, Germaine Cornelissen2 and Franz Halberg2
(1)
Department of Chronomics & Gerontology, Tokyo Women’s Medical University Medical Center East, Arakawa-ku, Tokyo, Japan
(2)
Halberg Chronobiology Center, University of Minnesota, Minneapolis, MN, USA
Abstract
Franz Halberg (1919–2013) developed chronobiology and founded the field of chronomedicine including chronomics, chronoastrobiology, and chronobioethics. His work is summarized in his over 3,600 scientific publications, in cooperation with colleagues from around the world. Progress in chronomedicine depends on how his research developed.
Several international meetings have revealed an accumulating body of reference values for well-established about-daily and about-yearly rhythms of photic origin. Evidence was also provided for about-7-day, about-27-day, about-half-yearly, about-10.5 and about-21-yearly, and even about-50-yearly rhythmicities in us as well as around us, as invisible nonphotic heliogeophysical signatures possibly built into individuals and/or populations. In time series, biological or other, the characteristics of rhythms, chaos, and trends can all be quantified as elements of structures, called chronomes. Chronome mapping with outcomes could eventually serve an individualized optimization of lifestyle, for chronoprevention and chronotherapy as well as for inquiries into the evolution and future of life. The historical path of Franz Halberg’s research leading to chronomedicine is presented herein.
Keywords
ChronotherapyRemove-and-replace approachChronomeChronomicsEnvironmental counterpart2.1 Back to My First Encounter with the Adrenocortical Cycle
At only one time in history, the earliest 1950s, adrenalectomy could be carried out on patients [1; cf. 2], in the wake of the euphoria from cortisone, making lame people walk again. Thus, a remove-and-replace approach taught us (Fig. 2.1) that the 24-hour rhythm in serum iron was altered but persisted (ACI: adrenocortical insufficiency), while the rhythm in circulating blood eosinophil counts [1] (and in phospholipid labeling and cell division of adrenalectomized mice) seemed to be eliminated or altered by removing the adrenal (in a 2-timepoint approach) [1]. Kaine et al. [2] found that a periodic administration of corticosteroid re-induced the rhythm in humans without adrenals.


Fig. 2.1
Chronobiologic self-experimentation extended to groups of medical students and staff served to find that the adrenal glands are an important source of circadian rhythmicity in the circulating eosinophil count. For instance, a circadian component of variation could not be demonstrated for eosinophil counts of patients with adrenocortical insufficiency (bottom), whereas in healthy people with either restricted or unrestricted activity, this rhythmicity was not only demonstrable but also amplified by enhanced motor activity. Whereas for patients with Addison’s disease, a circadian rhythm in eosinophil counts could not be statistically validated, circadians persisted, albeit altered, for the case of serum iron (P < 0.001) (top). As subsequently observed in the case of bilateral, histologically validated ablations of the suprachiasmatic nuclei, some rhythms persist; others may be altered but are not lost in remove-and-replace approaches studied thus far (© Halberg Chronobiology Center)
2.2 Life or Death from the Same Stimulus, Including Drugs: Chronotherapy
Circadian susceptibility rhythms accounting for the difference between survival and death from the same stimulus, e.g., bell ringing, by 1955, and then X-irradiation in mice [3] and a long series of drugs, became the basis of chronotherapy. By 1977, with B.D. Gupta and Akhil Deka, the 2-year disease-free survival rate of patients with perioral cancers (receiving radiation treatment at the time of their peak tumor temperature) could be doubled, Fig. 2.2a, b [4–6]. Susceptibility underwent rhythmicity along both the 24-hour and the 7-day (circaseptan) scale in vivo and in vitro (Fig. 2.3), in the hands of Waldemar Ulmer, who accounted for the circaseptan cycle at the atomic [7] and quantum [8] as well as macroscopic levels, the latter on the right of Fig. 2.3, while therapeutic benefit from treating at maximal β-ATP used as a marker is seen in the middle of this figure (in vitro).





Fig. 2.2
(a) Tumor regression rate is circadian stage-dependent in response to X-irradiation (© Halberg Chronobiology Center). (b) Treatment timing at peak tumor temperature leads to faster tumor regression (left) and to more than doubling of the 24-month disease-free survival rate (0 on abscissa, right), as compared to reference groups treated 4 or 8 hours before or after the tumor temperature peak (+8, +4, −4, −8 on abscissa, right) or “as usual” (last column, right). Studies implemented by B.D. Gupta and Akhil Deka, designed during a PL-480 program sponsored by the USA (© Halberg Chronobiology Center)

Fig. 2.3
Circaseptan optimization in a 9 L glioma rat tumor model may be even more important than circadian chronotherapy (© Halberg Chronobiology Center)
2.3 Cellular Timing
By 1958, a combination of methods, including radioactive tracers, differential centrifugation, and wet chemistry, enabled the demonstration of circadian rhythms in the formation of RNA and DNA [9], with RNA synthesis preceding that of DNA, and growth hormone acting in just one stage of this first mapped circadian cell cycle [10, 11]. In 1960, this cell cycle was reported and depicted as a basic complement to the hypothalamus, Fig. 2.4 [3], as accepted today but denied at the time and for several decades thereafter. Thus, against the prevailing bias of the vast majority of a Naito meeting published in 1979 [12], it was reemphasized that circadian timing resides in peripheral cells as well as in the suprachiasmatic nuclei (SCN) of the hypothalamus [13, 14]. Unless the data were interpreted by visual inspection alone [15, 16], SCN removal (as in the case of adrenal removal) eliminated some rhythms (in motor activity and water drinking) but not those in core temperature, alcohol drinking, corneal mitosis, or thymidine labeling in the gastrointestinal tract, Fig. 2.5a, b. A report on the loss of the circadian rhythm in core temperature was revised when the data were reanalyzed [15 vs. 16]. An amplification of thymidine labeling was found in the stomach after bilateral SCN ablation, Fig. 2.5b, and in core temperature after unilateral ablation, Fig. 2.5a (lower row), the latter finding interpreted as subtractive coupling between the two nuclei [13].




Fig. 2.4
Sketch of factors and pathways known or hypothesized to participate in frequency synchronization among circadian rhythms themselves, as well as in synchronization between rhythm(s) and environmental synchronizer(s). Emphasis upon the cell as a documented source of rhythmicity by 1960 [3, 9] (© Halberg Chronobiology Center)


Fig. 2.5
(a) Much controversy has revolved around the circadian rhythm alteration after lesioning of the suprachiasmatic nuclei (SCN). Several investigators have claimed, without cosinor analyses, that all circadian rhythms are obliterated after bilateral lesioning of the SCN; others including ourselves have found, with cosinor analyses, a rhythm alteration to a differing extent and in different directions (amplification as well as damping) for different variables rather than an overall obliteration after such intervention. Evidence supporting the persistence of rhythms after bilateral lesioning of the SCN illustrates the merits of chronobiometric analyses to obtain a valid interpretation of the data. This figure shows the circadian temperature patterns in Fischer rats, without or with unilateral or bilateral lesions of the SCN. A visual inspection of the original core temperature data (IIA) may erroneously convey the impression that rhythmicity clearly seen in the series on the left is abolished in the series on the right. The use of simple chronobiologic techniques such as a plexogram (i.e., the stacking of the data over an idealized anticipated cycle of 24 hours) shows that there is a persisting circadian pattern, albeit an altered one, with a reduced amplitude and an advanced phase, IIB and C. This is also shown in curves obtained from several different animals (IC and D). Whereas the circadian amplitude of core temperature of rats with a histologically validated bilateral lesioning of the SCN is much reduced, it is noteworthy that in rats with a unilateral lesion of the SCN, the amplitude is actually larger than that of sham-operated rats. Evidence supporting the influence of planetary-interplanetary phenomena is provided by the fact that sham-operated rats and rats with a unilateral lesion of the SCN kept in continuous light of low intensity have a circadian period of core temperature that is desynchronized from 24 hours, with an average period close to the “lunar” day (or rather one of the earth’s double tidal periods); this is not the case for rats with a bilateral lesioning of the SCN which can have either a shortened or lengthened circadian period (III) (© Halberg Chronobiology Center). (b) Persistence of the circadian rhythmicity of the 3H-TdR incorporation into DNA of different organs and of the mitotic index of the corneal epithelium of BD2F1 female mice after bilateral lesioning of the SCN has also been extensively demonstrated by Scheving (I-VI) [14] and Pasley (VI and VII). For animals with a bilateral lesion of the SCN, a cosinor on Pasley’s data could not validate a circadian rhythm in the drinking of water, but demonstrated it (P = 0.002) for the drinking of 5 % ethanol (VI and VII). The SCN is not the sole or primary pacemaker of the entire circadian system (© Halberg Chronobiology Center)
Along a different line of thought, removal of the SCN suggests its mediation of earth tidal effects by the loss of the 24.8-hour (double tidal) period in core temperature of rats kept in continuous light of low intensity, Fig. 2.5a-III. This 24.8-hour component was also found in the self-selected sleep pattern of a healthy man during 3 years, Table 2.1, and in the longest (>260-day) human isolation in a cave study [17, 18], Fig. 2.6, among many others, shorter but important studies of human isolation in shielded and unshielded bunkers by Ruetger Wever [19]. He recorded periods that could have been interpreted, as close to 24.8 hours.

Table 2.1
On a self-selected schedule (JFC), a double tidal pull competes with 24-hour environment while initially coexisting free-running circadian fades (global analysis)
Calendar date | Period | Amplitude | DT-A/S-A (%) | |||
---|---|---|---|---|---|---|
All (3-years) data | Double tidal (DT) | 24.836 (24.833,24.838) | 0.10606 (0.0854,0.1268) | Global | ||
02/19/1990 | Compromise | 24.432 (24.427,24.436) | 0.05643 (0.0357,0.0772) | |||
to 03/11/1993 | Free-running | 24.260 (24.252,24.268) | 0.03253 (0.0118,0.0533) | |||
Societal (S) | 24.001 (24.000,24.001) | 0.25443 (0.2337,0.2751) | 0.41685 | |||
Tidal | 12.414 (12.410,12.418) | 0.01728 (−0.0034,0.038) | ||||
Semidian | 11.999 (11.997,12.001) | 0.03204 (0.0113,0.0527) | ||||
Local | 1: 02/19/1990 | Double tidal | 24.872 (24.830,24.914) | 0.02513 (−0.0070,0.0573) | ||
to 04/28/1991 | Compromise | 24.409 (24.384,24.435) | 0.04117 (0.0089,0.0734) | |||
Free-running | 24.222 (24.210,24.235) | 0.07921 (0.0468,0.1116) | ||||
Societal | 24.008 (24.004,24.011) | 0.31605 (0.2838,0.3483) | 0.07951 | |||
Tidal | 12.421 (12.411,12.431) | 0.02615 (−0.0059,0.0582) | ||||
Semidian | 12.007 (12.001,12.013) | 0.04105 (0.0090,0.0731) | ||||
2: 04/28/1991 | Double tidal | 24.898 (24.832,24.964) | 0.12744 (0.0707,0.1842) | |||
to 09/23/1991 | Compromise | 24.707 (24.671,24.743) | 0.25208 (0.1973,0.3068) | |||
Compromise | 24.488 (24.434,24.541) | 0.10274 (0.0552,0.1502) | ||||
Societal | 24.011 (23.992,24.030) | 0.22758 (0.1815,0.2736) | 0.55998 | |||
Tidal | Did not converge | – | ||||
Semidian | – | – | ||||
3: 09/23/1991 | Double tidal | 24.862 (24.852,24.872) | 0.34858 (0.3089,0.3882) | |||
to 03/08/1992 | Compromise | 24.523 (24.468,24.579) | 0.06108 (0.0212,0.1009) | |||
Free-running | Did not converge | – | ||||
Societal | 23.997 (23.984,24.011) | 0.22362 (0.1841,0.2631) | 1.55881 | |||
Tidal | 12.432 (12.416,12.448) | 0.04969 (0.0106,0.0888) | ||||
Semidian | Did not converge | – | ||||
4: 03/08/1992 | Double tidal | 24.942 (24.896,24.989) | 0.15133 (0.0960,0.2067) | |||
to 07/14/1992 | Compromise | 24.451 (24.394,24.508) | 0.11847 (0.0630,0.1739) | |||
Free-running | – | – | ||||
Societal | 23.987 (23.967,24.006) | 0.32099 (0.2660,0.3760) | 0.47145 | |||
Tidal | 12.432 (12.398,12.465) | 0.04960 (−0.0051,0.1042) | ||||
Semidian | 11.982 (11.951,12.012) | 0.05028 (−0.0044,0.1049) | ||||
5: 07/14/1992 | Double tidal | 24.789 (24.782,24.797) | 0.31836 (0.2798,0.3569) | |||
to 03/11/1993 | Compromise | 24.474 (24.438,24.510) | 0.06218 (0.0236,0.1008) | |||
Free-running | – | – | ||||
Societal | 24.009 (23.997,24.022) | 0.17892 (0.1405,0.2173) | 1.77934 | |||
Tidal | 12.352 (12.328,12.376) | 0.02358 (−0.0147,0.0619) | ||||
Semidian | 12.001 (11.988,12.013) | 0.04128 (0.0030,0.0796) |

Fig. 2.6
During the longest span of comfortable isolation from a 24-hour schedule of a healthy young woman in a special underground laboratory, but not before or thereafter, a period of 24.8 hours dominantly characterizes her blood circulation (among other peaklets also resolved) upon meta-analysis of original data as a whole (globally) and in serial sections (locally) (© Halberg Chronobiology Center)
2.4 Prokaryotes
By 1961, a circadian cycle shorter than 24 hours (of ~21 hours) was found in growth or colony advance of a prokaryote [20], Fig. 2.7a–d, and extended in scope [21], but was ignored for subsequent decades by those “naturalists” for whom periodograms and power spectra were (in the words of a personal communication by a sympathetic committee member) “too much mathematics.” Not realizing that ruling out a rhythm is difficult with the unaided eye (it requires time series analysis), a committee erroneously decreed, and its decision was accepted by many others for many years that circadians were limited to eukaryotes [22] until eventually a flood of papers on rhythms in prokaryotes set the record straight [23]. In 2010, we learned about a rhythm in archaeon, Fig. 2.8 [24].








Fig. 2.7
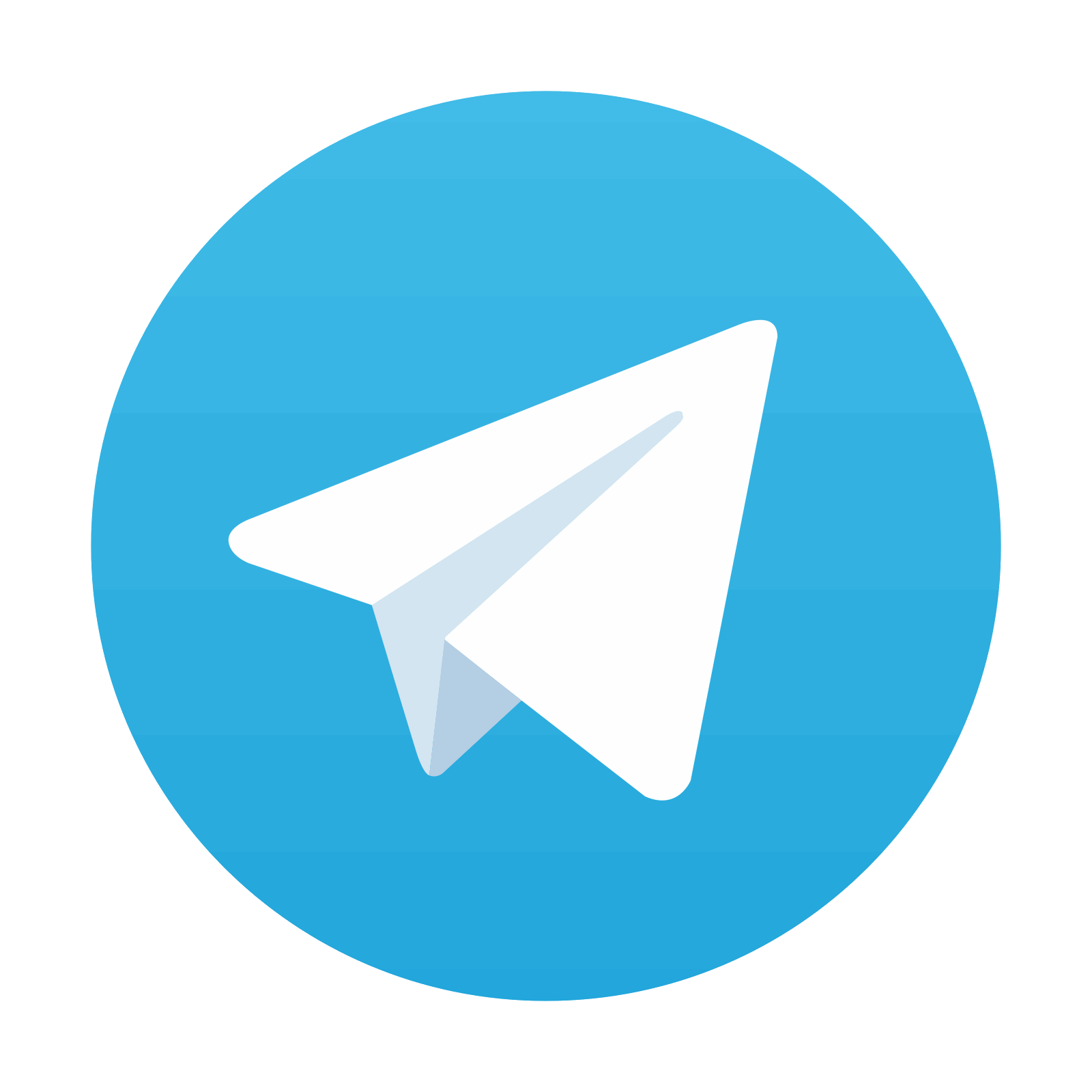
(a) Timeplot of the 1930 data of Rogers and Greenbank. Lore Rogers was a noted bacteriologist described by a Cosmos Club (Washington, DC) Vignette of December 1967 as “the bright star in the [US Department of Agriculture] scientific horizon before World War II.” In 1961, the data on growth rate (actually colony advance) of E. coli in a spiral glass tube, assessed at hourly intervals for slightly over 6 days, were taken off the graph published by Rogers and Greenbank in 1930. Some regularity in the time series is apparent from the spikes in colony growth occurring at intervals shorter than 24 hours, as seen by reference to the vertical dashed lines drawn at daily intervals, yet eyeballing can hardly serve for an estimate of a period and certainly forestalls any evaluation of uncertainties. Rogers and Greenbank wrote: “which is representative of a number of experiments, shows that there is a considerable degree of periodicity to the alternation of rapid and slow growth. In considering this graph, it should be kept in mind that the curve represents not extent of growth, but rate of advance of growth for definite time periods. The number of bacteria in the different periods could not be determined” (© Halberg Chronobiology Center). (b) By 1961, Rogers’ data on growth rate of E. coli (a) had been analyzed by a global periodogram and power spectra to find what the naked eye may miss, i.e., what was then interpreted as a free-running circadian rhythm, with a period of about 20.75 hours by Halberg and Conner [20]. An infradian modulation was subsequently reported. Herein, a result of linear-nonlinear rhythmometry summarizes period estimates for 2-day sections as well as for all data with their uncertainties. The spectral peak at a frequency slightly higher than 7 cycles per week (or 1 cycle per day) confirms the visual impression of the presence of a circadian variation with a period slightly shorter than 24 hours, presumably free-running. This about-21-hour component accounts for 14.4 % of the overall variance and is found to be statistically significant by cosinor (P < 0.001). Nonlinearly, the period is resolved as averaging 20.09 hours (95 % CI extending from 19.8 to 21.9 hours), as shown by the horizontal bar above the spectral peak. The peak’s amplitude averages 52.6 % of the MESOR (chronome-adjusted mean), with a 95 % CI extending from 19.4 % to 85.7 %, not overlapping zero (thereby rejecting the assumption of no rhythm). Additional nonlinear analyses applied to a moving 2-day interval (nonlinear chronomic serial section) indicate that this period is not fixed (c). The 95 % Cls of the shortest and longest periods are also shown as horizontal bars drawn at the corresponding amplitudes (© Halberg Chronobiology Center). (c) Periods and corresponding 95 % CIs characterizing the data shown in Fig. 21a are here estimated for intervals of 2 days progressively moved throughout the time series by 6 hours. An interval of 2 days was selected to have at least one replication to secure a more reliable estimate of the period while also allowing the investigation of any change in period length with time. Separate, nonoverlapping 1-day intervals analyzed similarly yield acceptable similar estimates. The period is seen to lengthen as a function of time. As a first approximation, the dash-dotted line is in keeping with a linear increase. Being only of the order of about 18 hours at the beginning of the series, it approaches 24 hours by the end of the first week of monitoring. With the qualification that consecutive estimates are not independent, the lengthening of the period has an ordering P < 0.001 (r = 0.900). The fit of a second-order polynomial (solid line) suggests that the period levels off. In this model, the quadratic term significantly reduces the residual variance (ordering P < 0.02) beyond the linear term. The results could be interpreted as an originally free-running circadian component that may be pulled by (if not become synchronized with) the 24-hour day, but there is a possible alternative, shown in D (© Halberg Chronobiology Center). (d) Amplitude estimates and 95 % CIs corresponding to the changing periods (in c) are shown herein. With the same qualification that consecutive amplitude estimates are not independent, further liner-nonlinear analyses of the amplitude sequence indicate the presence of an infradian component with a period of about 5 days (dashed curve). The “circadian” amplitude is quite large (of the order of 50 % of the MESOR), being larger in the middle of the series than at the start. The change in amplitude could be in keeping with a synchronization of the originally free-running component to a 24-hour cycle, but in that case, there should have been a more or less continuous increase until the end of the record. Results from the nonlinear analysis are in keeping with the alternative hypothesis of an infradian modulation of the circadian amplitude with a period of 4.96 days (95 % CI: 3.64–6.28 days). It should be noted that the data were collected under presumably constant conditions, except for the hourly illumination of the colony for taking the measurements. In his 90s, Lore Rogers (the senior author) first wrote in response to an inquiry that he could not recall the conditions of the study. Later he wrote to report that his technician and coauthor G.R. Greenbank (in Florida) had sent him a Christmas card. Rogers subsequently learned from Greenbank about the lack of any known external 24- or 20.75-hour cycle (the latter the average cycle length in a global analysis) (© Halberg Chronobiology Center)
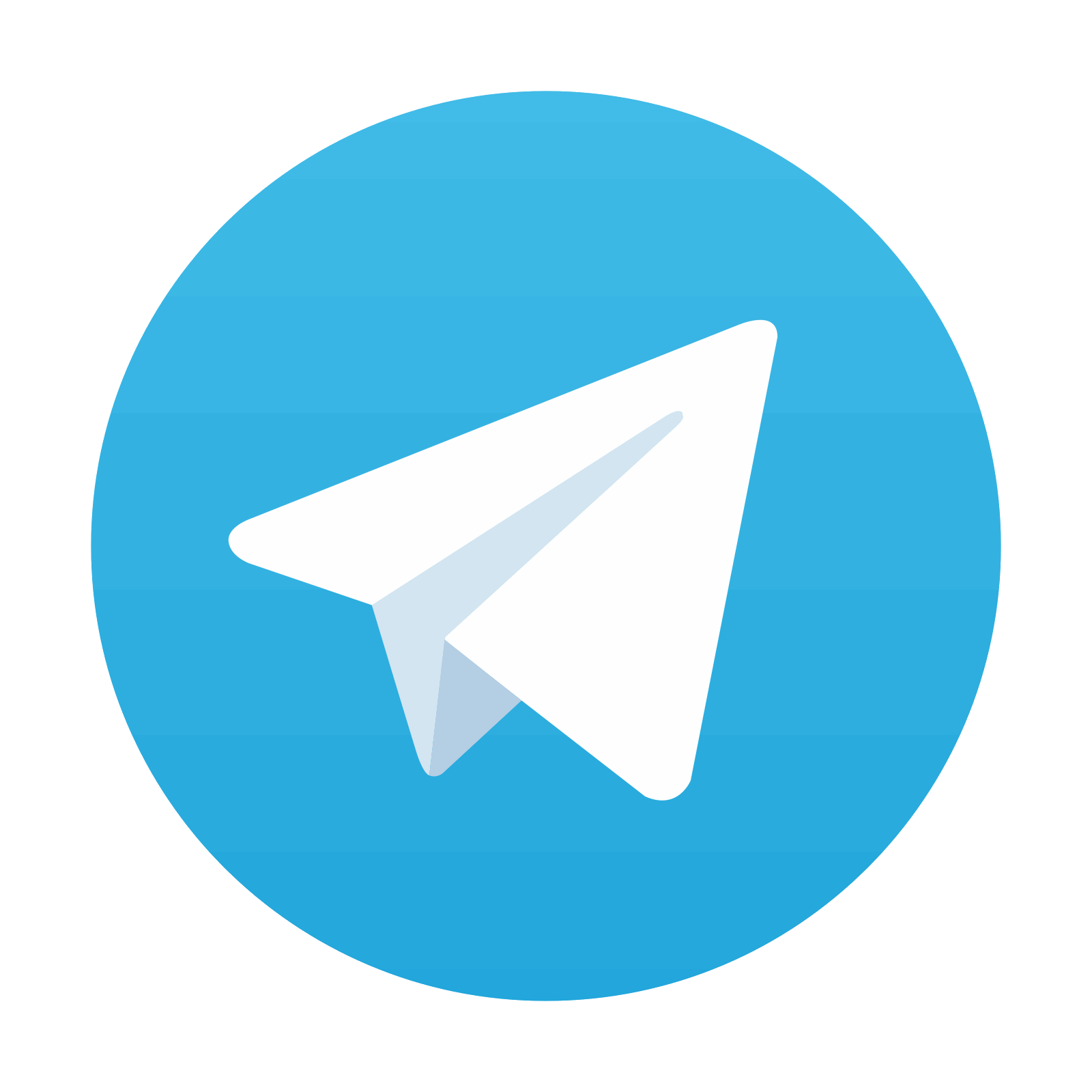
Stay updated, free articles. Join our Telegram channel

Full access? Get Clinical Tree
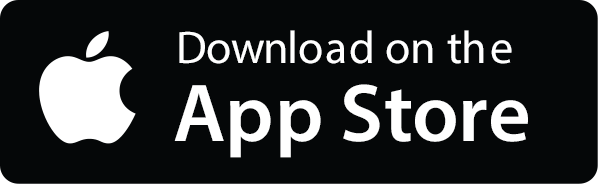
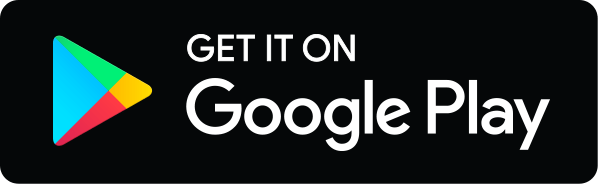
