Esophageal carcinomas remain a leading cause of cancer-related death worldwide, and the prognosis remains poor with 5-year overall survival of only 17%.1 In the mid-1990s, esophageal adenocarcinoma (EAC) overtook squamous cell carcinoma (ESCC) as the most common esophageal cancer in the United States.2,3 Risk factors include smoking, obesity, and Barrett metaplasia secondary to gastroesophageal reflux, whereas infection with Helicobacter pylori appears to be protective.4
The development of EAC is a multistep process involving numerous genetic changes. According to Hanahan and Weinberg, tumorigenesis involves six hallmark characteristics including self-sufficient growth, insensitivity to antigrowth signals, evasion of apoptosis, sustained angiogenesis, a limitless replicative potential, and tissue invasion and metastasis.5 The genetic changes involved in the metaplasia–dysplasia–adenocarcinoma sequence of EAC will be presented in this review. Whether further characterization of the molecular events leading to EAC will provide new targets for diagnosis, prevention, or treatment remains to be established.
During the progression from dysplastic Barrett esophagus to EAC a number of molecular events have been identified (Fig. 170-1),6 including altered expression of tumor suppressor genes such as p53 and p16, cyclin-dependent kinase inhibitor 2 A (CDKN2 A), and involvement of oncogenes including myc, EGFR, HER2/neu, and c-Met.7–10 Cell cycle markers including polyploidy and aneuploidy also have been identified early in the dysplasia–adenocarcinoma sequence.11–13
Figure 170-1
Genetic changes involved in the progression from Barrett metaplasia to esophageal adenocarcinoma. Many of the early changes persist as the lesions progress to dysplasia and adenocarcinoma. With permission from Lin J, Beer DG. Molecular biology of upper gastrointestinal malignancies. Semin Oncol. 2004;31(6):476–486.

With the development of high-throughput microarray technologies and concomitant analytic methodologies,14 investigators have sought to identify genes,15,16 proteins, or important regulatory elements such as microRNAs17 or protein kinases18 among which patterns of expression either might distinguish between esophageal dysplasia and carcinoma, or might identify tumors with more likelihood to respond to specific treatment regimens. At the chromosomal level, alterations of gene copy number, such as by gene amplification or deletion, are being characterized in ever-increasing number and detail.19 Such copy-number alterations may be associated with EAC progression and advancing stage.20 Investigators also have catalogued copy-neutral changes, particularly loss of heterozygosity, associated with EAC, using single nuclear polymorphism (SNP) microarrays.21–23 Genome sequencing techniques likely will provide further detailed characterization of gene rearrangements that are important in the pathophysiology of esophageal cancers, as has been demonstrated in a number of solid-tumor malignancies.24
As more studies are published that identify associations between genetic changes and the progression to EAC, it is readily apparent that there is wide variability among such alterations between one individual and another. Such variability may limit the diagnostic and prognostic utility for any one putative marker to only a small percentage of tumors. It also appears that there can be considerable genetic heterogeneity even within a tumor, reflecting the evolutionary nature of neoplastic progression. Measurement of such clonal diversity appears to be a strong predictor of the progression from Barrett esophagus to EAC, independent of the actual genetic events, such as loss of heterozygosity, frameshift or deletion mutations, or abnormal changes in promoter methylation status, that comprise this heterogeneity.25,26
Clinical trials evaluating the efficacy of preoperative chemotherapy with concurrent radiation therapy consistently have demonstrated pathologic complete response (ypT0N0 Mx) of approximately 20% to 30% among patients who undergo subsequent esophageal resection.27–30 Pathologic complete response has been associated as a significant determinant for long-term disease-free and overall survival.31 Conversely for patients who have either partial or no tumor response to preoperative chemoradiation, there does not appear to be a survival benefit but rather an overall detriment. Thus identification of predictive markers for response to preoperative therapy potentially can direct nonresponders to alternative treatment regimens while limiting the toxicity of first-line chemoradiation strategies and subsequent esophageal resection.
Molecular markers may be developed for a number of clinical applications, and can be categorized broadly as diagnostic, prognostic, and predictive.32 For patients with esophageal carcinomas, most putative biomarkers remain unproven for broad application in terms of analytic validity (accuracy and reliability of the measured analyte), clinical validity (accuracy and reliability of detecting or predicting a clinically defined disorder) and clinical utility (i.e., providing added value for patient management decision making).33 Several small cohort studies have identified possible markers for response to preoperative chemotherapy with or without radiation therapy, although large-scale validation studies remain to be completed. 34 Such markers include genes associated with chemotherapy metabolism such as thymidylate synthase (TYMS), thymidine phosphorylase (TP), dihydropyrimidine dehydrogenase (DPD), and methylenetetrahydrofolate reductase (MTHFR), all of which participate in the metabolism of 5-fluorouracil,35,36 or glutathione S-transferase π (GSTP) and the excision cross-complementing gene 1 (ERCC1), both of which have been associated with response to platinum-based chemotherapy.37,38
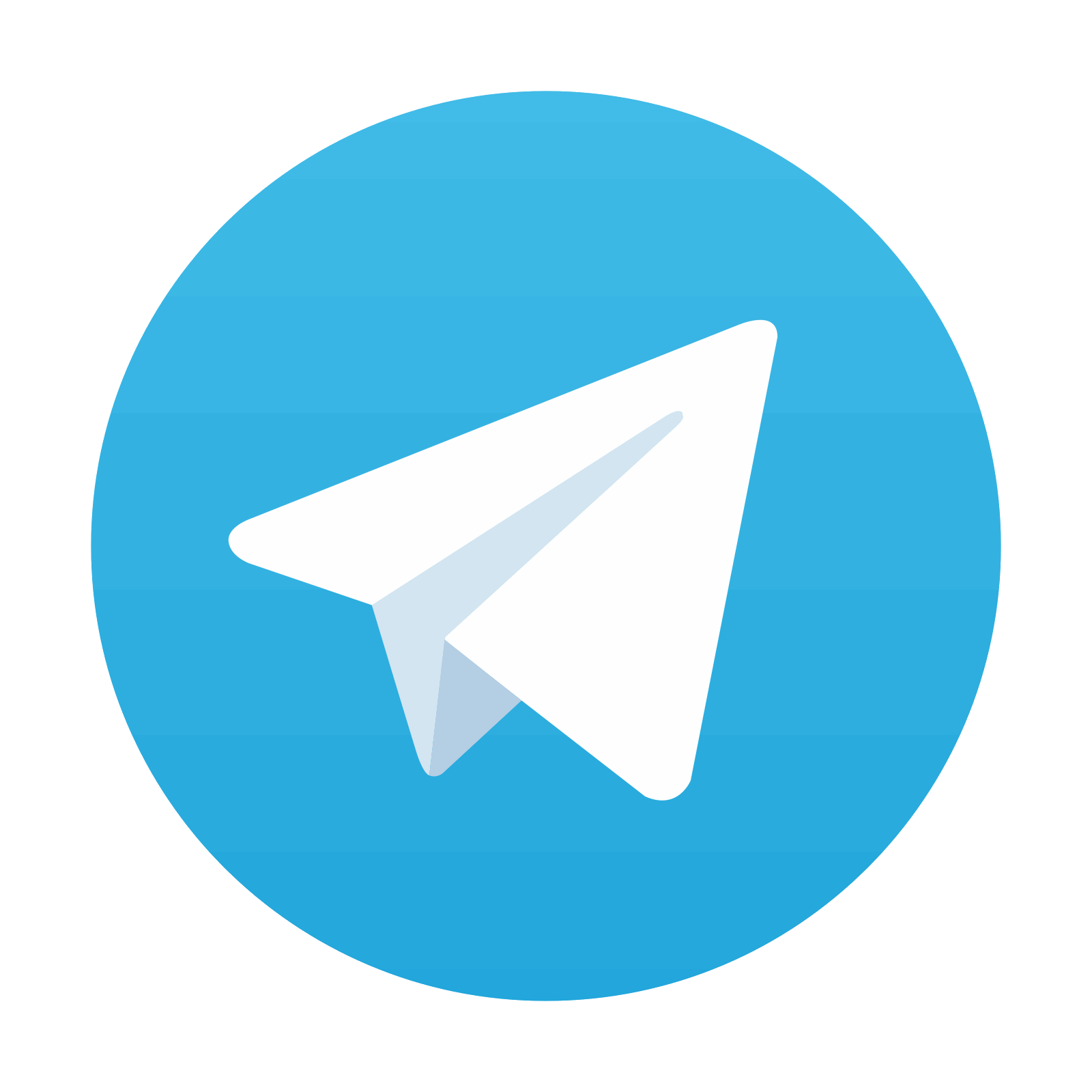
Stay updated, free articles. Join our Telegram channel

Full access? Get Clinical Tree
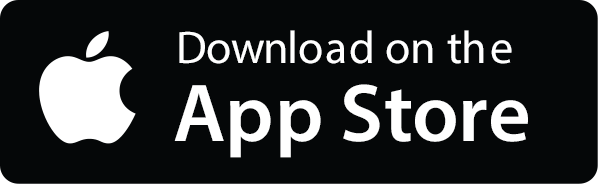
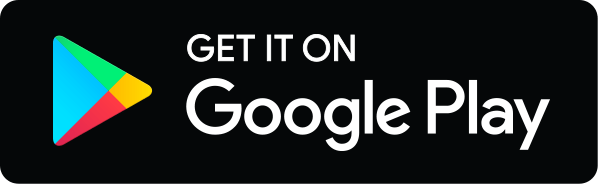