Fig. 8.1
3D Speckle-tracking applied to dobutamine stress echocardiography. Plastic bag and polar map views on the left belong to baseline acquisition, with normal LV function and normal regional values of 3D strain. Similar views on the right show acquisition at peak stress, with lower values of strain on apex and anterior/anterolateral wall due to ischaemia, and higher values on the rest of territories, as expected with dobutamine at high dose
Fusion of Coronary Multidetector Tomography and 3D Speckle-Tracking
Overview
In 2008, as explained on Chap. 6, the first 3D echocardiography equipment capable of performing three-dimensional analysis of myocardial deformation in all segments simultaneously from a single apical acquisition (3D-Speckle-tracking, 3DST) was validated. The three-dimensional imaging of the left ventricle with color coding of the degree of myocardial deformation, as volumetric representation or polar map, similar to Gated SPECT, has opened the possibility of performing hybrid imaging systems that combine these images with those obtained by MDCT. In 2013 a prototype of hybrid imaging software was introduced. The initial tests explored fusion between MDCT and 3DST rest echocardiography, which may be useful in the presence of regional contractility abnormality at rest (Fig. 8.2); however our group published the first case in which the hybrid image is used with images of 3D speckle-tracking within a pharmacological stress echocardiography protocol, in order to compare the 3D strain information at rest and at peak stress while assessing the relationship between these changes and the coronary anatomy [16]. See Fig. 8.3 for details. These hybrid images are obtained semi-automatically from the MDCT DICOM files and 3DST echocardiography, through the use of common landmarks that the system easily recognizes. In case of finding inaccuracies the overlapping images can be manipulated for better alignment (Fig. 8.4).




Fig. 8.2
Fusion of rest 3DST echocardiography and MDCT. The area of low contractility at the apex and anterior wall could easily be interpreted as a sign of LAD disease. The hybrid view made clear that it was related with a sub-occluded Diagonal branch

Fig. 8.3
First case ever documented of stress 3DST fusion with MDCT. A patient with three vessels disease underwent dobutamine stress and a large area of septal, apical and anterior ischaemia was assessed. The hybrid view showed the relationship of this area with the LAD artery. Images A/B belong to the baseline study and C/D belong to the peak stress

Fig. 8.4
Overlapping of MDCT and ultrasound images of the left ventricle for fusion
Hybrid Imaging Modalities
Volumetric 3D reconstruction (Fig. 8.5). It shows the aortic root, the coronary tree and left ventricular volumetric images that can be viewed as still images or moving throughout the cardiac cycle. Different color codes represent the magnitude of the deformation parameter chosen (usually 3D strain, but the representation of the radial, longitudinal strain, area-strain, etc. can be chosen). In the most common encoding, the dark blue areas correspond to regions with hypokinesia, and areas of yellow color mark segments with greater contractility. The visualization of the coronary arteries and the LV strain at the same time allows to visually check the “matching” between the coronary stenosis and hypokinetic regions.
Polar map or bullseye view (Fig. 8.6). It shows 16 or 17 myocardial segments (depending on the selected model) with the average strain in each segment, and the middle-distal segments of the coronary arteries superimposed on them. The color code of the polar map is the same as for encoding the LV volumetric reconstruction. There is also an anatomic coronary territories map (Fig. 8.7) that depending on the size and shape of the arteries of each particular patient, sets three areas that would belong to Left Anterior Descending, Circumflex and Right Coronary artery. This approach may be more useful that the usual assignation of certain myocardial segments to certain coronary arteries. Moreover, the average strain for each area can be shown, and the increase or decrease of strain during stress testing can be quantified. If this system is validated in the near future, quantitative stress echocardiography may be a reality.

Fig. 8.5
Volumetric 3D reconstruction of the aortic root, coronary arteries and left ventricle. A critical stenosis of the distal LAD matches with an area of low 3D strain at the apex

Fig. 8.6
Polar map with myocardial segments, coronary arteries and radial strain color coding in a patient with prior myocardial infarction involving occlusion of the CX. Fusion was performed after stenting. The dark area on the anterolateral and inferolateral wall matches with the CX territory

Fig. 8.7
Anatomic coronary territories in a patient with three vessels disease and ischaemic response on dobutamine stress. This modality of polar map shows the distribution of myocardium in relation with each coronary vessel, and graphics show curves of 3D strain for each territory. At baseline (left figures) strain is normal all over the surface of the polar map, and curves show high proportions of strain for the three territories; at peak (right figures) an area of low strain appears at the apex with extension to both LAD and RCA territories. The graphics show a decrease of strain in LAD and RCA related territory and an increase in CX territory
Advantages of the Hybrid TC + 3D-ST System
The advantages of the combination of coronary anatomy and functional assessment have been already tested with SPECT/PET and MDCT fusion, as explained in the first section of this chapter. The simultaneous assessment of both aspects of the disease on the same image provides an incremental value over the separate interpretation of both tests. This information may influence the decisions of physicians, and the matching of anatomic and functional findings correlates with prognosis. The new strategy of using echocardiography has an obvious advantage over SPECT or PET and is the absence of harmful radiation. With the same coronary tree as reference, several studies may be compared in the follow-up of a patient without additional radiation.
Limitations of the Technique
The inclusion of echocardiography as a functional component of these hybrid systems instead of perfusion techniques such as PET or SPECT has the limitation on not assessing perfusion directly, but the impact of such perfusion on cardiac mechanics. Also, 3DST technology allows for spectacular images thanks to automated endocardial and epicardial border detection, but the accuracy of these systems is highly dependent on the quality of the ultrasonic window. When this is not optimal, manual corrections are often needed, and the reproducibility of the studies may be not as good.
In addition, the frame rate of these studies (usually around 20–25 vps), is a major limitation, especially when used in patients with elevated heart rates, as it occurs during exercise or pharmacological stress, which is precisely the moment when the hybrid image has more clinical utility. However clinical validation studies of the system that are ongoing will provide the real clinical utility of the technique.
Future Directions
One line of research is the emerging application of the hybrid system for visualizing coronary veins in combination with image speckle-tracking, in this case fusion is aimed at locating the segments with the latest activation of contraction and their relationship with a particular coronary vein (Fig. 8.8). A clinical case applying this approach has been already published [17]. The hybrid image in this context might be helpful for locating the most appropriate vein for left ventricular pacing in cardiac resynchronization therapy.


Fig. 8.8
Fusion of coronary veins MDCT and 3DST-derived radial strain. The left image shows the coronary veins and the left ventricle at late systole, showing the area of latest activation in yellow color (lateral wall). The opposite wall (septal), with earlier contraction, shows negative strain at that moment and it is colored in blue. The right image displays the polar map at the same frame. The differences between both areas suggest dyssynchrony. The posterolateral vein that emerges just from the latest contraction area, would probably be the appropriate one for placing the lead if resynchronization therapy was applied
It is also expected further development of 3DST technology for increasing image quality, reliability of the automatic detection and tracking of endocardial and epicardial borders, and the frame rate. Additionally, a system with vendor independence allowing to use data from different 3DST systems would increase the clinical applicability on daily practice.
3D Echocardiography and Fluoroscopy: Echo Navigator
Minimally-invasive cardiovascular interventions are becoming wider and wider spread because of their demonstrated effectiveness and safety. Two-dimensional (2D) X-ray imaging used to be the dominant imaging modality for guiding this kind of cardiac procedures. It offers some advantages, such as its high definition of catheters, valves and metallic and calcified structures, as well as its good temporal resolution. However, fluoroscopy has also some pitfalls. It is inadequate to visualize soft tissues, only provides 2D imaging and implies contrast and radiation.
That is why echocardiography has turned into an essential tool inside the Cath Lab. It has all the attributes that fluoroscopy lacks: it is a non-radiation, non-contrast technique, that allows continuous soft tissue imaging. Moreover, if implemented, transesophageal echocardiography (TEE) and 3D echocardiography provide better understanding of morphology and spatial relation of intracardiac structures than transthoracic and 2D echocardiography (TTE) [18].
During interventional procedures, fluoroscopy for catheter and device visualization and echocardiography for anatomy and soft tissue imaging are most frequently used. But up to now, none of them offered a simultaneous evaluation of both types of structures, thus requiring several screens and extensive effort of coordination and communication between imagers and operators.
Recently, a new navigation system (EchoNavigator, Philips Healthcare, Best, the Netherlands) has been introduced which synchronizes echocardiography and fluoroscopy in real-time. This new imaging technology seeks to improve the communication between the echocardiographer and the interventionalist, to increase the confidence and anatomical awareness, to assist in guidance, and to increase procedural efficiency [19, 20].
The EchoNavigator places the two imaging modalities in the same coordinate system and is based on the localization and tracking of the TEE probe. After synchronization of TEE and fluoroscopy images, the system automatically tracks and follows the movements of the c-arm gantry. This way, if the c-arm is moved, echocardiography images are updated and reconstructed with the same angulation, allowing rapid understanding of the anatomy and the relation between cardiac structures and devices during complex interventions.
Furthermore, Markers (dots or crosses) can be set to highlight important structures on the echocardiography image, and they are automatically displayed and updated in real time on the fluoroscopy image. It appears particularly useful for guiding complex catheter or device manipulations with the target of the displayed marker, potentially reaching an important reduction of time and radiation.
In a more practical focus, we are going to describe how the usual performance of this technology is, how the images are presented and what its main clinical applications are.
At the beginning of a procedure the TEE probe is co-registered by the acquisition of two angulated fluoroscopy projections [21]. Once the algorithm recognizes the TEE probe and its orientation, the TEE and x-ray images are combined and can be manipulated.
The system offers a four image display (Fig. 8.9) usually displaying the following views: (1) the fluoroscopy view; (2) the c-arm gantry view, in which the echocardiographic view is oriented in the same plane as the x-ray view (and automatically updated by each movement of the c-arm); (3) the echocardiographic view which is the standard TEE projection showing up on the echocardiographer’s screen; and (4) a free image that can be rotated or cropped.


Fig. 8.9
Echo-Navigator. Whole screen with four simultaneous real-time imaging modalities during mitral prosthesis leak closure. Two upper images from 3D TEE show different points of view for showing the interventional cardiologist the situation of the leak and its relationship with the surrounding structures. On the right inferior image, the fluoroscopy view matches with the pyramidal 3D echo display on the left inferior display, and both images are synchronized for moving together when the X-ray arm is being moved
Therefore, EchoNavigator allows the physician (usually very experienced in the use of fluoroscopic images but less so in using ultrasonic images) to use the more intuitive perspective of fluoroscopic images but with the additional information, specifically 3-D soft-tissue visualization, provided by echocardiography. Major spatial transformations such as the mental rotation of ultrasound images displayed in an unfamiliar perspective, no longer have to be performed by the physician after the automatic registration of ultrasound with x-ray and the display of the modalities in a familiar perspective [22].
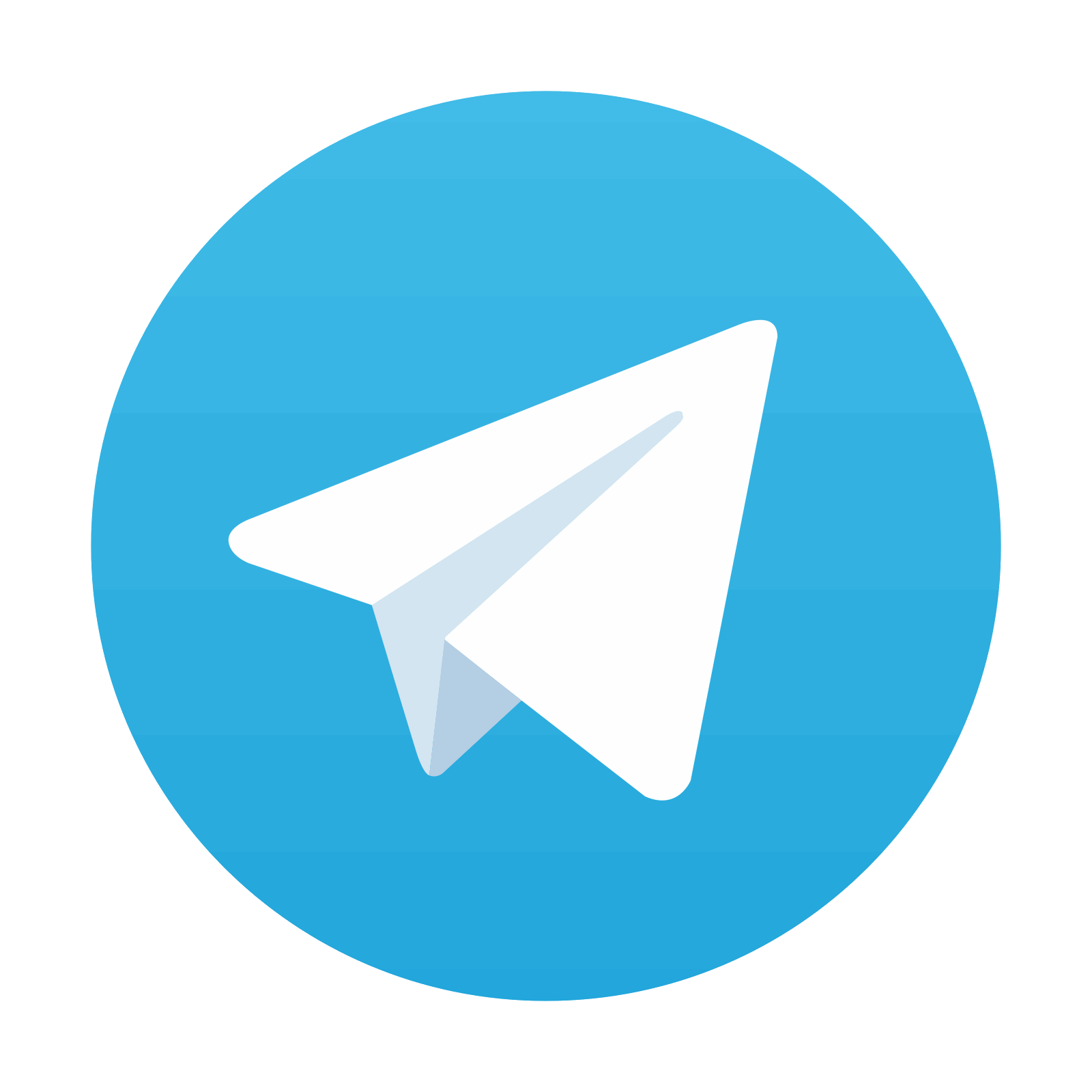
Stay updated, free articles. Join our Telegram channel

Full access? Get Clinical Tree
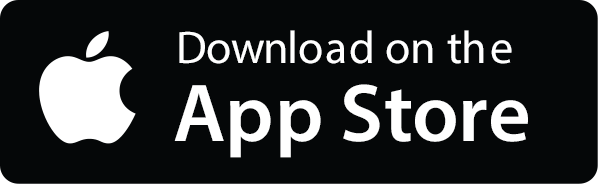
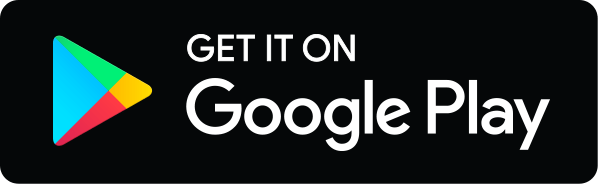