Gene/chromosome
MOI
Nr. Fam
Type AF
Age
Ethnicity
HR
(A)symp
TCM
QTc (ms)
Mechanism
I. No locus and gene identified
FAF 1-3 [8]
AD
3
PAF
38–51
?
High
symp
n = 2
Normal
?
FAF 4 [8]
AD
1
PAF
37
?
Slow
asymp
No
↑ in 2
?
II. Locus identified
10q22-q24 [15]
FAF 1
AD
1
CAF, (PAF)
18
Cauc
na
asymp
No
na
?
FAF 2-3
AD
2
CAF
2–46
Cauc
na
asymp
No
na
?
6q14-q16 [19]
AD
1
PAF, CAF
21–72
Cauc
na
na
Noa
na
?
III. Gene identified
KCNQ1 [23]
AD
1CAF
>5
Chin
na
na
na
50 % ↑
IKs ↑
KCNQ1 [27]
AD
1
PAF, CAF
30
Cauc
na
symp
No
Normal
IKs ↑
KCNE2 [28]
AD
2
PAF
>40
Chin
High
symp
No
Normal
IKs ↑
KCNE5 [35]
?
1b
CAF
59
Cauc
na
na
No
Normal
IKs ↓
KCNJ2 [30]
AD
1
PAF, CAF
50–58
Chin
63–118
symp
No
Normal
IK1 ↑
KCNA5 [32]
AD
1
PAF
30–40
?
na
symp
No
na
IKur ↓
KCNA5 [33]
?
1b
PAF, CAF
23–52
Chin
na
na
No
na
IKur ↓
KCNA5 [34]
AD
1
PAF
18–48
Cauc
na
symp
No
na
IKur ↓
SCN5A [36]c
AD
1
PAF/AFL
12–20
Cauc
na
symp
No
Normal
Excitability ↓
SCN5A [39]
AD
1
PAF
41
Cauc
na
symp
No
Normal
Excitability ↓
SCN5A [40]
AD
1
PAF, CAF
26–51
Jap
68–88
symp
No
Normal
Excitability ↓
SCN1B [41]
?
1b
PAF
35–58
Multi
na
na
No
Normal
Excitability ↓
SCN2B [41]
?
1b
PAF
35–58
Multi
na
na
No
Normal
Excitability ↓
SCN3B [42]
?
1b
PAF, CAF
30
Cauc
61–86
na
No
na
Excitability ↓
NUP155 [43]d
AR
1
CAF
Young
Uru
High
symp
Some
Normal
?
GJA5 [45]
?
1b
PAF
41
?
na
symp
na
na
Dispersed conduction
GJA5 [46]
AD
1b
PAF, CAF
45
Chin
73
na
na
na
Dispersed conduction
GJA5 [47]
AD
1b
PAF, CAF
45
Chin
73
na
na
na
Dispersed conduction
NPPA [48]
AD
1
PAF, CAF
40
Cauc
na
symp
n = 5
na
?
ANK2 [49]
?
2
PAF
11–79
?
45–88
na
No
Prolonged
?
Finally, Ravn et al. [35] sequenced the KCNE5 gene in a cohort of 158 patients with AF. In one individual, without a familial history of AF, they found a missense mutation (p.L65F) in the KCNE5 gene leading to a gain of function of IKs.
Sodium Channel Mutations
Besides changes in atrial action potential duration, such as caused by the above mentioned mutations in atrial potassium channels, also alterations in atrial conduction may lead to predisposition to AF.
Laitinen-Forsblom et al. [36] identified a mutation in the SCN5A gene in a large Finnish family with cardiac conduction defects and atrial arrhythmias (Table 35.1). Six of 44 family members were affected, of which five had a pacemaker implanted due to conduction defects and/or bradycardia before the age of 20. Five of these six subjects suffered from various atrial arrhythmias including paroxysmal AF and atrial flutter. Three suffered from stroke between the age of 20–30 years. None had symptoms before their teens. All six affected individuals had a normal left ventricular ejection fraction although the left ventricle and right ventricle were enlarged in one and three individuals, respectively. A mutation (D1275N) in the SCN5A gene was found in the six affected individuals as well as in two young asymptomatic family members. One of these two carriers had an abnormally wide QRS complex (116 ms). The other was only 12 years old at the time of analysis. The p.D1275N mutation has also been reported in a family with atrial standstill [37] (see below) and in different families with dilated cardiomyopathy [16, 17]. Although atrial standstill due to the p.D1275N mutation has been demonstrated in this Dutch family, it occurred only in combination with polymorphisms in the promoter and untranslated region of the GJA5 gene, encoding connexin 40 [37]. Although p.D1275N did not seem to cause serious channel dysfunction when studied in heterologous expression systems, Watanabe et al. [38] found in a mouse model that the p.D1275N mutation produced extensive channel dysfunction, and a cardiomyopathy phenotype. It is currently unknown why this mutation expresses these different phenotypes and whether the phenotype described in the Finnish family may be considered to be true lone AF.
Ellinor et al. [39] screened the SCN5A gene in a cohort of 57 probands with a familial history AF. They found a mutation (p.N1986K) in one family (two affected members available for analysis) with AF, leading to a loss of function of the sodium channel.
Makiyama et al. [40] studied a Japanese family with autosomal dominant segregated lone AF. Six out of 14 family members had AF; one additional family member had premature atrial beats, but no AF. They found a missense mutation (p.M1875T) in the SCN5A gene, again leading to a loss of function of the sodium channel.
Watanabe et al. [41] screened an AF cohort for mutations in the sodium channel β-subunit genes SCN1B, SCN2B, SCN3B, and SCN4B. A cohort of 480 individuals with AF, including 118 with lone AF and 362 patients with AF and cardiovascular disease was sequenced. They discovered two mutations in SCN1B (p.R85H, p.D153N), and two mutations in SCN2B (p.R28Q, p.R28W). In three of four mutation carriers, the ECGs showed saddleback-type ST-segment elevation in the right precordial leads. Expression studies demonstrated that the mutant SCN1B and SCN2B reduced the sodium current.
In Denmark, Olesen et al. [42] studied 192 unrelated lone AF patients, and sequenced the entire coding sequence and splice junctions of SCN3B and SCN4B. Three non-synonymous mutations p.R6K, p.L10P, and p.M161T, were detected in the SCN3B gene. Electrophysiological studies revealed that all mutations caused a reduction in sodium channel current.
Nucleoporin Mutation
Nucleoporin, encoded by the NUP155 gene, is a major part of the nuclear pore complex [43]. The nuclear pore complex mediates bidirectional transport of mRNAs and proteins between the nucleus and the cytoplasm, potentially regulating the expression of important atrial genes, and subsequently increases the susceptibility of AF. Oberti et al. [44] identified a locus on chromosome 5p13 in autosomal recessively inherited neonatal AF. The affected children showed a rapidly progressive disease: AF with early onset at the fetal stage, neonatal sudden death, ventricular arrhythmias and cardiomyopathy (Table 35.1). The heterozygous parents, both without AF, could be identified by a broad P wave on the electrocardiogram. In 2008, the investigators were able to find the causative homozygous mutation (p.R391H) in the NUP155 gene. These findings provide a novel non-ion-channel mechanism for the pathogenesis of AF, however the exact molecular mechanism linking the nuclear pore complex to AF is still unknown. Whether this disease is caused primarily by AF or, more likely, by an underlying cardiomyopathy remains to be determined.
Gap Junction Protein Mutations
The cardiac gap-junction protein connexin 40, encoded by the GJA5 gene, is expressed selectively in the atria and coordinate the electrical activation of the atrial myocardium. Gollob et al. [45] sequenced the GJA5 gene, encoding connexin 40, in cardiac tissue and lymphocytes in 15 unrelated patients with lone AF. In three patients a novel heterozygous missense mutation was identified in cardiac tissue alone (somatic mutation). However, in one patient the mutation (p.T286G) was present in both cardiac tissue and lymphocytes (germ-line mutation). This indicates that somatic mutations may underlie AF, but inheritable germ-line GJA5 mutations may also occur. Analysis of the expression of the mutant proteins revealed impaired intracellular transport or reduced intercellular electrical coupling, which may result in anisotropic atrial conduction, promoting reentry. Yang et al. [46] sequenced the coding region of the GJA5 gene in 126 Chinese unrelated probands with familial AF. A heterozygous mutation, c.145C > T (p.Q49X) was detected in one proband. This nonsense mutation was present in all relatives with AF of the carrier.
The same group published another report on 218 Chinese unrelated probands [47]. It is unknown whether this study includes the same patients as the other report [46]. Three heterozygous missense GJA5 mutations, p.V85I, p.L221I, and p.L229M, were found in three of 218 AF families, and co-segregated with AF. These results indicate that in some patients lone AF may have a genetic basis confined to the diseased myocardial tissue.
Atrial Natriuretic Peptide Mutation
Atrial natriuretic peptide, encoded by the NPPA gene, is a well-known circulating hormone regulating intravascular blood volume by stimulating natriuresis, diuresis, and vasodilatation. In addition, via cGMP signaling pathways, atrial natriuretic peptide has been shown to modulate ion channel currents in cardiac myocytes leading to shortening of the atrial refractory period. Also anti-apoptotic effects of atrial natriuretic peptide have been described.
Hodgson-Zingman et al. [48] described a family of European ancestry with autosomal dominant AF. Eleven of 21 family members had AF, five of whom presented with a tachycardiomyopathy. In all affected family members a heterozygous two-basepair deletion (c.456-457delAA) in exon 3 of the NPPA gene was found. This frameshift mutation leads to an extension of the reading frame, translating to a mutant protein that includes the normal atrial natriuretic peptide plus a carboxyl terminus of 12 residues. In all heterozygous affected family members increased concentrations of mutant atrial natriuretic peptide were found. The exact mechanism linking the frameshift mutation in NPPA to the phenotype AF needs to be elucidated.
Ankyrin-B Genetic Variants
Membrane adapter protein ankyrin-B, encoded by the ANK2 gene, is expressed in atrial cardiomyocytes, ventricular cardiomyocytes, and cardiac Purkinje conduction fibers. Ankyrin-B is known to target ion channels, transporters, and signaling molecules like Na/K ATPase, Na/Ca exchanger, inositol 1,4,5 trisphosphate (InsP3) receptor. Loss-of-function mutations in the ANK2 gene may affect cardiac conduction, the atrial and ventricular rhythm, and the sinus and atrioventricular nodes.
Cunha et al. [49] investigated 2 previously published large families with severe sinus node dysfunction associated with the ANK2 locus [50], and found that individuals with loss-of-function mutations in ANK2 developed early-onset AF. In additional experiments with atrial myocytes a shortened action potential was found, and loss of L-type Ca2+ current. Furthermore, Cav1.3 was found to function as ankyrin-B–binding target. In ankyrin-B+/− atrial myocytes, Cav1.3 protein expression and membrane function were reduced. Cav1.3 may be a potential molecular mechanism underlying the association between Ankyrin-B mutations and early-onset AF.
AF in the Setting of Other Monogenetic (Cardiac) Conditions
In certain individuals, AF is also related to other monogenic (cardiac) diseases including dilated cardiomyopathy [16, 20, 22, 51, 52], hypertrophic cardiomyopathy [53], peripartum cardiomyopathy [54, 55], arrhythmogenic right ventricular cardiomyopathy [56–58], skeletal myopathies [59–61], long QT syndromes [62–64], short QT syndromes [65–67], Brugada syndrome [68], familial amyloidosis [69], congenital cardiac abnormalities [70, 71], and preexcitation syndromes [72]. In some of these conditions, AF will occur secondary to left ventricular dysfunction rather than to primary atrial disease [73]. These associations are beyond the scope of this chapter and are not further discussed.
Genetic Variants Associated with Common AF
Heritability of AF in the Community
AF predominantly occurs in the setting of concomitant (cardiovascular) conditions, in particular, hypertension, coronary heart disease, valve disease and heart failure. Genetic differences may also contribute to this more common form of AF, explaining why some individuals will suffer repeatedly from AF, while others never will. Data from population-based studies suggest that familial clustering and heritability of AF is widespread and that the common AF phenotype has a significant genetic component [5, 6].
Fox et al. [5] demonstrated that parental AF not only increases the risk for offspring AF in lone AF, but also in the more common forms of AF in the setting of structural diseases. In the Offspring cohort of the Framingham Heart study, they found that 681 out of 2,243 persons (30 %) had at least one parent with documented AF. During follow-up, 70 of these 681 persons (10 %) developed AF. If AF was present in at least one parent, the risk of offspring AF increased by 1.85 (95 % confidence interval 1.12–3.06, p = 0.02) when compared to the absence of parental AF. As expected, the results were stronger if only younger parents and offspring (<75 year) without a previous myocardial infarction, heart failure, or valvular disease were assessed (odds ratio 3.17, 95 % confidence interval 1.71–5.86, p < 0.001). More recently, Lubitz et al. [74] studied the predictive value of familial AF. In 1,185 of the 4,421 individuals (27 %) from the Original and Offspring Cohorts a first-degree relative was documented with AF. Individuals with a first-degree relative with AF more frequently developed AF during follow up than individuals without documented AF in family members. This association (hazard ratio 1.40, 95 % confidence interval 1.13–1.74, p = 0.002) was not altered by adjustment for traditional AF risk factors such as age, sex, body mass index, systolic blood pressure, treatment for hypertension, PR interval, heart murmur, or heart failure. Assessment of AF in a first-degree relative younger than 65 years was associated with a very slight increase in predictive accuracy compared with traditional risk factors. Arnar et al. [6] described similar findings in a cohort of 5,269 inhabitants of Iceland. In this study, 4,195 patients (80 %) with AF were related to others with AF. The risk of AF for first-degree relatives of a family member with AF was 1.77 (95 % confidence interval 1.67–1.88, p < 0.001), when compared with individuals in the general population. In patients younger than 60 years of age the risk for AF increased to 4.67 (95 % confidence interval 3.57–6.08, p < 0.001).
The Danish Twin Registry [75] reported on the risk of AF in monozygotic and dizygotic twins when their co-twin had documented AF. They studied 1,137 same-sex twin pairs in which one or both were diagnosed with AF. The risk of AF was two times higher for a monozygotic twin whose co-twin has AF in comparison to the risk for a dizygotic twin.
Polymorphisms Associated with Common AF
Candidate Gene Association Studies
Numerous candidate gene association studies have been published on common genetic variants that may increase risk of AF (Table 35.2). In general, the candidate genes studied in these reports were previously detected in monogenetic forms of AF. So, some of the underlying mechanisms underlying AF seem similar in both monogenetic AF and more common AF.
Table 35–2.
Single nucleotide polymorphisms associated with common AF
Gene | SNP | Subjects | Type AF | Age | Ethnicity | HR | (A)symp | UHD | Mechanism |
---|---|---|---|---|---|---|---|---|---|
KCNE1 (minK) [76] | S38G | 108 | CAF | 63 | Chin | na | na | Yes | IKs ↓ |
KCNE1 (minK) [78] | S38G | 331 | CAF | 73 | Cauc | na | na | Yes | IKs ↓ |
KCNE5 [79] | C97T | 158 | PAF, CAF | 66 | Cauc | na | na | na | Possible (IKs) |
KCNH2 (HERG) [80] | K897T | 1,207 | PAF, CAF | 69/60a | Cauc | na | symp | Yes | Possible |
KCNH2 (HERG) [81] | rs1805120 | 297 | PAF, CAF | 66 | Chin | na | na | Yes | Possible |
SCN5A [82] | H558R | 157 | PAF | 52 | Cauc | 72 | na | No | Excitability/cond vel ↓ |
SCN5A [83] | M138I | 375 | PAF, CAF | 56 | Multi | na | na | Yes | Excitability/cond vel ↓ |
E428K | |||||||||
H445D | |||||||||
N470K | |||||||||
E655K | |||||||||
T1131I | |||||||||
R1826C | |||||||||
V1951M | |||||||||
eNOS [78] | T-786C | 331 | CAF | 73 | Cauc | na | na | Yes | Stimulate ACE? |
eNOS [88] | G894T | 51 | PAF, CAF | 63 | Cauc | na | na | Heart failure | Stimulate ACE? |
GJA5 [84] | −44A and +71G | 30 | PAF | 33 | Cauc | na | symp | WPW | Dispersed conduction? |
GJA5 [85] | −44A and +71G | 173 | PAF, CAF | 70 | Chin | na | symp | Yes | Dispersed conduction? |
GJA5 [86] | rs10465885 | 1,090 | PAF, CAF | ≤60 | Cauc | na | na | No | Dispersed conduction? |
GJA1[87] | c.932delC | 10 | PAF | <55 | Cauc | na | na | No | Dispersed conduction? |
AGT [90] | M235T | 250 | CAF | 68 | Chin | na | symp | Yes | Atrial remodeling? |
G-6A | |||||||||
G-217A | |||||||||
AGT [91] | A-20C C/C | 968 | PAF, CAF | 68 | Cauc | na | na | 70 % hypertension | Atrial remodeling? |
A-20C C/C and ACE D/D | |||||||||
ACE [88] | D/D | 51 | PAF, CAF | 63 | Cauc | na | na | Heart failure | Atrial remodeling? |
ACE [92] | D/D | 404 | PAF, CAF | 71 | Cauc | na | na | 60 % hypertension | Atrial remodeling? |
ACE [93] | D/D | 97 | PAF, CAF | 73 | Chin | na | na | Hypertension + LVH | Atrial remodeling? |
GNB3 [98] | C825T | 227 | PAF, CAF | 58 | ? | na | na | 59 % hypertension | Possible (IK,Ach) |
IL6 [105] | G-174C | 110 | Post op AF | 61 | ? | na | na | Yes | Inflammation? |
IL10 [106] | C-1306T | 196 | PAF, CAF | 68 | Jap | na | na | 40 % hypertension | Inflammation? |
MMP2 [106] | A-592C | 196 | PAF, CAF | 68 | Jap | na | na | 40 % hypertension | Atrial remodeling? |
MMP9 [108] | C-1562T | 128 | PAF, CAF | 73 | Chin | na | na | Hypertension + LVH | Atrial remodeling? |
TIMP2 [107] | G-418C | 128 | PAF, CAF | 73 | Chin | na | na | Hypertension + LVH | Atrial remodeling? |
NPPA [109] | rs5063 | 384 | PAF, CAF | 59 | Chin | na | na | Yes | Possible |
Potassium Channel Genetic Variants
Alterations in potassium channel function may affect the action potential duration and the effective refractory period in atrial myocytes. The electrophysiological changes may be important for the development of AF.
Lai et al. performed a case control study in 108 consecutive non-familial AF patients from Taiwan with any underlying disease but excluding patients with hyperthyroidism [76]. Mean age was 63 years and a history of hypertension was present in 52 % of the patients. More than 80 % of the patients had permanent AF. Matching was performed regarding sex, age, left ventricular dysfunction and valvular disease. An association between the KCNE1 (encoding the minK β(beta)-subunit of IKs potassium channel) 38G allele and AF was observed. The odds ratios for AF in patients with 1 and 2 KCNE1 38G alleles were 2.16 (95 % confidence interval 0.81–5.74) and 3.58 (95 % confidence interval 1.38–9.27), respectively, compared to patients without the KCNE1 38G allele. Recently, the functional role of this polymorphism has been demonstrated [77].
Fatini et al. [78] likewise found an association between the presence of the 38G minK allele and AF in 331 Caucasian patients. Additionally, they studied several polymorphisms in the endothelial nitric oxide synthase (eNOS) gene including c.894G > T nucleotide exchange in exon 7, a T/C exchange in the promoter region (−T786C), and the eNOS 4a/4b polymorphism. Only eNOS -T786C was significantly more prevalent in patients with AF when compared to healthy volunteers. Surprisingly, the combined presence of minK 38G and eNOS -786C resulted in a stronger predisposition for AF than the minK variant alone.
Ravn et al. [79] investigated the 97T-polymorphism of the KCNE5 gene. This gene is located on the X-chromosome and encodes an inhibitory β(beta)-subunit, MiRP4, of the repolarizing cardiac potassium channel KCNQ1. When compared to 158 patients with AF, a greater proportion of the 96 healthy control subjects was carrier of the 97T-allele (15.2 % vs. 29.2 %). The OR for AF if a patient did not have a copy of the 97T-allele was 2.17 (95 % CI 0.89–5.28) in men and 1.94 (95 % CI 0.80–4.75) in women. This difference may be explained by the localization on the X chromosome. The exact mechanism by which this gene changes atrial electrophysiology has not been investigated yet.
Sinner et al. [80] analyzed 1,207 individuals with AF and 2,475 controls in Germany and genotyped 40 single nucleotide polymorphisms in the KCNH2 (HERG) gene, encoding the α-subunit of the IKr ion channel, important for both ventricular and atrial repolarization. They found 5 polymorphisms associated with AF, of which one (the common K897-allele of the KCNH2-K897T variant) was replicated in an additional cohort of 536 individuals with AF and 1,781 controls. The odds ratio of the association between the K897-allele and AF was 1.25 (95 % CI 1.11–1.41, P = 0.0003). A case–control study performed by Wang et al. [81] included 297 individuals with AF and concomitant cardiovascular diseases, and the same number of controls. They genotyped four single nucleotide polymorphisms in the KCNH2 gene region. Single nucleotide polymorphism rs1805120, located in exon 6 of the KCNH2 gene, was associated with an increased risk of AF (odds ratio 1.45, 95 % confidence interval 1.09–1.93, P = 0.012). The underlying causal mechanism linking these common polymorphisms in KCNH2 to AF needs to be elucidated.
Sodium Channel Genetic Variants
In contrast to potassium channel alterations it is less clear which effects loss-of-function sodium channels exert. Reduced sodium channel functionality may lead to decreased excitability of atrial myocardium.
Chen et al. [82] performed targeted genotyping of a common loss-of-function p.H558R polymorphism of the SCN5A gene in 157 patients with early-onset lone AF, and 314 matched controls. In comparison to the controls, the R558 allele was more common in AF patients (30 versus 21 %, P = 0.002), resulting in an odds ratio for AF of 1.6 (95 % confidence interval 1.2–2.2). Darbar et al. [83] genotyped the SCN5A gene for the presence of mutations or rare variants in cohort of 375 AF patients, 118 with lone AF and 257 with AF in the setting of heart diseases. In ten probands (2.7 %), eight novel variants not found in the control population (0 %, P = 0.001) were discovered, that were predicted to disturb cardiac sodium channel function. In 6 families with more than one affected family member, the variant segregated with AF. In addition, 11 rare missense variants in 12 probands (3.2 %) that have previously been described in patients with inherited arrhythmia syndromes (congenital long QT syndrome, Brugada syndrome) were found in this cohort.
Gap Junction Protein Genetic Variants
Loss of gap junction protein function may lead to dispersed conduction and increase the vulnerability for AF.
Firouzi et al. [84] reported that a GJA5 gene, encoding connexin 40, promoter polymorphism (−44AA genotype) is linked to an increased coefficient of spatial dispersion of refractoriness and an increased risk of AF (Table 35.2). They investigated the coefficient of dispersion, defined as the standard deviation of all local mean fibrillatory intervals expressed as a percentage of the overall mean fibrillatory interval, in 30 unrelated adults. These patients had no structural heart disease and underwent an electrophysiological study because of the presence of an accessory pathway (n = 27) or atrioventricular nodal reentrant tachycardias (n = 3). Of these subjects, 14 had prior documented sporadic episodes of AF whereas 16 had no history of AF. The AF patients suffered a mean of 1 (range 1–5) previous episode of AF with a median duration of 1 h (range 15 min to 3 h). The AF free interval before the study was 148 days (range 9–365 days). The prevalence of the minor connexin 40 allele (−44A) and –44AA genotype was significantly higher in subjects with increased dispersion (n = 13) compared to patients with a normal coefficient of dispersion (n = 17, p = 0.00046 and p = 0.025, odds ratio 6.7 and 7.4, respectively). Subjects with the –44AA genotype had a significantly higher coefficient of dispersion as compared to those with the –44GG genotype. Finally, all subjects with an increased dispersion had a history of AF compared with only 1 subject with a normal coefficient of dispersion. The mechanism is at present unknown. It may be surmised that an abnormal gap junction distribution may cause abnormal conduction with increased anisotropy. By creating a substrate, this may result in an increased propensity for AF.
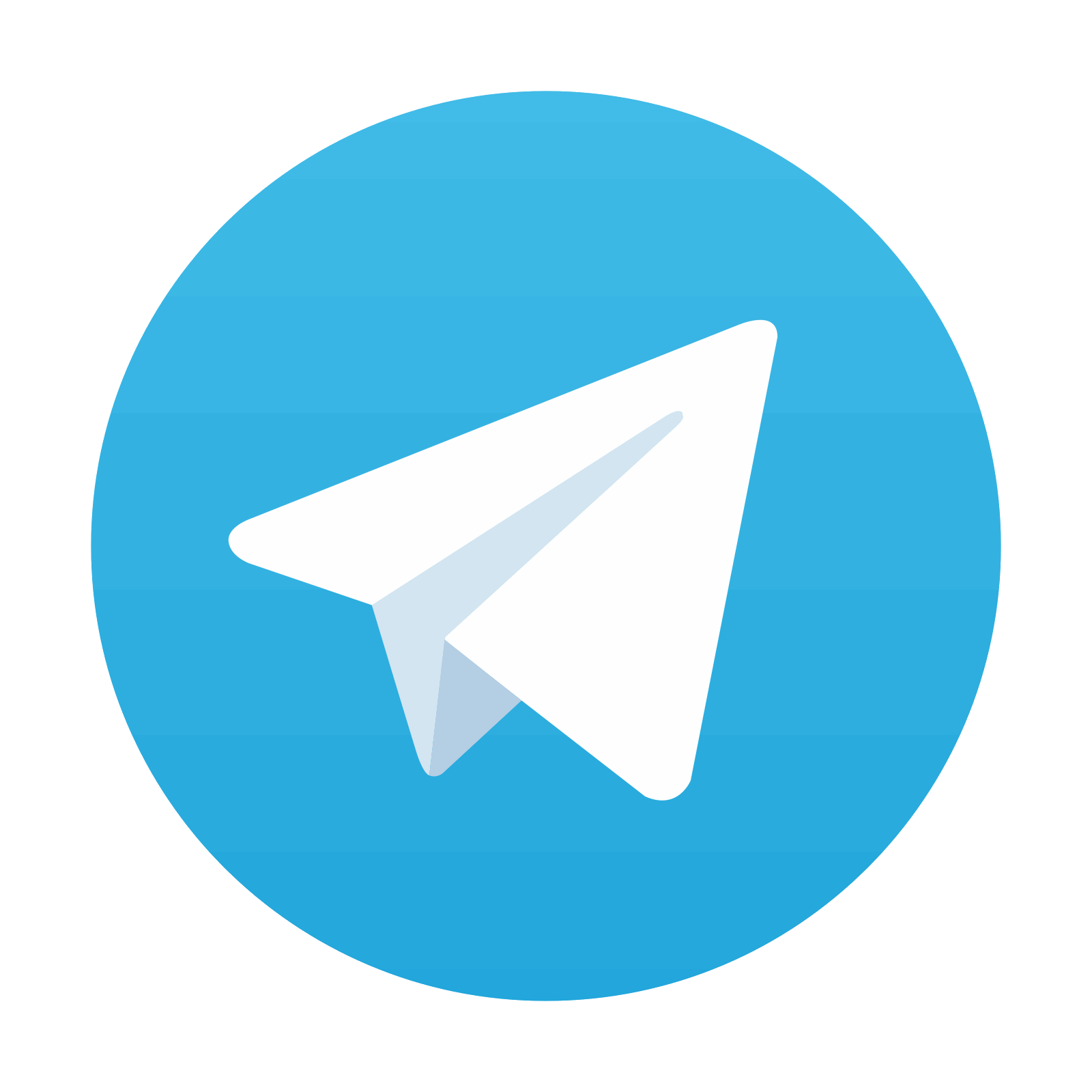
Stay updated, free articles. Join our Telegram channel

Full access? Get Clinical Tree
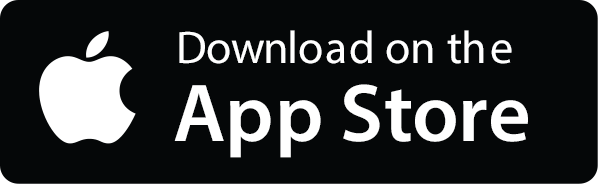
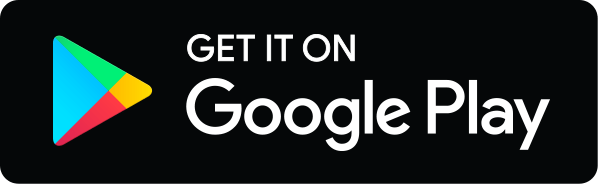