Fig. 6.1
Windows on the left (a–c) demonstrate the process of identification of different cardiac structures by applying the designated color mesh on the standard anatomical planes (axial, sagittal, and coronal) [5]. A three-dimensional model with markers placed on the location of paravalvular leaks (d, white arrows) and metallic valve (d, blue arrow) are shown
6.3.2 Image Acquisition—Echocardiography
The acquisition of 2D and 3D datasets is an important aspect of using fusion imaging for PVL closure. Acquisition is done live at the time of the procedure which can then be fused with live fluoroscopy. We describe it in this section for continuation. For acquiring high-quality and relevant images, the 3D fully sampled matrix array TEE transducer (3D–MTEE) has become integral [6]. It has approximately 2500 elements, in contrast to the 64 elements in the multiplane TEE probes [7]. With this transducer several 3D echo protocols or imaging modes are routinely used; (1) The “xPlane mode” displays two images, one is the reference 2D image and the second a rotated view of the reference image which can be manipulated (rotated or tilted) (2). The “real-time narrow-angle mode” displays a narrow pyramidal dataset with excellent spatial and temporal resolution; however cardiac structures cannot be fully imaged (3). The “3D–zoom mode” displays a wider sector view of the region of interest which can be rotated in all directions. This mode is useful for guiding catheters/wires and visualizing devices. However, it suffers in temporal resolution (4). In the “full-volume ECG-gated mode,” a full-volume 3D dataset and 3D full-volume color Doppler are obtained over four heartbeats and stitched together. This can then be manipulated online or offline in dedicated software [6]. The full-volume mode is useful to identify the location of the PVLs but may be limited by poor spatial resolution and stitch artifact [8–10]. With the use of the above modes, PVL can be visualized in multiple views; e.g., the mitral PVL can be visualized both from the surgical view and the ventricular view, thus guiding catheters and wires. The color Doppler helps in locating PVLs pre- and interprocedurally. All the above modes can be used in the echo-fluoroscopy fusion imaging.
6.3.3 Identification of PVL and Virtual Planning
The value of tailoring preprocedural planning for PVL closure for each patient cannot be understated. It includes evaluation of the anatomical location, number, extent, course, severity, and surrounding structures of PVL. The post-processing of CTA data is performed as described above facilitating annotation of relevant structures during or prior to the procedure.
MDCT combined with echocardiography can determine the size, shape, course, and the surrounding cardiac structures of the PVLs [11, 12]. (Figs. 6.1d, b) In addition, valuable information on the prosthetic valve structure (pannus formation, leaflet thickening, adjacent calcification, pseudoaneurysm, etc.) and function (abnormal leaflet mobility) can be obtained [13, 14]. Two-dimensional and 3D color Doppler helps with localizing the PVL and when fused with fluoroscopy can help with precise steering of catheters and wires.
Virtual planning can be done by post-processing pre-acquired CT data (Fig. 6.2). A virtual line joining the skin entry, LV entry, and PVL can be drawn which then can be fused with fluoroscopy. In addition, virtual implantation of various devices can be simulated on the model which may help the interventionalist in predicting the size and the number of devices that may be required (Fig. 6.2c).


Fig. 6.2
Virtual PVL closure planning. The safe path from the skin puncture site to the location of PVLs at different locations can be virtually placed (a, b, white arrows). On the 3D segmented model, virtual models of the Amplatzer vascular plugs (c, asterisk) are placed to examine the possible size and interactions with surrounding structures including prosthetic valve (c, blue arrows)
6.4 Principles of Fusion Imaging
Fusion image can be defined as a composite synergistic image where the most relevant information datasets from two or more different imaging modalities are combined in a comparable scale and displayed as a new image. Fusion imaging does not provide new information, but it enhances it in a more relevant manner. Fusion imaging is being used in multiple specialties of medicine. One such example is the single photon emission computed tomography (SPECT) and CT fusion which is used for evaluation of coronary disease, localizing infection (white cell infection imaging), orthopedic/sports injury imaging (bone scan SPECT-CT), etc. The ability to merge these images provides a more accurate identification of the anatomical location and extent (from CT) of the abnormalities highlighted on SPECT nuclear medicine scans. In performing complex structural heart procedures like PVL closure, merging and projection of a live or preprocessed image (typically echocardiograph or CTA) over another image (typically live fluoroscopy) is used (Fig. 6.3).


Fig. 6.3
Schematic of fusion imaging for PVL closure. Pre-acquired CTA/MRI data (post-processed) and 3D TEE data are fused with live fluoroscopy for CTA-fluoroscopy fusion and echo-fluoroscopy fusion
6.4.1 Echocardiography-Fluoroscopy Fusion
The initial step of the fusion process is co-registration of two distinct images, which is to orient one image (images from echocardiography) to another image (fluoroscopy). Echo-fluoroscopy fusion relies on real-time co-registration which involves spatially orienting echocardiographic image to match fluoroscopic image. This can be automatically performed by the EchoNavigator® system which uses TEE probe localization and calibration algorithm for co-registration (Fig. 6.4). It relies on rapid, automated identification of TEE probe tip during live fluoroscopy. The TEE transducer is housed in a plastic shell and has a characteristic signature on X-ray projection, which changes on probe position and angulation predictably [15].


Fig. 6.4
TEE probe registration is performed automatically when the probe is in the X-ray field of view by stepping on the X-ray pedal. When registration is successful, a check mark is visible, and the probe is displayed in green (a); transparent, registration timed out (b); red, unsuccessful (c); in special cases registration could be performed manually (d) by adjusting the contour position till it becomes green
It is able to automatically tract the position and the direction of the TEE probe and overlay chosen 3D echo imaging mode on the fluoroscopic image [16]. Subsequently, the system tracks and follows the rotation of the C-arm and synchronizes the echo image with fluoroscopic image. Several different echo views can be displayed by the system (Fig. 6.5); (1) The echo view shows images that are seen by the echocardiographer (2). The C-arm view shows the TEE field of view (i.e., echo cone) as a purple sector along with corresponding TEE images. (3) The free view allows additional post-processing capability like reorientation, cropping in any plane of interest [15].


Fig. 6.5
Work windows of EchoNavigator. (a) The free view where the 3D image can be manipulated with active cut plane tool (red plane) allowing cutting the 3D reconstructed volume and free 360° rotation. (b) The standard 3D view controlled by 3D TEE operator. (c) A 3D–view orientation defined by the position of TEE probe (green) is shown. (d) The C-arm view which shows the fusion of the 3D TEE volume (purple sector) over the live fluoroscopy
6.4.2 CTA-Fluoroscopy Fusion
Co-registration between CTA and fluoroscopy can be achieved by using existent radiopaque markers (i.e., prosthetic valve metal frame, pacemaker wires, calcification, etc.) or by registering with contrast aortography (Fig. 6.6). Once the co-registration is achieved, the overlaid CTA image and markers move with real-time fluoroscopy providing a 3D orientation of the cardiac structures during procedures.


Fig. 6.6
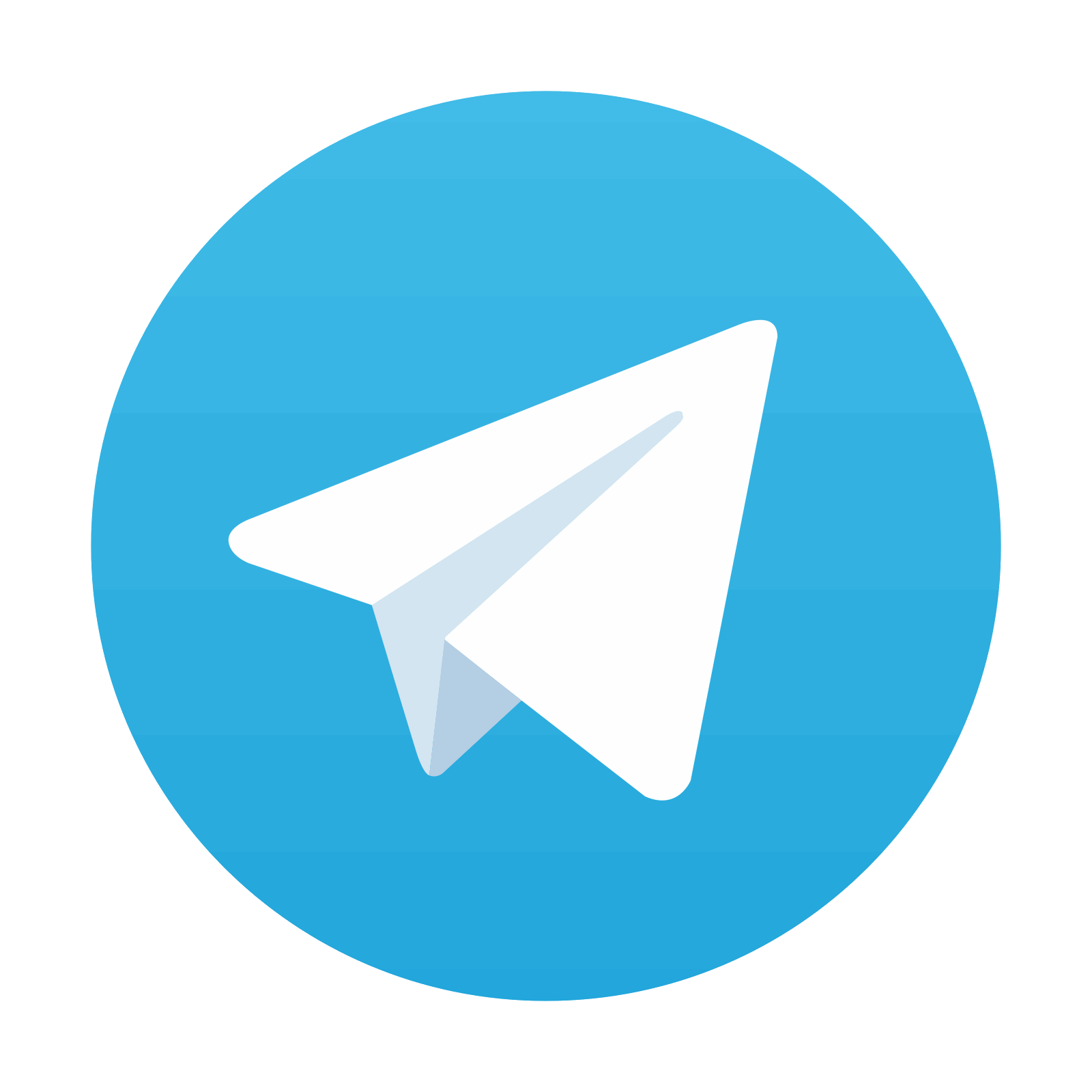
Registration of CT segmentation over the live fluoroscopy. Registration is achieved by manually adjusting the segmented prosthetic valve (a, blue color) over the prosthetic valve visible on fluoroscopy. Two orthogonal planes at least 20° apart (a: RAO 29° and LAO 38°) are used to move the segmented prosthetic valve to overlay the position seen on the fluoroscopy (a). After the desired positioning is achieved and accepted, the 3D model of the cardiac structures with target markers placed during preprocedural planning will follow the C-arm position and will help to guide the intervention by displaying the precise location of the defects in 3D space (b)
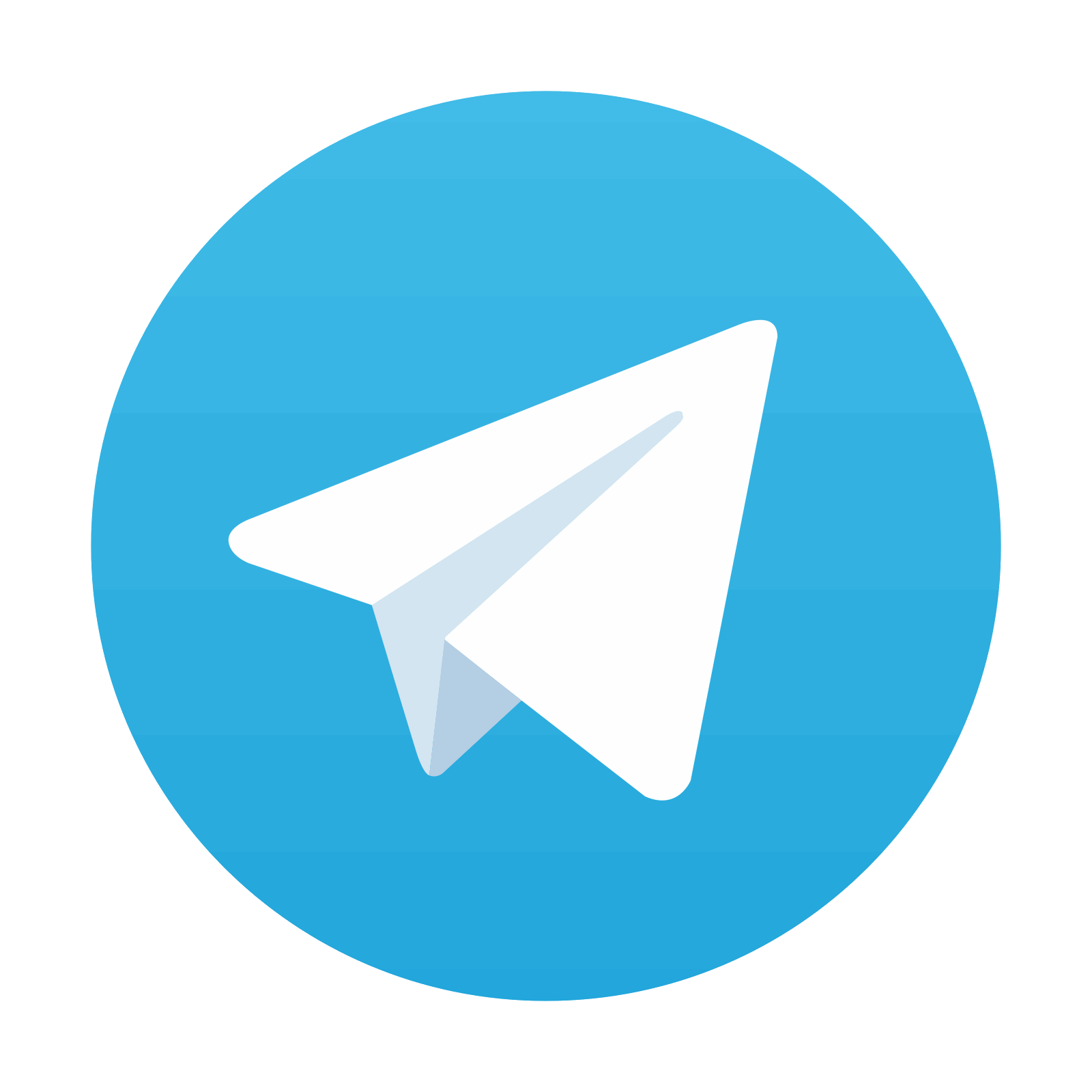
Stay updated, free articles. Join our Telegram channel

Full access? Get Clinical Tree
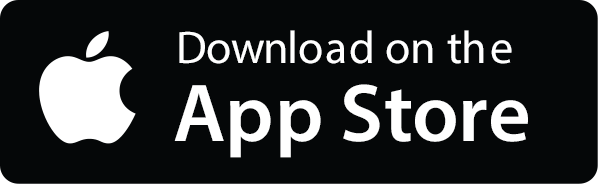
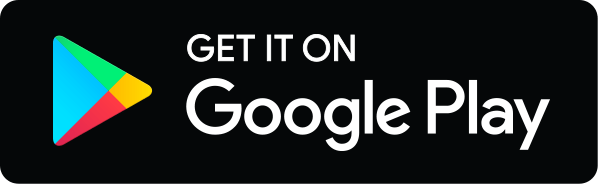
