Fig. 8.1
Pathophysiological mechanisms contributing to vascular disease
The withdrawal of NO-mediated beneficial effects on vascular function and to the formation of free radicals with proatherogenic properties. In particular, the term “oxidative stress” describes conditions involving increased ROS levels, produced by enzymes within vascular tissue, such as nicotinamide adenine dinucleotide phosphate oxidase (NADPH), and xanthine oxidase [22]. Also, tetrahydrobiopterin, an essential cofactor for NO synthases, has been recently suggested as a critical determinant for eNOS activity. Moreover, significant data have demonstrated that vascular but not plasma tetrahydrobiopterin (BH4) is a central factor of eNOS coupling, endothelium-dependent vasodilation, and superoxide production in human vessels, whereas plasma biopterins are associated with systemic inflammation [23, 24].
NO is essential to vascular homeostasis, while disturbance of the eNOS ability to produce NO is a major contributor to the pathogenesis of vascular disease. It has been shown by in vivo studies that expression of eNOS is vital for endothelial function and that this enzyme is subjected to significant degrees of regulation by numerous physiological and pathophysiological stimuli via mechanisms that alter steady-state eNOS mRNA levels. Such stimuli include shear stress, transforming growth factors, cell growth, hydrogen peroxide (H2O2), hypoxia and oxLDL [25–27]. Moreover, it is well-known that oxidative stress is implicated in all stages of inflammation, including hypertrophy, apoptosis, migration, fibrosis, angiogenesis and ED participating in complex processes leading in vascular remodeling. It stimulates inflammatory processes, via multiple intracellular proteins, enzymes and transcription factors activated by ROS [28, 29]. Importantly, angiotensin-II (Ang II) plays a pivotal role in cascades leading in expression of proinflammatory mediators and extracellular matrix modification. Accordingly, vascular NADPH oxidase is activated, and the produced ROS stimulate redox-sensitive inflammatory genes, such as those encoding monocyte chemotactic protein-1 (MCP-1) and IL-6 [29, 30].
8.2.2 Biomarkers in Vascular Disease
Comprehension of mechanisms which are implicated in the development of ED is vital for establishing biomarkers as potential diagnostic tools and designing prevention or treatment strategies. This approach could be equally attractive in both preclinical and clinical ATH and accordingly, in CVDs [31]. According to recent studies, selected biomarkers may be associated with ED and scientific interest has focused on detection and monitoring of them (Table 8.1).
Table 8.1
Biomarkers associated with endothelial dysfunction
Category | Biomarker (plasma) |
---|---|
Acute phase protein | CRP |
Adhesion molecules | ICAM-1 |
VCAM-1 | |
Cytokines/chemokines | IL-1, IL-6 |
TNF-a | |
MCP-1 | |
Pro-coagulant and thrombotic markers | PAI-1 |
Fibrinogen | |
vWF | |
Other biomarkers | EPCs |
Soluble CD40 ligand | |
Selectins (E-Selectin) | |
Tetrahydrobiopterin |
8.2.2.1 C-Reactive Protein (CRP)
The most exploited circulating inflammatory marker in plasma is CRP. In addition to its role as a marker, it can exert modulatory effects through its well-demonstrated presence in atherosclerotic plaques [32]. CRP is an acute-phase protein produced in the liver and has a homopentameric structure, each with 206 amino acids that are combined in a three-dimensional structure, and Ca2+-binding specificity for phosphocholine [33]. Besides being a sensitive marker for the detection of subclinical inflammation, CRP has been suggested to participate in the pathophysiology of ATH. Moreover, it stimulates the secretion of proinflammatory cytokines and tissue factor and may contribute to vascular remodeling via its effects on eNOS [34].
A growing body of literature has demonstrated that CRP levels may be correlated with ED. Moreover, it may stimulate expression of various biomarkers, such as ICAM, vascular cell adhesion molecule 1 (VCAM-1) and E-selectin [35]. Moreover, according to experimental data CRP may also induce a significant impairment of endothelial-dependent vasorelaxation in various conditions which could lead to an increased cardiovascular risk profile [36]. In line with this evidence, Venugopal et al. [37] have demonstrated that CRP decreased eNOS mRNA, protein abundance, and enzyme activity in cultured human aortic ECs. Furthermore, increased CRP plasma levels across the coronary circulation have been associated with impairment in coronary endothelial-dependent function, even though its relationship with the severity and extent of coronary atherosclerosis is not clear [38].
8.2.2.2 Other Biomarkers in ED
Impaired endothelium dependent vasodilation along with alterations in the expression of adhesion molecules and proinflammatory molecules are present in state of ED. More specifically, increased IL-6, TNF-a, and especially ICAM-1, VCAM-1, and E-selectin are associated with ED. These molecules may also participate in ECs stimulation to promote an atherogenic phenotype [39]. In addition, other chemotactic factors could be expressed such as MCP-1, macrophage colony-stimulating factor, and TNF-β which contribute to the development of inflammation within the arterial wall related with the pathogenesis of atherosclerosis [40]. As aforementioned, vascular endothelium produces various other molecules that affect blood fluidity and thrombosis, such as plasminogen activator inhibitor-1 (PAI-1), tissue factor and vWF. These in turn, are regulated by the production of NO, prostacyclin, tissue plasminogen activator and thrombomodulin [41]. Moreover, CD40 ligand (CD40L) is expressed on monocyte/macrophages, ECs, smooth muscle cells and platelets, and as a consequence of CD40L binding to CD40, several inflammatory processes are initiated by the release of cytokines and the expression of adhesion molecules. In particular, the link between CD40/CD40L and CVDs has been established in numerous studies [41]. The pathophysiological substrate is still under investigation however, evidence suggests that initially, CD40L has an unfavorable effect on the vascular redox state [41].
Accordingly, it has been suggested that circulating markers of oxidative stress, including F2 isoprostanes and antibodies against oxLDL, are increased in humans with diabetes [42]. Notably, given that there is oxidative degradation of BH4 by ROS in ED, BH4 plasma levels along with levels in the vascular wall could reflect vascular endothelial health [43]. Moreover, clinical data have suggested that hyperhomocysteinemia may be associated with impaired renal function, potentially through affecting endothelial function. Thus, plasma homocysteine levels may be considered as an intermediate factor between ED and renal function [44]. Also, asymmetrical dimethylarginine (ADMA) as an endogenous eNOS inhibitor has been associated with ED in several CVDs, such as hypercholesterolemia and coronary artery disease [45]. It is worth mentioning, endothelial progenitor cells (EPCs) are currently considered to participate in vascular repair and maintain endothelial integrity thus, they may exhibit a role as prognostic biomarker in CVD. EPC number and function may be used as biomarkers and more specifically, reduced circulating numbers and functional capacity may predict future cardiovascular events, independently of other cardiovascular risk factors [46].
8.3 Genetic Contribution in Vascular Endothelium: The Role of Polymorphisms
Currently, a growing body of evidence has emerged regarding genetic component and its role in the aim of assessing vascular endothelium. Of note, genetic variation within the population seems to determine endothelial responses and potential modify both atherogenesis and an individual’s responses to risk factors. It has been estimated that only 10–20 % of the variation in endothelial function may be accounted for by genes [47].
8.3.1 eNOS
The eNOS gene includes 26 exons, 25 introns and it is located at 7q35–7q36 of chromosome 7, while the protein consists of 1,203 amino acids and has a molecular weight of 133 kDA [48]. It has been the focus of intensive research to identify potentially functional polymorphisms influencing ED, as more than 100 polymorphisms have been identified in the eNOS gene (Table 8.2). Some of them (e.g. T–786C promoter polymorphism) are situated in the eNOS promoter and might influence mRNA transcription reducing gene expression [53]. More specifically, Nakayama et al. [53] have demonstrated the existence of three linked mutations in the 5′-flanking region of the eNOS gene (T-786C, A-922G, and T-1468A) which are more prevalent in patients with coronary spasm than in the control group. Also, the T-786C mutation resulted in a significant reduction in eNOS gene promoter activity, whereas neither the A-922G nor the T-1468A mutation had any affect, suggesting that this single nucleotide polymorphism (SNP) may reduce endothelial NO synthesis and predispose to coronary spasm.
Table 8.2
Most important single nucleotide polymorphisms of eNOS gene and their relationship with endothelial dysfunction
SNPs | Participants | Examined parameters | Comments |
---|---|---|---|
G894T [49] | 104 patients with CAD | Endothelium-dependentvasorelaxations | It is associated with impairedNO-mediated endothelialvasomotor function |
G894T [50] | 248 healthy subjects | FMD/GTN-induced dilation | It is associated with differences in endothelial responses to bothsmoking and n-3 FA in healthyyoung subjects |
G894T [51] | Human endothelial cells | Subcellular localization andinteraction of eNOS withmodulatory proteins | It does not have a major effect inmodulating eNOS activity in vivo |
G894T [52] | 56 patients with ahistory of premature MI | Forearm blood flow | It is associated with impairedendothelial function and higherlevels of von Willebrand factor |
T786C [53] | 11 patients with CAD and 9 controls | Endothelial NO synthesis and coronary spasm | It is associated with reducedendothelial NO synthesis andpredisposes to coronary spasm |
T786C [54] | Endothelial cellscultures (154 patientswith CAD and 174non-CAD subjects) | NO-dependent relaxation was examined in segments of saphenous vein | It is associated with blockingshear stress-dependentmaintenance of NOS-3 expression |
Shear stress-induced NOS-3mRNA and protein expression | |||
ecNOS4a/b [55] | 549 subjects with, and 153 without CAD | Distribution of polymorphismin CAD in relation to smoking | It is associated with predispositionto endothelial dysfunction |
A922G [53] | 11 patients with CADand 9 controls | Endothelial NO synthesis and coronary spasm | It is associated with coronary spasm |
T1468A [53] | 11 patients with CADand 9 controls | Endothelial NO synthesis and coronary spasm | It is associated with coronary spasm |
One of the most studied NOS polymorphisms is the polymorphism in exon 7 of NOS3, a G-T transition at position 894 that results in a Glu to Asp amino acid substitution for codon 298 (894GT) within exon 7. It is the only common polymorphism identified thus far that encodes an amino acid substitution. Significant data have shown that eNOS Asp298 is subjected to selective proteolytic cleavage in ECs and vascular tissues that might account for reduced vascular NO generation, even though it has been elsewhere suggested that eNOS Asp298 could be a marker for another possibly functional variant [51, 56, 57]. Moreover, given a novel method to accurately determine molar quantities of each variant of G894T polymorphism (expressing them as green fluorescent protein fusion proteins and using recombinant adenoviruses to facilitate transient infection of human microvascular ECs), the Asp substitution at 298 does not seem to have a major effect in modulating eNOS activity in vivo [51]. In turn, Guzik et al. [49] have investigated the relationships between NO-mediated endothelial function with the presence of the eNOS Glu298Asp variant via endothelium-dependent vasorelaxation to different agonists which were determined in human saphenous veins of coronary artery disease (CAD) patients. NO-mediated endothelial vasorelaxation was highly variable between patients, and reduced values were associated with increased number of clinical risk factors for ATH. This variant was not associated with any differences in contractions to phenylephrine, NO-mediated vasorelaxations to acetylcholine, bradykinin or calcium ionophore, nor relaxations to the NO donor sodium nitroprusside, suggesting that this polymorphism may not have a major direct functional effect on vascular eNOS activity in human ATH [49].
More recently however, a study by Antoniades et al. [52] has provided new evidence about its potential association with endothelial function and with markers of ECs injury and activation. The study population consisted of 56 patients with a history of premature myocardial infarction, while the forearm blood flow was measured using strain-gauge plethysmography. Of note, the presence of 894 T allele on eNOS gene is associated with impaired endothelial function and higher levels of von Willebrand factor in relatively young patients with myocardial infarction. Subsequently, the same investigators have attempted to explore the determinants of GCH1 gene expression, which codes for a rate-limiting enzyme in the biosynthesis of BH4, an eNOS cofactor important for maintaining enzymatic coupling. They have demonstrated that GCH1 gene expression modulated by a particular GCH1 haplotype, is a major determinant of BH4 bioavailability both in plasma and in the vascular wall thus, this genetic variation may be a determinant of eNOS coupling, vascular redox state, and endothelial function in human vascular disease [58].
8.3.1.1 CRP Gene Variation
Its role has already been discussed in Sect. 8.2.2. It has been suggested to participate in several related processes, such as the binding of LDL-cholesterol from macrophages to form foam cells and the development of vulnerable plaques, while it stimulates the secretion of pro-inflammatory cytokines and tissue factor, thus contributing to vascular remodeling [59, 60]. Moreover, CRP levels have been inversely correlated with ED and with the prediction of future or recurrent cardiovascular events [61]. The CRP gene is located on chromosome 1 in the region 1q21–q23. It consists of two exons encoding the CRP monomer of 206 amino acids, with an intervening intron, while the circulating protein is a symmetric, noncovalently associated pentamer with a central pore [62]. Due to the fact that circulating levels of CRP are widely used to determine inflammatory response, it is not surprising that CRP gene variation has been studied in relation to inflammatory disorders. More specifically, it seems that CRP SNPs may be associated with the risk of developing inflammatory disease and also with its severity [63]. Furthermore, multiple CRP gene polymorphisms have been shown to associate with circulating CRP, and in turn with CVD. According to Lawlor et al. [64], however, according to data derived from five large trials (a total of 18,637 individuals) rs1130864 (on the 3′ untranslated region of CRP) was associated with serum CRP, and serum CRP was associated with coronary heart disease, though there was no a direct association between the SNP and disease.
8.3.1.2 Cytokines
Most of the cytokines are glycoproteins with a monomeric molecular mass of 15–25 kDa. IL-6 and TNF-a are some of the main cytokines participating in atherogenesis and as regards their structure, IL-6 is a four helix bundle, while TNF-a is a trimeric protein [1]. Notably, genetic variants of interleukin genes have been associated with their plasma concentrations and with the risk of CVDs [65, 66]. Moreover, according to experimental studies which examined the functional effect of four polymorphisms in the IL-6 promoter (G-597A, G-572GC, −373A(n)T(n), G-174GC), more than one of the polymorphic sites is functional and they may influence IL-6 transcription not by a simple additive mechanism but rather through complex interactions determined by the haplotype [67]. Also, Stoica et al. [68] have recently evaluated the association between IL-6 (G-174 T, nt565 G/A) and IL-10 (G-1082A, C819T, C-592A) gene polymorphisms with the short-term risk of postoperative cardiovascular events and with endothelial function in patients with peripheral artery disease (Table 8.3). They have shown that IL-6 -174CC and nt565AA genotypes, as well as IL-10 ATA haplotypes are correlated with ED in these patients, as indicated by decreased values of flow mediated dilation (FMD), and with a high short-term risk of acute postoperative cardiovascular events. Moreover, in the setting of non-ST-elevation acute coronary syndromes (ACS), genetic variation at the IL-1 gene locus contributes to the changes in soluble endothelial activation markers (vWF and E-selectin) [75]. It is also worth-noting that according to preliminary data, there is a significant and independent association between the IL-6 G-572C gene polymorphism (presence of C allele) and adolescents with a family history of premature ATH [76].
Table 8.3
Most important single nucleotide polymorphisms associated with endothelial dysfunction
Study | SNPs | Genes | Comments |
---|---|---|---|
Antoniades et al. [52] | G894T | eNOS | Association with impaired endothelial functionand higher levels of von Willebrand factor |
Nakayama et al. [53] | T786C | eNOS | Association with reduced endothelial NOsynthesis and predisposition to coronary spasm |
Wang et al. [55] | ecNOS4a/b | eNOS | Association with predisposition to endothelial dysfunction |
Nakayama et al. [53] | A922G | eNOS | Association with coronary spasm |
Nakayama et al. [53] | T1468A | eNOS | Association with coronary spasm |
Stoica et al. [68] | G-174 T | IL-6 | Association with ED |
Stoica et al. [68] | nt565 G/A | IL-6 | Association with ED |
Stoica et al. [68] | G-1082A | IL-10 | Association with ED (ATA haplotypes) |
C819T | |||
C-592A | |||
Ponthieux et al. [69] | G/R241 | ICAM-1 | Association with serum ICAM-1 levels |
Motawi et al. [70] | K469E | ICAM-1 | Association with vascular disease |
Motawi et al. [70] | Ser128Arg | E-selectin | Association with vascular disease |
Yoshida et al. [71] | Thr715Pro | E-selectin | Association with predisposition to atherosclerosis |
Vadapalli et al. [72] | 3A/4A | ET-1 | Association with more pronounced ED |
Lys198Asn | |||
Ezzidi et al. [73] | 4G/5G | PAI-1 | Association with PAI-1 levels and microvascular disease |
G-844A | |||
Robinson et al. [74] | C-7351T | tPA | No association with endothelial function and microvascular disease |
T20 099C | |||
T27445A |
8.3.1.3 Adhesion Molecules-Chemokines
Cell adhesion molecules (CAMs) are transmembrane glycoproteins consisting of an extracellular component with a hydrophobic transmembrane component and an intracytoplasmic component. Integrins, the immunoglobulin superfamily and the selectins are the three families of CAMs. Also, chemokines are members of a group of structurally related and secretable, chemotactic cytokines divided into four families (CC, CXC, CX3C, XC) and can be located in different vascular cell types, such as ECs but also inflammatory cells [77, 78]. ICAM-1 is thought to be associated with non-ECs inflammation, while VCAM-1 is expressed more locally within the vascular system. It has been assessed the effect of a single-base polymorphism at codon 241 in exon 4 of ICAM-1 gene on serum ICAM-1 (sICAM-1) concentration in a large healthy population, taking into account other biological determinants of sICAM-1 level. The R241 allele was significantly associated with lower sICAM-1 levels and explained 3.4 and 1.9 % of the sICAM-1 variability in children and adults, respectively, probably due to the impairment in binding of ICAM-1 to leukocyte integrin Mac-1 protein [69].
< div class='tao-gold-member'>
Only gold members can continue reading. Log In or Register a > to continue
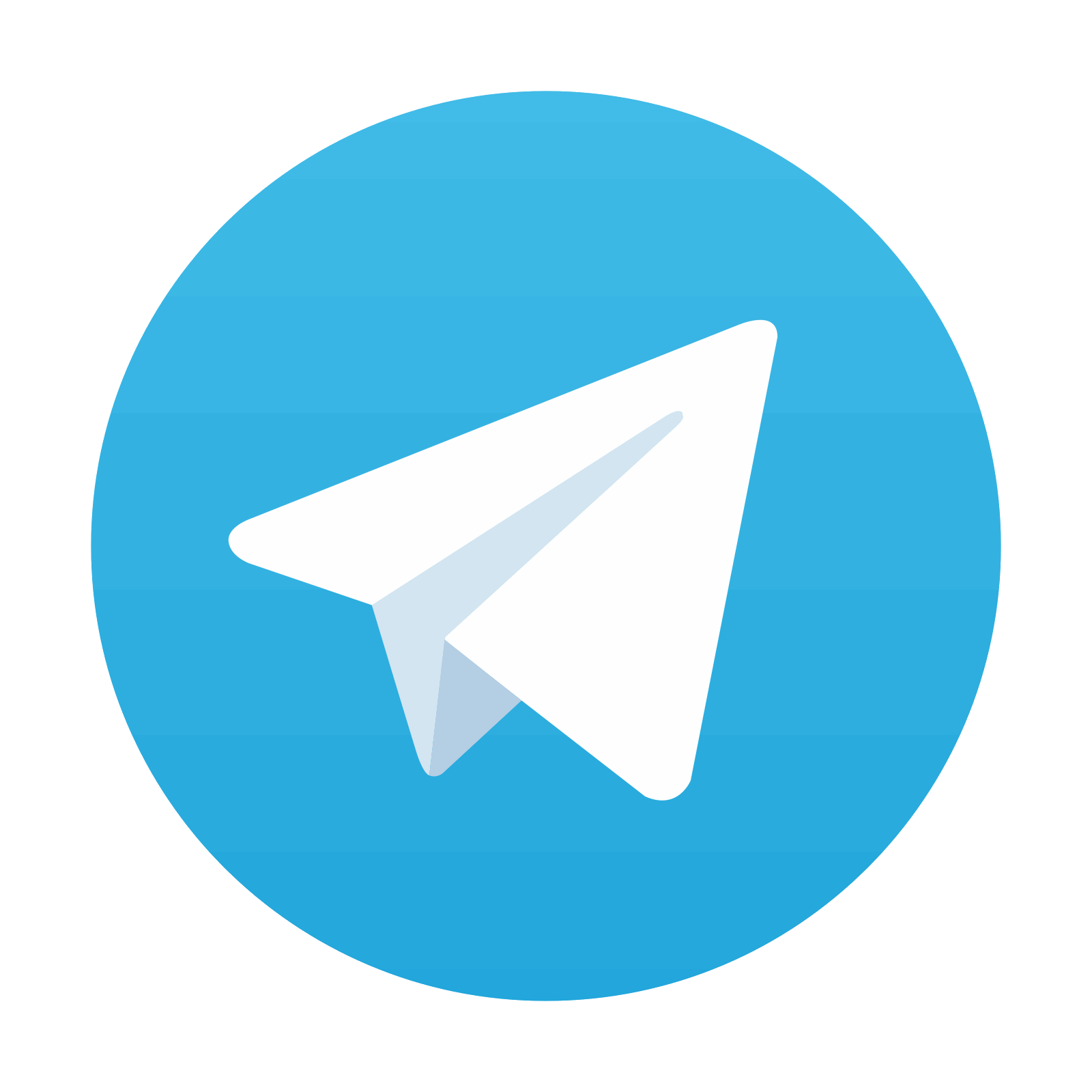
Stay updated, free articles. Join our Telegram channel

Full access? Get Clinical Tree
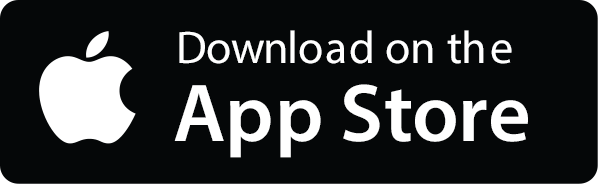
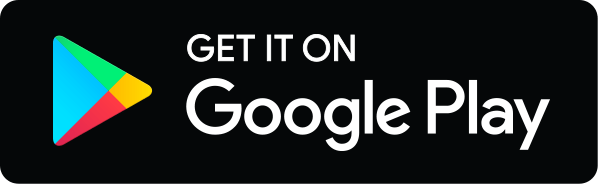