 [51; 82] | 77 [55; 100] | 0.036 |
Alanine aminotransferase (U/L) | 28 [16; 48] | 30 [19; 50] | 0.683 |
Cardiac mass indexed (g/m 2 ) | 119.5 [85.2; 126.2] | 99.5 [73.1; 118.1] | 0.099 |
Left ventricular end diastolic volume (mL) | 84.2 [62.4; 98.0] | 67.5 [55.5; 85.2] | 0.021 |
Ejection fraction (%) | 60.0 [54.5; 65] | 62.0 [57; 69] | 0.163 |
Left atrial volume indexed (mL/m 2 ) | 23.4 [15.7; 32.0] | 20.5 [15.3; 27.5] | 0.285 |
Right atrial volume (mL) | 28.0 [23.0; 37.2] | 31.0 [22.0; 42.1] | 0.753 |
E/A | 0.98 [0.85; 1.39] | 0.91 [0.75; 1.21] | 0.306 |
E/E’ | 8.7 [7.1; 12.3] | 6.3 [5.1; 6.1] | 0.002 |
Right ventricular diameter (mm) | 33.5 [31.7; 39.0] | 24.0 [31.0; 38.0] | 0.660 |
TAPSE (mm) | 24.5 [19.0; 30.0] | 25.0 [21.0; 28.5] | 0.849 |
Systolic pulmonary artery pressure (mm Hg) | 21.5 [15.4; 35.0] | 24.0 [10.0; 30.0] | 0.624 |
Left ventricular global longitudinal strain (%) | -14.5 [-13.4; -16.3] | -16.6 [-14.4; -18.5] | 0.023 |
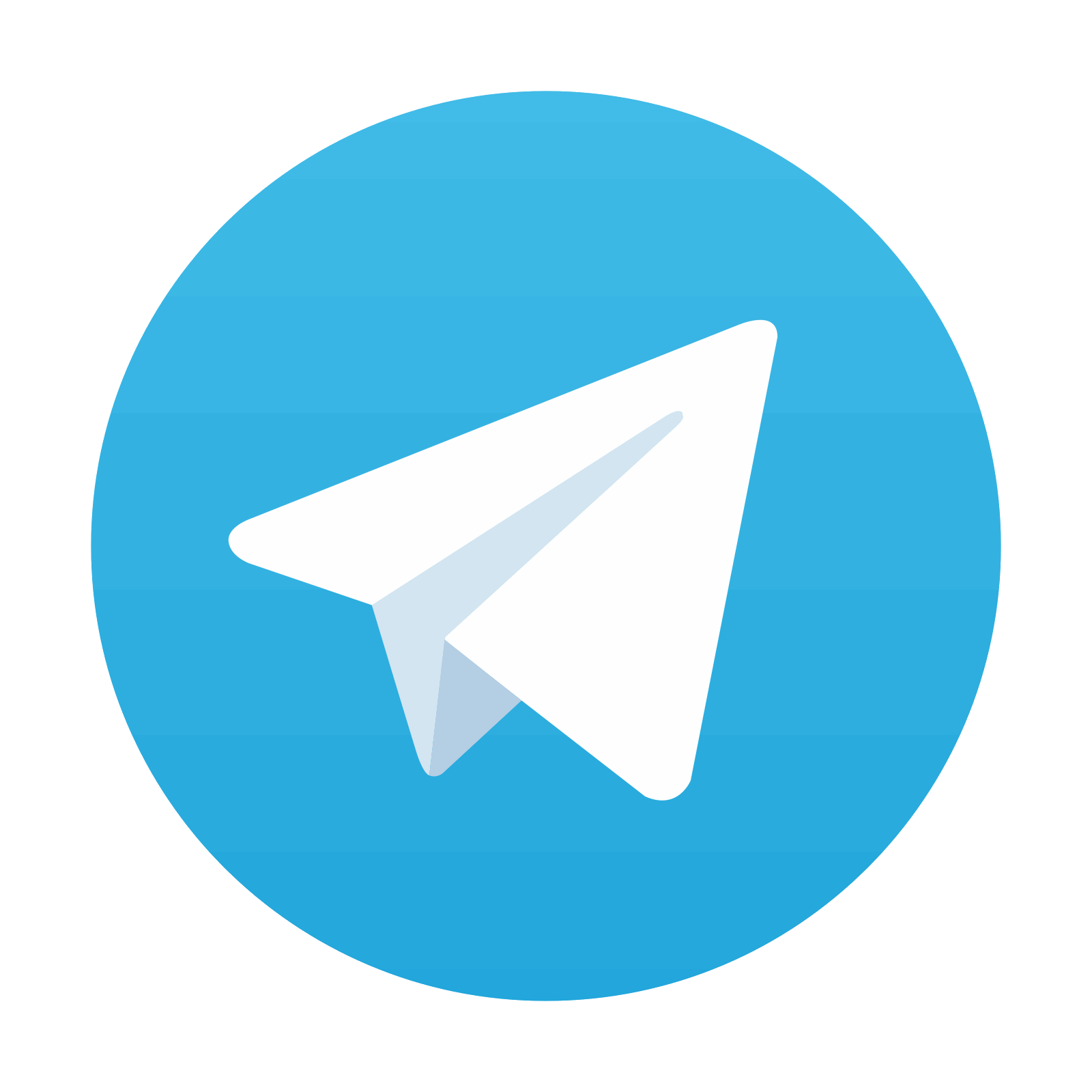
Stay updated, free articles. Join our Telegram channel

Full access? Get Clinical Tree
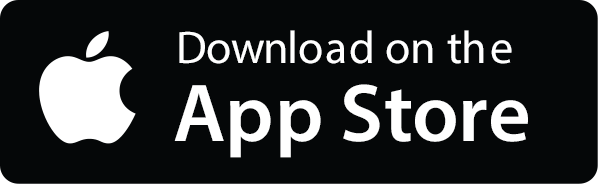
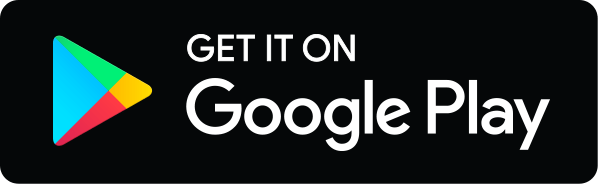
