Summary
Despite the progress achieved in conventional treatment modalities, heart failure remains a major cause of mortality and morbidity. The identification of novel signaling pathways has provided a solid scientific rationale which has stimulated preclinical development of gene-based therapies for heart failure. Advances in somatic gene transfer technologies have been crucial to the advent of the first human clinical trials which are currently in progress. As these and other trials of gene transfer-based therapies are initiated, these approaches have generated excitement and hope for novel treatments for cardiovascular disease. In this review, we present a summary of advancements in construction of different vectors and methods of delivery that have been used for specific myocardial gene delivery. In addition, we will show results from studies focusing on the use of gene therapy to target heart failure mechanisms in animal models of cardiac dysfunction. Finally, we discuss the limited but highly promising results from clinical studies that have served as catalysts to translate preclinical achievements towards new treatment modalities for heart failure.
Résumé
L’insuffisance cardiaque demeure une cause majeure de mortalité et de morbidité malgré le progrès dans les modalités de traitement conventionnel. L’identification de nouveaux mécanismes de signalisation a formé une base scientifique solide pour développer le traitement génétique de l’insuffisance cardiaque. Le succès des nouvelles technologies de transfert génétique a été crucial pour l’initiation des premiers essais cliniques qui sont actuellement en cours. Ces derniers génèrent beaucoup d’enthousiasme et un espoir de découvrir de nouvelles percées thérapeutiques dans le domaine des maladies cardiovasculaires. Dans cet article, nous présentons un résumé de l’évolution dans la construction des différents vecteurs et des méthodes de livraison utilisés pour le transfert génétique au myocarde. Nous montrerons aussi des résultats d’expériences de traitement génétique dans des modèles animaux de dysfonctionnement cardiaque. Finalement, nous discuterons les premiers résultats d’essais cliniques qui promettent de lancer la course de la thérapie génétique dans le traitement de l’insuffisance cardiaque chez l’homme.
Abbreviations
AAV
adeno-associated virus
AC
adenylyl cyclase
β-AR
beta adrenergic receptor
GRK
G protein-coupled receptor kinase
HF
heart failure
I-1
(protein phosphatase) inhibitor-1
NCX
sarcolemnal sodium/calcium exchanger
PLN
phospholamban
PP
protein phosphatase
SERCA
sarcoplasmic/endoplasmic reticulum calcium ATPase
SR
sarcoplasmic reticulum
Despite the advances in conventional pharmacology, implantable devices and surgery, heart failure (HF) remains one of the leading causes of mortality in contemporary societies. There is an ongoing need to explore novel and more promising approaches targeting the HF pathobiology. Over the past decades, advancements in somatic gene transfer delivery have transformed cardiovascular gene therapy from a futuristic fantasy to a scientific reality.
This review focuses on the multiple applications of gene therapy in HF. The first part will serve as an overview of the principal types of vectors used in gene therapy. The following section will be a synopsis of the various methods of gene delivery. We will then discuss the different contemporary targets within the cardiac myocyte. The last part will present early results of the first in-human clinical trial (phase 1) of gene therapy in patients with HF. Far from being complete, this article emphasizes the most explored molecular techniques used in a field which, although remains in its infancy, promises to offer a breakthrough that might change the dismal history of heart failure.
Viral vectors for gene transfer
Classical models of gene therapy rely on recombinant protein expression. In contrast, RNA interference (RNAi) therapy is a novel technology that uses short regulatory RNA sequences to modulate gene expression as its basic principle. Successful use of those strategies for heart failure requires efficient myocardial transduction and long-term transgene expression. Viral vectors have been shown to be clearly superior to non-viral vectors such as plasmid DNA, liposome-DNA and polymer-DNA complexes. They consist of a genetic material surrounded by a capsid that interacts with specific cell surface receptors to allow binding, internalization and delivery of the genome to the nucleus of the target cell. In this part, we will briefly review the characteristics of the three most commonly used viral vector families which are adenovirus, lentivirus and adeno-associated virus. Table 1 summarizes the advantages and limitations of each one of these families.
Vectors | Adenovirus | AAV | Lentivirus |
---|---|---|---|
Functional titer (per mL) | Up to 10 12 | Up to 10 10 | Up to 10 9 |
Genome | dsDNA | ssDNA | ssRNA |
Insert capacity | 7 to 30 kb | 4.8 kb | 7 kb 10 kb |
Integration | No | Yes – chromosome 19 – wild type AAV No -recombinant AAV | Pseudo-random |
Pattern of transgene expression | Transient | Long-term | Long-term |
Cell-cycle dependent transduction | No | No | No |
Host/vector interactions | Cytotoxic and immunogenic | Minimally immunogenic | Minimally immunogenic |
Clinical trial approved | Yes | Yes | No |
Adenoviral vectors
Adenoviruses are double-stranded DNA viruses capable of carrying large genes of up to 30 kilobase pairs. They bind to the Coxsackie-Adenovirus receptors located on the plasma membrane and interact with cell surface integrins allowing viral endocytosis. Adenoviruses deliver and express their genomes within the nucleus of both dividing and non-dividing cells. Recombinant human adenoviruses are relatively cheap to produce in high titers and have a broad tropism to target cells especially within the cardiovascular system, which makes them widely used in myocardial gene therapy.
However, the use of adenoviruses is limited by a significant immune response that results in clearance of the transduced cells and transient gene expression lasting 10 to 14 days in animal models . The immune reaction destructs the cells enclosing the virus which in practice can result in adenoviral-induced myocarditis . Adenoviruses have also an innate tropism to multiple human tissues which can result in transfection of unintended host tissues. Multiple side effects including fever, chills, shivering, myalgias and even death are reported in clinical trials . Another major disadvantage is the presence of either preexisting or de novo formed antibodies that neutralize the adenoviral vectors limiting their transfection capacity.
Lentiviral vectors
Lentiviruses belong to the Retroviridae family. The most commonly used lentiviral vector is based on the human immunodeficiency virus type 1 (HIV-1). Lentiviruses have the capacity to transduce non-dividing cells, a feature required for targeting cardiovascular tissue. They can carry genes up to 8 kilobase in size. The efficiency of myocardial transfection is similar to adenoviruses but with longer duration of gene expression.
Due to the derivation from HIV, lentiviruses raised biosafety concerns that were addressed by modifying the native vector. The modifications include deletion of all the wild-type HIV accessory proteins and modification of the long terminal repeat (LTR) sequences of the vector . These adjustments render the manufactured lentiviruses unable to replicate and minimize the risk of gene expression through recombination of a wild-type LTR with the therapeutic vector.
Adeno-associated virus vectors
Adeno-associated viruses (AAVs) are single-stranded DNA agents, members of the Parvovirus family. They are non-pathogenic with minimal immunogenicity and sustained expression which might be related to their inability to infect antigen-presenting cells. AAVs enter cells by endocytosis after binding to specific cell surface receptors. They are capable of transfecting non-dividing cells similar to cardiomyocytes. There are 11 identified serotypes of AAV, of which AAV1, 6, 8 and particularly 9 have excellent tropism for the heart . The recombinant AAVs (rAAVs) used for gene therapy persist as episomal DNA in target cells instead of inserting into the human genome. This should render the risk of insertional mutagenesis from rAAVs negligible . On the other hand, one of the major disadvantages of AAVs is their limited genome packaging capacity of 4.7 kilobase. In addition, since AAVs have single-stranded DNA, they require synthesis of a complementary strand before transcription of the delivered gene can happen. Thus, the onset of gene expression is delayed and peak levels are attained between 2 and 4 weeks postmyocardial delivery . Moreover, human exposure to AAV capsid through natural infection or vector administration can result in production of all subclasses of Immonuglobulin G antibodies . Natural exposure to AAVs results in the presence of neutralizing antibodies (NAbs) against some serotypes in about 20–40% of the population. Those antibodies, together with those generated by the immune system in response to previous treatment with similar viral vectors limit the efficiency of gene therapy with AAVs .
Despite their relatively high cost of production, rAAVs are currently considered to be an agent of choice for myocardial gene therapy. To date, there have been more than 20 trials using AAV vectors including the first clinical trial of AAV1/SERCA2a in advanced heart failure, all of which found no unexpected safety concerns .
Methods of vector delivery
In addition to the major advances in engineering vehicles for myocardial gene therapy, the last two decades have also witnessed development of novel strategies for gene delivery that we will briefly discuss in this section. Catheterization has been used to target the myocardium by antegrade intracoronary injection, retrograde coronary vein infusion and by direct endomyocardial or pericardial injection. Surgical delivery to the myocardium or pericardium can also be used and might be the preferred route in certain clinical settings.
Antegrade injection
Antegrade injection in the coronary circulation is characterized by its capacity to homogenously deliver the vector to the myocardium. Agents increasing permeability of the vascular bed such as substance P, histamine or vascular endothelial growth factor, have been successfully used to improve the transduction efficiency of this technique . Percutaneous coronary venous blockade can also enhance gene delivery when performed simultaneously with antegrade coronary injection . In addition, the V-focus system is a recently developed percutaneous modality that separates the coronary and systemic circulations to establish a closed circuit between the coronary arteries and the coronary sinus. The procedure involves three percutaneous catheters, two of which to perfuse the right and left coronary arteries and a third catheter with a balloon to occlude the coronary sinus. The perfusate circulates through an extracorporeal membrane oxygenation system that provides adequate myocardial oxygen supply. The V-focus system delivers the vector almost exclusively to the myocardium, keeping in mind however that less than 10% of the coronary circulation flow continues to communicate with the cardiac chambers and the systemic circulation through the Thebesian veins.
Retrograde injection
Percutaneous retrograde delivery of vectors in coronary veins has been validated in large animal models and achieved gene expression comparable to antegrade delivery . The pressure resulting from antegrade flow is a limiting factor that is eliminated by simultaneous brief blockade of the coronary artery flow. Knowing that coronary veins remain mostly disease-free, the retrograde route is particularly useful when antegrade delivery is limited by a diseased arterial coronary circulation.
Direct injection
Direct injection of the vector into the myocardium can be achieved surgically or percutaneously. It overcomes numerous potential drawbacks that can be encountered with the intravascular route including the first-pass effect of the liver and spleen, the effect of neutralizing antibodies, the T-cell response and the impermeability of the endothelial barrier. Surgical gene transfer can be performed through a subxiphisternal or transthoracic approach and can be an attractive approach when the myocardium is easily accessible as during cardiothoracic surgeries. Catheter-based needle endomyocardial injection can be performed with several types of catheters and under different guidance modalities including fluoroscopy, electromagnetism and 3D mapping systems . Both the surgical and the percutaneous approaches have limited vector delivery secondary to the restricted area of injection and the leakage at the site of the myocardial injection .
Pericardial delivery
The pericardial sac is a closed space in close proximity to the myocardium and is accessible to both surgical and percutaneous delivery. Vectors in this space preferentially transduce the pericardial cells with minimal myocardial expression. Administration of collagenase and hylauronidase along with the vector can disrupt the pericardial cellular lining and extracellular matrix which improves myocardial transduction. This is however associated with a higher systemic contamination and local inflammatory changes . In addition to the surgical techniques, a percutaneous approach allows a safe and effective pericardial access appropriate for the delivery of therapeutic agents .
The optimal method of delivery depends largely on the proportion of target tissue to be genetically modified. Focal transduction might be desired to salvage an ischemic area whereas diffuse gene expression is more likely to reverse global myocardial dysfunction.
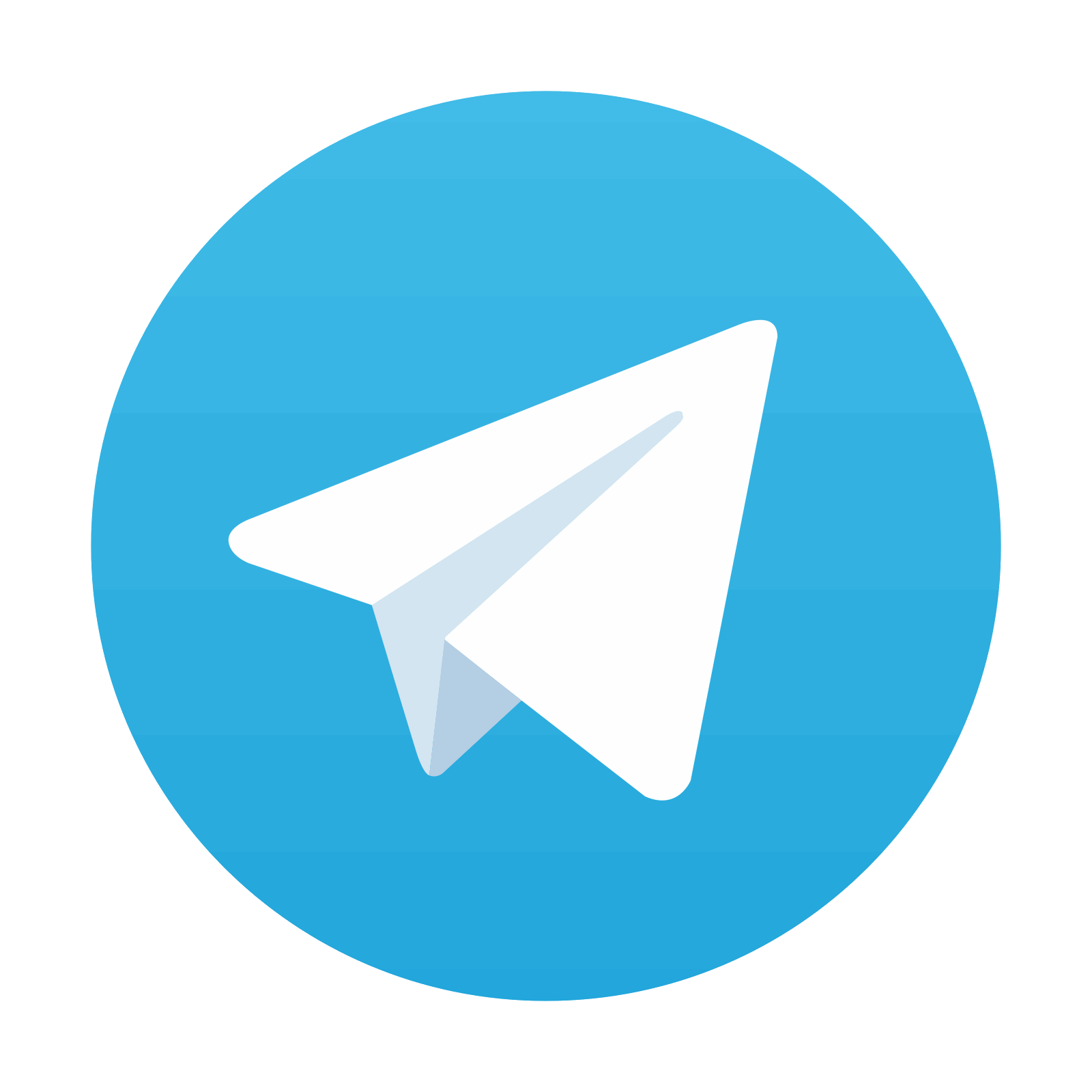
Stay updated, free articles. Join our Telegram channel

Full access? Get Clinical Tree
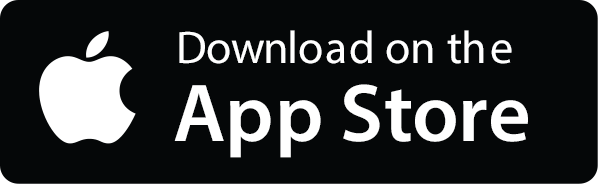
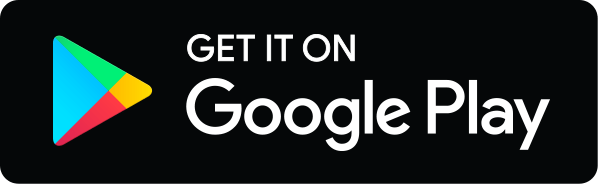