, Hui-Ping Liu1 , Sean C. Wightman2, Mark K. Ferguson3 , Christopher W. Seder4 , Michael J. Liptay4, Yi Feng5 and Juan Zhu5
(1)
Department of Thoracic Surgery, Chang Gung Memorial Hospital, Taoyuan, Taiwan
(2)
Department of Surgery, The University of Chicago, 5841 S. Maryland Avenue, MC 5040, Chicago, IL 60637, USA
(3)
Thoracic Surgery Service, University of Chicago Medicine, 5841 S. Maryland Avenue, MC 5040, Chicago, IL 60637, USA
(4)
Department of Cardiovascular and Thoracic Surgery, Rush University Medical Center, 1725 W. Harrison St, Suite 774, Chicago, IL 60612, USA
(5)
Department of Anesthesiology, Peking University People’s Hospital, No.11 Xizhimen South St, Beijing, 100044, China
The corresponding author of section 2.1 is H.-P. Liu, Email: hpliu125@ms21.hinet.net (✉);
The corresponding author of section 2.2 is Mark K. Ferguson, Email: mferguso@surgery.bsd.uchicago.edu (✉);
The corresponding author of section 2.3 is C.W. Seder, Email: Christopher_w_seder@rush.edu (✉);
The corresponding author of section 2.4 is Yi Feng, Email: yifeng65@sina.com (✉)
2.1 Overview of Minimally Invasive Surgery in Lung Cancer
(6)
Department of Thoracic Surgery, Chang Gung Memorial Hospital, Taoyuan, Taiwan
2.1.1 History of Development of Minimal Invasive Thoracic Surgery
The application of thoracoscopy can be traced back to 100 years ago, when Dr. Jacobaeus first reported his experiences in the diagnosis and treatment of pleural effusions by thoracoscope in 1909 [1]. Most patients who needed to undergo thoracoscopy at that time suffered from pulmonary tuberculosis [2]. The development of fibro-optic light transmission, the illumination and the image processing techniques, as well as the refinement of related instruments made video-assisted thoracoscopy more easily and broadly applied after the 1990s [3, 4]. And now video-assisted thoracic surgery (VATS) has become a basic and important technique for a thoracic surgeon.
2.1.2 Definition of VATS Lobectomy
Frequently, minimal invasive thoracic surgery for lung cancer includes three approaches: (1) VATS, (2) Hybrid VATS and (3) Hand-assisted VATS. VATS usually refers to thoracic surgery that involves insertion of instruments through one (Uniport) or two to four small chest incisions under two-dimensional video images, and hand-assisted VATS mainly refers to thoracic surgery performed by inserting the surgeon’s hand into the chest cavity through one of the chest incisions to control the target organs under a two-dimensional video images. A “Hybrid VATS” was defined as using a rib spreading retractor and operates directly through the thoracotomy (usually 8–10 cm in length). In these cases the camera is only used for illumination. Recently VATS can be performed through uniportal or subxiphoid approach for lung lesion.
In 2012, the VATS Lobectomy Consensus Meeting was held in Edinburgh, UK, which marked the 20th anniversary of this procedure. For the first time in history, consensus agreements on several important issues on VATS lobectomy, including its definition, patient eligibility, surgical standard of care were reached [5]. Since then, the Cancer and Leukemia Group B (CALGB) definition has become the globally accepted state-of-the-art VATS lobectomy technique [6], which comprised (1) no use of rib-spreading, (2) a maximum length of 8 cm for the utility incision (3) individual dissection of pulmonary vessels and bronchus. While a small retractor isonly acceptable in selected circumstances, such as conducting complex procedures (e.g. sleeve resection) or delivery of a large specimen.
2.1.3 Minimally Invasive Surgery in Lung Cancer: Current Evidence
In the current era, a prospective, randomized comparison of open versus VATS lobectomy for lung cancer will likely never occur, leaving us to rely on the best available current evidence to draw meaningful clinical conclusions. In the following sections, several important studies to address this issue.
2.1.3.1 Impact on Perioperative Outcome
Perioperative outcomes of VATS versus open lobectomy have been compared in one small prospective trial and five retrospective case control series, and one large systematic review including more than 6,000 patients [7–12]. All of these studies indicate less perioperative morbidity for VATS lobectomy (10–30 %) than for open lobectomy (20–50 %). The mortality rates are similar between the two procedures. Table 2.1 summarizes the published studies that directly compare VATS versus open lobectomy.
Table 2.1
Studies comparing perioperative outcome between VATS and open procedure
Author/year | Study design | Procedure | N | Morbidity rate (%) | Mortality rate | LOS (days) |
---|---|---|---|---|---|---|
Kirby, 1995 | Prospective | VATS | 25 | 24 | 0 % | 7.1 |
Open | 30 | 53 | 0 % | 8.3 | ||
Whitson, 2008 | Systematic review | VATS | 3114 | 16.4 | NA | 8.3 |
Open | 3256 | 31.2 | NA | 13.3 | ||
Handy, 2009 | Retrospective | VATS | 49 | 10 | 4.1 % | 5.2 |
Open | 192 | 22.5 | 2.6 % | 6.6 | ||
Villamizar, 2009 | Retrospective | VATS | 284 | 31 | 3 % | 4 |
Open | 284 | 51 | 5 % | 5 | ||
Flores, 2009 | Retrospective | VATS | 398 | 23 | 0.3 % | 5 |
Open | 343 | 33 | 0.3 % | 7 | ||
Stephens, 2014 | Retrospectivea | VATS | 307 | 37 | <1 % | 4 |
Open | 307 | 19 | 2 % | 6 | ||
Nwogu, 2015 | Retrospectivea | VATS | 175 | 14.9 | 1.7 % | 5.4 |
Open | 175 | 25.1 | 1.7 % | 8 |
2.1.3.2 Oncological Perspective
Critics of VATS lobectomy suggest that inadequate nodal sampling and the potential for port site contamination by tumor will lead to inferior survival compared to open lobectomy. Important studies comparing nodal clearance and survival between VATS and open lobectomy were discussed in the following sections.
Nodal Clearance: VATS Versus Open
Three prospective trials have investigated the adequacy of nodal sampling during VATS lobectomy. The first is a small trial of 29 patients [13]. What is unique about this study is that following VATS dissection, a thoracotomy was carried out by another surgeon and any remaining mediastinal lymph nodes were removed. Based on weight and number of nodes, the authors concluded that only 2–3 % of nodal tissue was “missed” with thoracoscopic techniques. Two other randomized studies have also documented a equal degree of lymph node clearance between VATS and open procedure. In the second trial, the mean number of hilar and mediastinal nodes removed during open lobectomy were 8 and 13 respectively, exactly the same as in the VATS group. Furthermore, an equal number of patients were upstaged to N1 or N2 disease in each group [14]. In the third trial, 39 patients were randomized to undergo either a complete VATS or an “Hybrid-VATS” (in which the thoracotomy was 10 cm in length and rib-spreading was used) [15]. In the complete VATS group 32 nodes were submitted for pathologic review, compared to 29 in the control group (p = 0.12).
Long Term Survival
The first and only randomized trial to report survival data was published in 2000 from Japan [14]. In this trial 100 patients with clinical stage IA lung cancer were randomized to either a VATS or open lobectomy. With the median follow-up of 4.9 years, 6 % of patients in both group developed a local recurrence. The 5-year survival was 85 % in the open group and 90 % in the VATS group (p = 0.91).
Several retrospective reports support the aforementioned findings. In these separate reports, the 5-year survival for VATS lobectomy is near 80 %, similar to that for open lobectomy (75–82 %). In a 2008 systematic review, Whitson and colleagues provided an analysis of 39 studies comparing VATS with open lobectomy [16]. In this study, patients with VATS lobectomy had similar survival when compared with those who underwent open resection. At 4 years, patients who underwent VATS lobectomy even had improved survival versus patients with open lobectomy (88.4 % vs 71 %; p = 0.003), suggesting that VATS lobectomy is at least oncologically equivalent to open lobectomy. Table 2.2 summarized the major findings from the aforementioned studies.
Table 2.2
Studies comparing survival of VATS versus open lobectomy for early-stage non-small cell lung cancer
Author/year | Study design | Procedure | N | 5 years-survival (%) | P value |
---|---|---|---|---|---|
Sugi, 2000 | Prospective randomized | VATS | 48 | 90 | N.S |
Open | 52 | 85 | |||
Yang, 2009 | Retrospective | VATS | 43 | 79 | N.S |
Open | 98 | 82 | |||
Flores, 2009 | Retrospective | VATS | 398 | 79 | N.S |
Open | 343 | 75 | |||
Whitson, 2008 | Systematic review | VATS | 3114 | 80 | N.S |
Open | 3256 | 65.6 | |||
Stephens, 2014 | Retrospective | VATS | 307 | 78 | N.S |
Open | 307 | 73 |
2.1.4 Summary
To the best of my knowledge, there has been no publication thus far demonstrating inferior outcomes of VATS lobectomy compared to open thoracotomy. Without a doubt, VATS has completely revolutionized modern thoracic surgery and significantly improved patient outcomes over the last two decades.
2.2 Physiologic Evaluation of Candidates for Lung Cancer Resection
(7)
Department of Surgery, The University of Chicago, 5841 S. Maryland Avenue, MC 5040, Chicago, IL 60637, USA
(8)
Thoracic Surgery Service, University of Chicago Medicine, 5841 S. Maryland Avenue, MC 5040, Chicago, IL 60637, USA
2.2.1 Introduction
One goal of perioperative surgical care is to minimize postoperative complications. This process includes preoperative decision making, intraoperative judgment and technical considerations, and postoperative care. Knowing which clinical factors are associated with complications permits physicians to assess which candidates are appropriate for major surgery. Furthermore, it allows patients to both understand the risk of procedures and make an informed decision on agreeing to a planned operation versus pursuing an alternative treatment modality. The development and standardization of algorithms for evaluation of risk has made risk estimation more uniform. There is increasing understanding of how to mitigate risk through appropriate preoperative interventions or through altering standard surgical approaches to minimize risk while maintaining the original treatment objectives. Knowing potential risks for a given patient allows the treatment teams to allocate intraoperative and postoperative resources to identify and treat complications more efficiently, thus increasing resiliency.
2.2.2 Background
After pulmonary resection, there are substantial changes in the physiology of the cardiopulmonary system. Lung resection decreases postoperative pulmonary function as measured by forced expiratory volume in the first second (FEV1) and the shuttle walk test [17]. While FEV1 decreases maximally the first few days postoperatively, it improves to only 75 % of predicted values at 1 month and 85 % at 3 and 6 months [17]. Similarly, the shuttle walk test is 70 % of its preoperative value when assessed at 1 month and improves to 84 % of preoperative values when reassessed at 6 months [17]. The immediate postoperative decrease is related to reduced respiratory mobility of the ribs and diaphragm after a thoracic operation, predominantly due to pain. This subsequently leads to decreased pulmonary expansion, alveolar collapse, and subsequent atelectasis [18].
The changes in pulmonary spirometry are associated with the degree of pulmonary resection performed. For patients undergoing a segmentectomy, FEV1 and forced vital capacity (FVC) are relatively preserved [19]. After lobectomy, and once recovered from the initial postoperative period, spirometry values for FVC, FEV1 and total lung capacity (TLC) are reduced by 7–10 % [20]. For those patients undergoing pneumonectomy, FVC, FEV1, and TLC are typically decreased by 30–35 % [20]. In patients undergoing wedge or lobectomy, no postoperative changes in cardiac hemodynamics are observed [21]. Pneumonectomy is associated with elevated right ventricular volumes at end-systole and end-diastole, while right ventricular ejection fraction is decreased by 10 % [21]. The decreased ejection fraction after pneumonectomy is likely due to the increased afterload, while the increased right heart volume compensates to improve ejection based on the Frank-Starling Curve. These changes underline the importance of preoperative evaluation to determine which patients are likely to tolerate surgery without increased cardiopulmonary risk.
General anesthesia adversely affects pulmonary function by relaxing respiratory muscles leading to a reduction in functional residual lung volume. This in turn collapses bronchioles leading to atelectasis [22]. These changes contribute to postoperative hypoxemia and can last many days after surgery. A thoracotomy incision reduces chest wall compliance leading to decreased total lung volume due to limited expansion. Appropriate pain control and minimally invasive procedures are thought to limit this physiologic restriction.
Induction chemotherapy reduces diffusion capacity of the lung for carbon monoxide (DLCO) in 15 % of patients [23]. This reduction does not typically affect clinical symptoms and only 2 % of patients are ineligible to proceed with surgery based on the change in DLCO. For heavy smokers, the reduction after induction chemotherapy is greater. The relation of induction chemotherapy to increased post-operative complications is controversial [23, 24]. Due to pulmonary changes after induction chemotherapy, an updated assessment of the patient’s lung function should be obtained [25].
Lung resection is associated with the development of postoperative complications including pulmonary, cardiovascular, infectious, surgical, and others (Table 2.3). Some postoperative complications are associated with specific preoperative patient characteristics, permitting risk stratification. The two most common categories of complications, pulmonary and cardiovascular, are listed in Table 2.4 with their preoperative predictive variables. Aside from demographics, significant predictive ability is found in cardiac and pulmonary function. For this reason, specific evaluation of these parameters should be performed in all patients undergoing major lung resection regardless of the surgical approach [26].
Table 2.3
Classification of postoperative complications after lung resection
Category | Components |
---|---|
Pulmonary | Prolong postoperative intubation |
Reintubation for respiratory insufficiency | |
Prolonged postoperative air leak | |
Atelectasis requiring bronchoscopy | |
Pleural effusion requiring drainage | |
Pneumonia | |
Adult respiratory distress syndrome | |
Pneumothorax required intervention | |
Cardiovascular | Arrhythmia requiring intervention |
Myocardial infarction | |
Pulmonary embolism | |
Use of inotropic agents | |
Deep venous thrombosis requiring therapy | |
Cerebrovascular | Cerebrovascular accident |
Transient ischemic attack | |
Delirium | |
Surgical | Bronchopleural fistula |
Chylothorax | |
Recurrent nerve injury | |
Bleeding requiring reoperation | |
Other reoperation | |
Infectious | Emypema |
Wound infection | |
Sepsis | |
Other | Acute renal insufficiency |
Urinary retention | |
Postoperative transfusion |
Table 2.4
Preoperative parameters associated with complication categories
Complication category | Preoperative parameters |
---|---|
Pulmonary | FEV1, FEV1% |
DLCO% | |
Stair climb, shuttle walk, 6 min walk | |
Peak VO2 | |
Age | |
Smoking status | |
Induction therapy | |
Body mass index (BMI) | |
Cardiovascular | FEV1, FEV1% |
Age | |
Diabetes mellitus | |
Coronary artery disease | |
Renal insufficiency | |
Cerebrovascular disease |
2.2.3 Pulmonary Assessment
Physiologic assessment of lung function includes measurement of both ventilatory capacity and gas exchange capacity. Each of these is an independent predictor of postoperative complications, especially cardiopulmonary complications [27–32]. The most useful spirometric parameters are the forced expiratory volume during the first second expressed as a percent of predicted (FEV1%) and the predicted postoperative value for this parameter estimated based on the amount of lung to be resected (ppoFEV1%). Gas exchange capacity is assessed by measurement of the diffusing capacity of the lung for carbon monoxide (DLCO), which is an estimate of pulmonary capillary surface area or pulmonary capillary blood volume. The value is often corrected for hemoglobin, which provides a more accurate assessment of gas exchange ability. In contrast, corrections for lung volume are not typically performed, as the basis for such corrections are not physiologically sound. DLCO is usually expressed as a percent of predicted (DLCO%) or as an estimate of expected postoperative function (ppoDLCO%).
Predicted postoperative values are more accurate than preoperative values in estimating the risk of postoperative complications as well as long-term survival [33, 34]. Preoperative alterations in FEV1% may be related to underlying lung disease, neuromuscular disorders, prior lung surgery, extreme obesity, and other conditions (Table 2.5). Alterations in DLCO% may be related to serum hemoglobin level, primary lung disease, disorders of the pulmonary vasculature, and cardiac insufficiency. Predicted postoperative values of FEV1% and DLCO% usually can be accurately estimated using the functional segment counting technique [35].
The accuracy of such estimates can be affected by the location of the resected lobe (upper versus lower) and the presence of heterogeneous distribution of emphysematous changes [36]. In patients with heterogeneous lung disease, prior lung resection, or major airway obstruction, more accurate estimates are obtained using quantitative ventilation/perfusion scans or quantitative computed tomography [37–39].
Table 2.5
Causes of abnormal lung function tests
Parameter tested | Cause of abnormal result | Etiology |
---|---|---|
FEV1% | Chronic obstructive pulmonary disease | Decreased air flow |
Asthma | Decreased air flow | |
Interstitial lung disease | Loss of alveoli | |
Major airway obstruction | Decrease air flow | |
Chest wall disorders | Loss of lung volume | |
Severe obesity | Impaired diaphragm excursion | |
Prior lung resection | Loss of lung volume | |
Phrenic nerve dysfunction | Impaired diaphragm excursion | |
Dysfunction of respiratory muscles | Impaired air flow | |
DLCO% | Anemia | Low oxygen carrying capacity |
Polycythemia | Increased oxygen carrying capacity | |
Emphysema | Loss of pulmonary capillary surface area | |
Obstructive lung disease | Gas trapping | |
Interstitial lung disease | Increased alveolar wall thickness | |
Pulmonary edema | Increased alveolar wall thickness | |
Congestive heart failure | Increased alveolar wall thickness | |
Pulmonary hypertension | Increased vascular wall thickness | |
Low cardiac output | Low blood flow limiting gas delivery | |
Restrictive lung disease | Loss of pulmonary capillary surface area | |
Pulmonary embolism | Decreased pulmonary blood flow |

Exercising testing is also a useful method for evaluating patients’ postoperative risk after lung resection, as it assesses the interactive function of the respiratory and cardiovascular systems [40–45]. Although the routine use of exercising testing provides slightly more accurate risks of postoperative complications, its use is usually reserved for patients who are identified as being at increased risk based on pulmonary function testing. Selective use decreases the costs and duration of the preoperative evaluation. Exercise testing can be performed using low technology methods (stair climb, 6 min walk test, shuttle test) or using high technology testing, usually measurement of peak oxygen consumption during maximal exercise (peak VO2), which is typically performed during cycle ergometry. Exercise testing may not be possible in some patients because of lower extremity weakness, cardiovascular disease, or other physical limitations, and is often precluded because of the lack of availability the required equipment and expertise to use it.
2.2.4 Cardiac Assessment
The American College of Cardiology and the American Heart Association (ACC/AHA) guidelines stratify procedures by their level of risk and in turn identify patients who have clinical risk factors placing the patient at increased cardiac risk during non-cardiac procedures [46]. According to the ACC/AHA, major lung resection qualifies as having intermediate risk (a cardiac risk of 1–5 %). If patients are undergoing an elective lung resection, they should be evaluated and screened for severe or increasing angina, recent myocardial infarction (MI), decompensated heart failure, severe arrhythmias, or severe valvular disease. If any of these symptoms or history is present, the patients should have an appropriate cardiac work-up and management prior to an elective lung resection. If no history is present, patients are then evaluated for typical physical activity levels based on metabolic equivalents (METs). One MET is expended during effectively taking care of one’s self daily by dressing and feeding. Four METs are expended doing light work around the house or climbing a flight of stairs. If a patient meets the 4 MET threshold, the likelihood of important cardiovascular disease in the absence of symptoms is low and the operation can be planned. If less than 4 METs are typically expended or if METs are unknown, clinical risk factors of ischemic heart disease, heart failure, stroke, diabetes, and renal insufficiency are evaluated. If none is present, proceeding with the operation is appropriate. If one or more risk factors are present, a non-invasive stress test should be considered prior to scheduling an operation.
2.2.5 Perioperative Risk
2.2.5.1 Cardiac Risk Scoring Systems
The European Respiratory Society (ERS), the European Society of Thoracic Surgery (ESTS), and the American College of Chest Physicians (ACCP) recommend cardiac evaluation prior to lung assessment as recommended by the ACC/AHA. An index was developed to identify patients at high risk for complications in patients undergoing major noncardiac surgery [47]. The Revised Cardiac Risk Index (RCRI) identifies patients at increased risk for cardiac death, myocardial infarction, and cardiac arrest. The index is based on preoperative risk factors including a history of ischemic heart disease, congestive heart failure, cerebrovascular disease (stroke or TIA), the presence of diabetes mellitus requiring insulin therapy, the presence of chronic kidney disease with a creatinine >2 mg/dL, and planned high risk surgery including intrathoracic surgery [47]. Patients with 0, 1, 2, or more risk factors are divided into classes I, II, III, or IV respectively. This correlates with progressively increasing risks of major cardiac complications.
Recalibration of the RCRI to a system for risk estimation specific to lung resection was recently performed, resulting in the Thoracic RCRI (ThRCRI). The ThRCRI is a four-class risk score based on weighted values for serum creatinine, coronary artery disease, cerebrovascular disease, and extent of lung resection. Classes A, B, C, and D are assigned from the cumulative risk score, which correlates well with an increasing incidence of major cardiovascular complications [48–51]. This risk score also is predictive of the long-term risk of death in patients undergoing resection for lung cancer [52].
2.2.5.2 Cardiopulmonary Risk Algorithms
Algorithms for risk assessment and preoperative pulmonary evaluation were developed by both the ERS/ESTS and the ACCP [25, 45, 53]. Both recommend cardiac risk assessment for all patients; those found to be at increased risk should undergo a preoperative cardiology evaluation. The ACCP recommends pulmonary assessment of FEV1 and diffusion capacity for carbon monoxide (DLCO) with calculation of the predicted post-operative values (Fig. 2.1). If both the ppoFEV1% and ppoDLCO% are >60 %, no further testing is needed. If either the ppoFEV1% or ppoDLCO% is <60 %, but both are >30 %, a stair climb or shuttle walk test should be performed. If either of the predicted post-operative values is <30 %, a formal cardiopulmonary exercise test with maximal oxygen consumption assessment (peak VO2) is recommended. Those patients with <25 shuttles or <22 m climbed in low-tech testing should proceed to high-tech cardiopulmonary exercise testing with measurement of peak VO2. Patients with a peak VO2 >15 mL/kg/min do not have importantly increased risk. Those with a peak VO2 10–15 mL/kg/min are at increased risk, and a detailed discussion of the relative risks and benefits associated with surgery as well as alternative treatments should take place. Those patients found to have a peak VO2 of <10 mL/kg/min are at substantially increased risk and non-operative treatment should be seriously considered.


Fig. 2.1
The American College of Chest Physicians algorithm for preoperative cardiopulmonary function assessment [37]
The ERS/ESTS guidelines also rely on FEV1, DLCO, and measurement of peak VO2 [25, 45]. The ERS/ESTS guideline starts with assessment of a patient’s of FEV1 and DLCO (Fig. 2.2). If the values are >80 % of predicted, surgery can proceed without further testing. If one of the values are <80 % of predicted, then a measurement of peak VO2 is obtained. If this is >20 mL/kg/min, then the recommendation is for surgery. If it is <10 mL/kg/min, then non-operative management is recommended. If it is 10–20 mL/kg/min, then ppoFEV1 and ppoDLCO are reviewed. If both the ppoFEV1% and ppoDLCO% are >30 %, then it is reasonable to proceed with surgery. If one or both are <30 %, then surgery is only performed if the predicted post-operative peak VO2 is >10 mL/kg/min.


Fig. 2.2
The European Respiratory Society and the European Society of Thoracic Surgery algorithm for preoperative cardiopulmonary function assessment [29]
The main differences between the AACP and ERS/ESTS guidelines are that that the ERS/ESTS is quicker to obtain the measurement of peak VO2 and doesn’t utilize a stair climb or shuttle walk test. The AACP also recommends initially using the ppoFEV1% and ppoDLCO% while the ESTS guidelines utilize this later in the algorithm.
Utilization of these guidelines has been variable and a review of the compliance to the ERS/ESTS guidelines demonstrated that nearly 20 % of cases were non-compliant due to the omission of the exercise test [54].
2.2.6 Preoperative Risk Reduction
2.2.6.1 Smoking
A Society of Thoracic Surgeons database study evaluated the impact of patient’s smoking on perioperative risk [55]. Many patients undergoing lung resection are either current or past smokers. Perioperative mortality decreased in relation to the interval of smoking cessation. Major pulmonary complications were more frequent in current or past smokers [55]. Over time, smoking cessation mitigates these risks but no optimal time interval was identified.
2.2.6.2 Preoperative Physical Rehabilitation
Preoperative exercise therapy in lung cancer patients undergoing resection demonstrates mixed results [56]. Some studies demonstrate decreased hospital length of stay and pulmonary complications while others demonstrate no difference between the groups. Using the patient’s mean distance walked per day to calculate an estimated peak VO2, also including age and DLCO%, is more predictive of postoperative cardiorespiratory complications than the peak VO2 measured during a standard exercise test [57]. Due to a narrow preoperative window after cancer diagnosis, it is difficult to determine if an intense program will strengthen or simply fatigue those with poor preoperative conditioning. Furthermore, it is difficult to know if frequent outpatient sessions are feasible due to patient commitment, and inpatient rehabilitation is often cost prohibitive. Because of the limited and conflicting data surrounding preoperative rehabilitation, future research is needed in the area.
2.2.6.3 Frailty
Frailty is a clinical state with decreased physical function and low physiologic reserves [58]. It is the frail patient’s low organ system reserves, due to diseases, decreases activity, inadequate nutrition, and physiologic changes that are attributed to poor clinical outcomes. These poor reserves make physical compensation difficult in times of acute stress; like undergoing pulmonary resection. Assessment of frailty ranges from in-person encounters, usually in the form of an office visit, to a developed frailty index involving functional, medical, and cognitive health. More than 50 % of potential lung resection candidates are pre-frail or frail. Frailty is associated with adverse perioperative outcomes after lung resection; as an aging-frailty index increases, both morbidity and mortality increased incrementally [59].
There is increasing interest in identifying methods of mitigating frailty preoperatively, which theoretically may reduce perioperative risk. These include nutritional, pharmacologic, and physical interventions such as strength and/or endurance training. Early results demonstrate that physical interventions provide the most consistent positive results, and that results can be achieved in a time period suitable for planning lung cancer surgery [60].
Conclusions
Utilization of algorithms guide risk assessment and help quantify risk probability for an individual patient in the perioperative period. Research continues to change the assessment protocols of patients and their estimated risk. Algorithms not only aide surgeons in selecting appropriate patients for operations, but also allow patients to make informed decisions on risks and benefits prior to lung resection or alternate therapy. Future research is still needed in areas of patient optimization prior to surgery after cancer diagnosis as well as continued improvement in preoperative risk stratification for postoperative complications.
2.3 Staging and Selection of Patients for Minimally Invasive Lung Cancer Resection
(9)
Department of Cardiovascular and Thoracic Surgery, Rush University Medical Center, 1725 W. Harrison St, Suite 774, Chicago, IL 60612, USA
2.3.1 Introduction
Lung cancer remains the leading cause of death from malignancy worldwide [61]. Accurate staging of non-small cell lung cancer (NSCLC) is essential for prognostication and determination of the optimal treatment strategy. Proper risk stratification and patient selection is equally important; every patient must be assessed for both operability and resectability. The terms operability and resectability are often used interchangeably, however they represent different concepts [62]. Operability refers to a patient’s cardiopulmonary fitness and physiologic ability to undergo the required surgery. Alternatively, resectability describes the tumor characteristics and how that relates to the ability to achieve a curative operation. A given patient being considered for pulmonary resection can have any combination of operability and resectability.
All patients with lung cancer should be evaluated, staged, and treated in a multidisciplinary fashion, which includes input from thoracic surgeons, medical and radiation oncologists, pathologists, radiologists, and palliative care specialists [63]. The thoracic surgeon is an integral part of this process and is primarily responsible for the selection of patients for lung resection and technique in which it is performed. With rapid technologic advances instrumentation and increasing experience with advanced endoscopy and video-assisted thoracoscopic surgery (VATS), the range of operations able to be performed in a minimally invasive fashion is growing. There is emerging evidence that VATS may actually expand the patient population able to benefit from anatomic pulmonary resection [64–68].
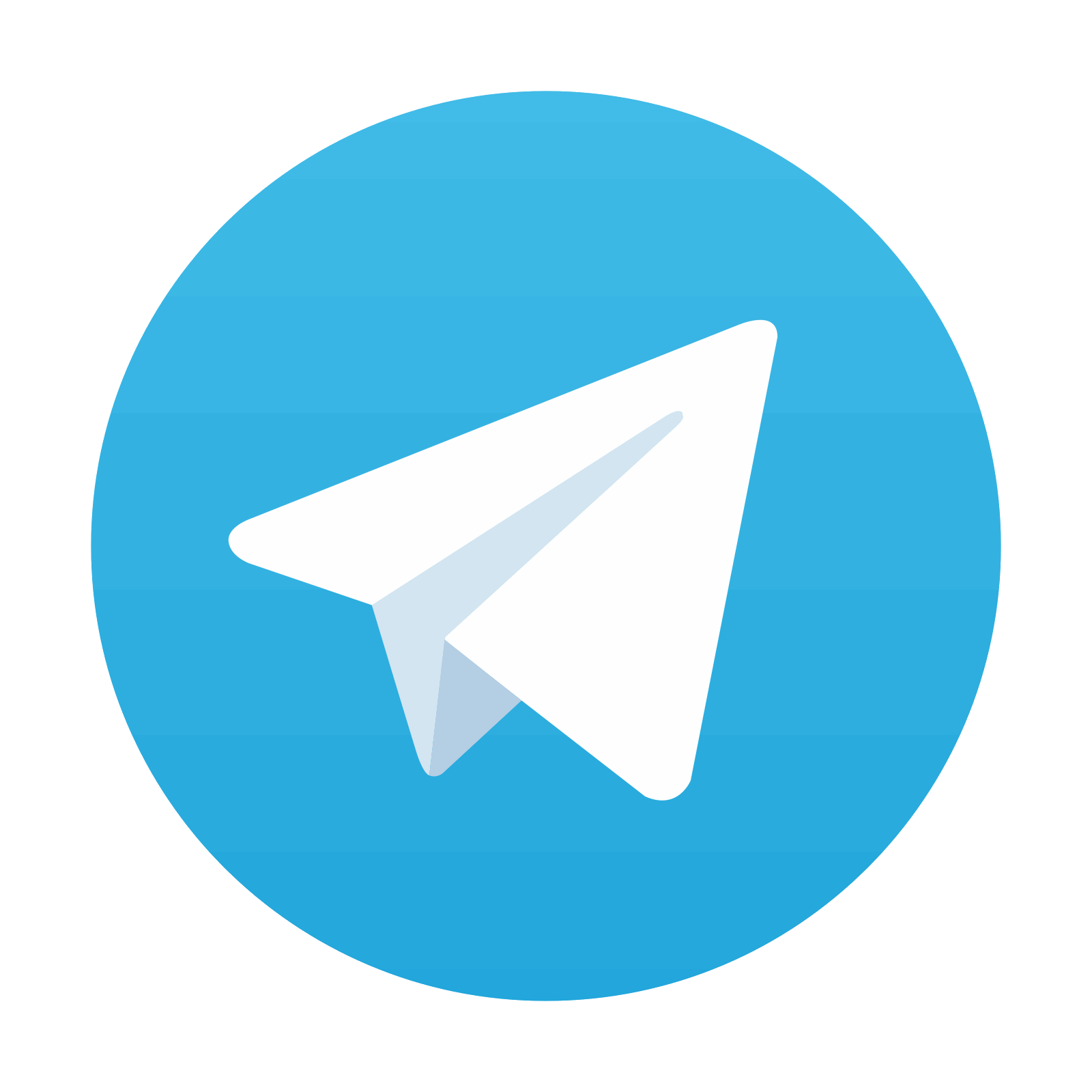
Stay updated, free articles. Join our Telegram channel

Full access? Get Clinical Tree
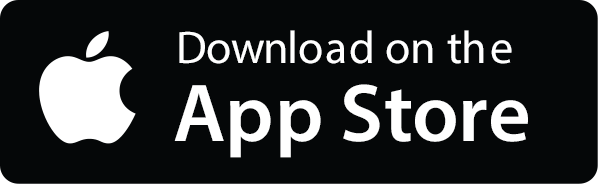
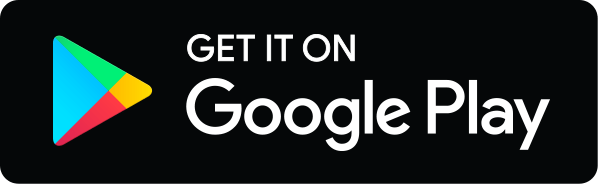