CHAPTER 9 Bone perfusion derives from both the medullary and periosteal circulatory systems.1–4 The medullary system is mainly comprised of the nutrient arteries which penetrate the diaphyseal cortex through vascular for aminas and supply both the medulla and inner After fracture, an immediate decline of cortical blood flow of approximately 50% has been reported.12 The mechanism of injury and the forces at the fracture site dictate the “personality of the fracture” and the associated soft-tissue injuries that affect the local blood supply. Additional factors also affect local blood supply and include the specific nature of patient management and fracture care — from initial transport and resuscitation to subsequent surgical intervention and implant placement.13 The biologic responses during fracture healing depend on an intact blood supply,10 and fracture management should focus on optimizing the fracture site environment for osseous healing. Fig. 9.1. Microangiogram depicts the intracortical anastomosis between the medullary circulation and periosteal circulation (medulloperiostealanastomosis). Source: Rhinelander FW (1968). The normal microcirculation of diaphyseal cortex and its response to fracture. J Bone Joint Surg Am, 50(4), 784–800. Reprinted with permission. With non-displaced fractures, an intact medullary circulatory system continues to dominate the blood supply throughout the entire healing process.3 Displacement of fracture fragments interrupts the medullary system, the major contributor of blood flow to the cortical bone. The medulloperiosteal anastomoses are of significant importance, allowing the periosteal system to provide centripetal perfusion to the bone.2, 10 Fracture hematoma, which develops as the initial fracture healing response, interrupts communication of the medullary systems leaving the periosteal system as the sole connection of vascular supply between the fracture fragments through the external callus that initially forms.10 Surrounding muscles are the immediate origin of the expanded periosteal circulation observed during displaced fractures.2, 4, 10 Once endosteal osseous union and sufficient stabilization develops, the medullary capillaries can cross the fracture site and re-establish the normal predominant medullary circulation.2, 10 The mechanical conditions created during fracture care determine the biologic response of fracture healing and associated angiogenesis.13 When absolute fracture stability is achieved and the deformation, due to an applied given force (strain),14 at the fracture site is <5%, primary bone/direct healing occurs without fracture callus.15 This type of fracture healing is by osteonal bone remodeling, and consists of cutting cones that cross the fracture site.13, 16, 17 With direct contact of the fracture edges, osteoclasts are followed by osteoblasts directly laying down lamellar bone. If there is a fracture gap of 200–500 microns, woven bone is laid down first which is then remodeled to lamellar bone, but at the expense of increased time.13 Relative stability allows increased motion at the fracture site, but maintaining a mechanical strain between 5 and 15% creates secondary/indirect healing. This alternate form of fracture healing includes both intramembranous and endochondral bone formation. It is divided into fourstages: (1) inflammation, (2) soft callus formation, (3) hard callus formation, and (4) remodeling.13 Ruptured blood vessels resulted in the initial fracture hematoma; granulation tissue follows that becomes soft callus, eliminating free motion, and subsequently becoming hard callus, finally remodeling to the native shape. The ideal fracture fixation provides the optimal stability to stimulate an adequate bone healing process and maintains the reduction until osseous union is achieved. Current fracture treatments fall within a spectrum of stability where each end of the spectrum can lead to a delay in fracture healing.13 The composition of the metaphysis and epiphysis of both the proximal humerus and the proximal femur consists of mainly trabecular bone with a lesser component of cortical bone than the diaphysis and an excellent intraosseous anastomosis. When fractures occur through these bony segments, the arterial supply to the subchondral bone seems to be in great peril, and the development of osteonecrosis is of major concern. A careful surgical approach to achieve both an anatomic reduction and a stable fixation while maintaining the remaining blood supply is crucial to providing the ideal environment for fracture healing to occur. The arterial supply to these structures has received increased interest over the years. Much research has been focused on seeking a better understanding of the vascular injury during fracture and on new methods for optimizing surgical treatments of these injuries. We performed cadaveric injection studies that provided qualitative and quantitative assessment of the vascularity of the humeral head, femoral head, patella, and talus.18–23 This vascularity protocol utilizes gadolinium-enhanced magnetic resonance imaging (MRI) to quantify perfusion into a specific region of interest (ROI). Images were obtained using a 3.0 tesla GE MRI scanner (General Electric, Milwaukee, WI). High-resolution fat-suppressed three-dimensional gradient echo sequences were obtained both before and after administration of the contrast solution (gadoliniumdiethylenetriaminepenta-acetic acid, Magnevist; Bayer Health Care Pharmaceuticals Inc., Wayne, NJ). Fat-suppressed images were used to eliminate bone marrow signal and provide improved detail of the contrast. Both pre- and post-contrast images produced weighted average of signal intensities and histograms, which were normalized using a baseline of non-enhancing muscle. A single reading of signal enhancement was obtained for each area on both the control and test specimens. Following the MRI protocol, a polyurethane compound, using an added color dye, is injected into the arterial system and subsequently, careful gross dissection of the arterial system is performed in order to confirm injection into the correct arterial system and to correlate MRI findings and the previously assessed anatomical findings. The arterial supply to the humeral head arises from the axillary artery through the anterior and posterior humeral circumflex arteries.24, 25 The previous consensus in the literature was that the anterolateral branch of the anterior humeral circumflex artery provided the major blood supply to the humeral head.24–27 Our study results demonstrated that the posterior humeral circumflex artery provides a greater percentage (64% versus 36%) of blood supply to the humeral head, than did the anterior humeral circumflex artery as previously thought (Fig. 9.2a, b). This study highlighted the importance of avoiding dissection on the postero-medial aspect and to respect the posterior humeral circumflex artery during fracture manipulation and fixation. It provides a probable explanation for the relatively low rate of humeral head osteonecrosis following fixation of displaced three- and four-part proximal humerus fractures.28 Fig. 9.2a. Contrast-enhanced MRI images that illustrate the anterior humeral circumflex artery perfusion through the arcuate artery (left axial image) and the anterolateral branch (right coronal image). Source: Hettrich CM, Boraiah S, Dyke JP, Neviaser A, Helfet DL, Lorich DG (2010). Quantitative assessment of the vascularity of the proximal part of the humerus. J Bone Joint Surg Am, 92(4), 943–948. Reprinted with permission. Fig. 9.2b. Contrast-enhanced MRI images that illustrate the posterior humeral circumflex artery perfusion to the entire humeral head (left – axial; middle — sagittal; right — coronal). Source: Hettrich CM, Boraiah S, Dyke JP, Neviaser A, Helfet DL, Lorich DG (2010). Quantitative assessment of the vascularity of the proximal part of the humerus. J Bone Joint Surg Am, 92(4). 943–948. Reprinted with permission. Femoral head vascularity derives from three arterial systems: (1) retinacular, (2) foveolar, and (3) intraosseous.29–34 The retinacular system is the dominant arterial supply to the femoral head,29, 32, 34–38 and branches off from the medial (superior and inferior retinacular arteries) and lateral (anterior retinacular artery) femoral circumflex arteries. Once intracapsular, these terminal branches course within prolongations of the capsular wall, known as the retinacula of Weitbrecht,29, 31, 39–42 and enter the femoral head-neck junction distal to the articular rim (Fig. 9.3).30, 32, 37, 43 With growth of the femoral neck during childhood, the anterior retinacular artery recedes to penetrate the anterior metaphyseal area, while the superior and inferior retinacular arteries advance and provide the predominant arterial supply to the femoral head.32 Gross dissection of the terminal branches of the medial femoral circumflex artery (the superior and inferior retinacular arteries) demonstrated that these branches mainly penetrate the posterior-superior and posterior-inferior aspect of femoral head-neck junction (80% superiorly and 86% inferiorly),44 with the superior retinacular artery being the primary blood supply to the femoral head.1, 3, 6–10, 12, 15, 23, 24, 26–28 More recent studies have considered the inferior retinacular artery as a significant arterial supply to the femoral head, specifically when the superior retinacular system has been compromised. Our cadaveric studies, using quantitative contrast-enhanced MRI, demonstrated that 32–49% of femoral head perfusion is maintained when the inferior retinacular artery was preserved.18, 20 With fracture of the femoral neck, the inferior retinacular artery is protected by the inferior retinacula of Weitbrecht, which is separated and elevated from the femoral neck and can potentially avoid direct injury, contrary to the superior retinacular artery which courses in close proximity to the femoral neck and is at higher risk of injury in the event of a fracture (Fig. 9.4).44–46 A clinical series of displaced femoral neck fractures reported intraoperative confirmation of an intact inferior retinacula of Weitbrecht in 98% (108/110) of cases.47 Our series of displaced femoral neck fractures, using dynamic contrast-enhanced MRI, demonstrated maintenance of some perfusion to the femoral head despite fracture displacement, presumably through the intact inferior retinacular artery (Fig. 9.5).48 This study also demonstrated a decrease in venous outflow in the fractured side, probably secondary to the tamponade effect created by the contained intra-articular fracture hematoma. Fig. 9.3. The diagram depicts the combined results of 14 specimens. The outer circle represents the femoral capsular attachment; the inner circle represents the articular rim of the femoral head; and the millimeter scale represents the distance of the entry point of each terminal branch to the articular rim of the femoral head. MFCA = medial femoral circumflex artery; FHNJ = femoral head-neck junction. Entry point for the terminal branches: T = transverse MFCA; A = ascending MFCA; D = deep MFCA. Source: Lazaro LE, Klinger CE, Sculco PK, Helfet DL, Lorich DG (2015). Surgical Anatomy of the Medial Femoral Circumflex Artery Terminal Branches: Arterial Supply to the Femoral Head. (2015) Bone and Joint Journal, 97(9). Reprinted with permission. The arterial supply to the patella arises from the peripatellar anastomotic ring formed by the supreme genicular artery, superior/inferior medial genicular arteries, superior/inferior lateral genicular artery, and the anterior tibial recurrent artery (Fig. 9.6);21, 49 Quantitative and qualitative MRI data demonstrated that the dominant arterial system penetrates the patella at the distal pole mainly inferomedially (80%), supporting the recommendation of preserving the distal pole and the infrapatellar fatpad and the associated anastomotic network (Fig. 9.7).21 Transverse fractures of the patella jeopardize the blood supply to the proximal fragment with an average reduction of 36% (Fig. 9.8), probably contributing to the frequent reports of residual persistent pain following patellar fractures despite osseous union and articular congruency.21, 49 Fig. 9.4. The diagram demonstrates the intracapsular of the terminal braches arising from the medial femoral circumflex artery (MFCA). (Left) posterior aspect of one of the specimens demonstrates the posterior course of the MFCA, its relationship with the obturator externus muscle and conjoined tendon and the intracapsular course of the terminal braches. The inner square ilustrates the posterior inferior aspect of the femoral neck, illustrating the subsynovial course of the terminal branch of the ascending MFCA and intra-retinacular course of the inferior retinacular artery elevated off of the femoral neck. Source: Lazaro LE, Klinger CE, Sculco PK, Helfet DL, Lorich DG (2015). Surgical Anatomy of the Medial Femoral Circumflex Artery Terminal Branches: Arterial Supply to the Femoral Head. (2015) Bone and Joint Journal, 97(9). Reprinted with permission. (Right) superior aspect of a specimen that illustrates the superior retinaculum of Weitbrecht before and after (inner square) being incised, exposing the superior retinacular arteries (terminal branches of the deep MFCA) coursing closely against the femoral neck. Source: Lazaro LE, Klinger CE, Sculco PK, Helfet DL, Lorich DG (2015). Surgical Anatomy of the Medial Femoral Circumflex Artery Terminal Branches: Arterial Supply to the Femoral Head. (2015) Bone and Joint Journal, 97(9). Reprinted with permission. Fig. 9.5. Average time intensity curves of the dynamic contrast enhanced MRI (n = 27) demonstrating the perfusion to the entire femoral head on both the injured and contralateral (uninjured) hips. Source: Dyke JP, Lazaro LE, Hettrich CM, Hentel KD, Helfet DL, Lorich DG (2013). Regional analysis of femoral head perfusion following displaced fractures of the femoral neck. J Magn Reson Imaging. Reprinted with permission. Fig. 9.6. The drawing depicts the extraosseous and intraosseous vasculature of the patella. The terminal branches supplying the patella penetrate the patella at the anterior cortex from the dorsal vessel system (mid-patellar vessels, shown in orange color), and the radial vessel system supplies the terminal branches entering at the border of the patella (peripatellar and polar vessels, shown in brown color). Source: Lazaro LE, Wellman DS, Klinger CE, Dyke JP, Pardee NC, Sculco PK, Helfet DL, Lorich DG (2013). Quantitative and qualitative assessment of bone perfusion and arterial contributions in a patellar fracture model using gadolinium-enhanced magnetic resonance imaging: Acadaveric study. J Bone Joint Surg Am, 95(19), e1401–e1407. Reprinted with permission. Fig. 9.7. Gross dissection of the infrapatellar fat pad area illustrates the transverse infrapatellar branch giving off the polar vessels and connecting the medial and lateral vessels of the peripatellar anastomotic ring. (Left) the patellar tendon was detached and elevated proximally to illustrate the infrapatellar area posterior to the patellar tendon. (Middle) A lateral arthrotomy was performed and the patella was inverted to illustrate the polar vessels penetrating the patella on the inferiomedial aspect of the distal pole, posterior to the patellar tendon and anterior to the articular cartilage. (Right) the coronal MRI image revealed the dominant vessel entering at the inferior pole at the inferomedial aspect. Source: Lazaro LE, Wellman DS, Klinger CE, Dyke JP, Pardee NC, Sculco PK, Helfet DL, Lorich DG (2013). Quantitative and qualitative assessment of bone perfusion and arterial contributions in a patellar fracture model using gadolinium-enhanced magnetic resonance imaging: Acadaveric study. J Bone Joint Surg Am, 95(19), e1401–e1407. Reprinted with permission.
FRACTURES AND BONE REPAIR
9.1 Mature Long Bones Vascularity and Fractures
of the cortex in a centrifugal fashion.1–6 At the metaphysis and epiphysis, additional arterial systems penetrate the bone and join the medullary system supplying their respective segments of medulla and cortex. The periosteal system, mainly sustained by the surrounding muscles, provides a centripetal supply through small arterioles that pierce and supply the outer
of the cortex, especially when the medullary blood supply has been interrupted.7–9 These two microcirculations anastomose (medulloperiosteal anastomosis) within the cortex and allow for blood to flow through the cortex (Fig. 9.1).2, 10 Under normal conditions, the medullary system dominates the arterial supply.1, 2, 10, 11
9.2 Vascularity of Key Periarticular Bones
9.2.1 Humeral head vascularity
9.2.2 Femoral head vascularity
9.2.3 Patellar vascularity
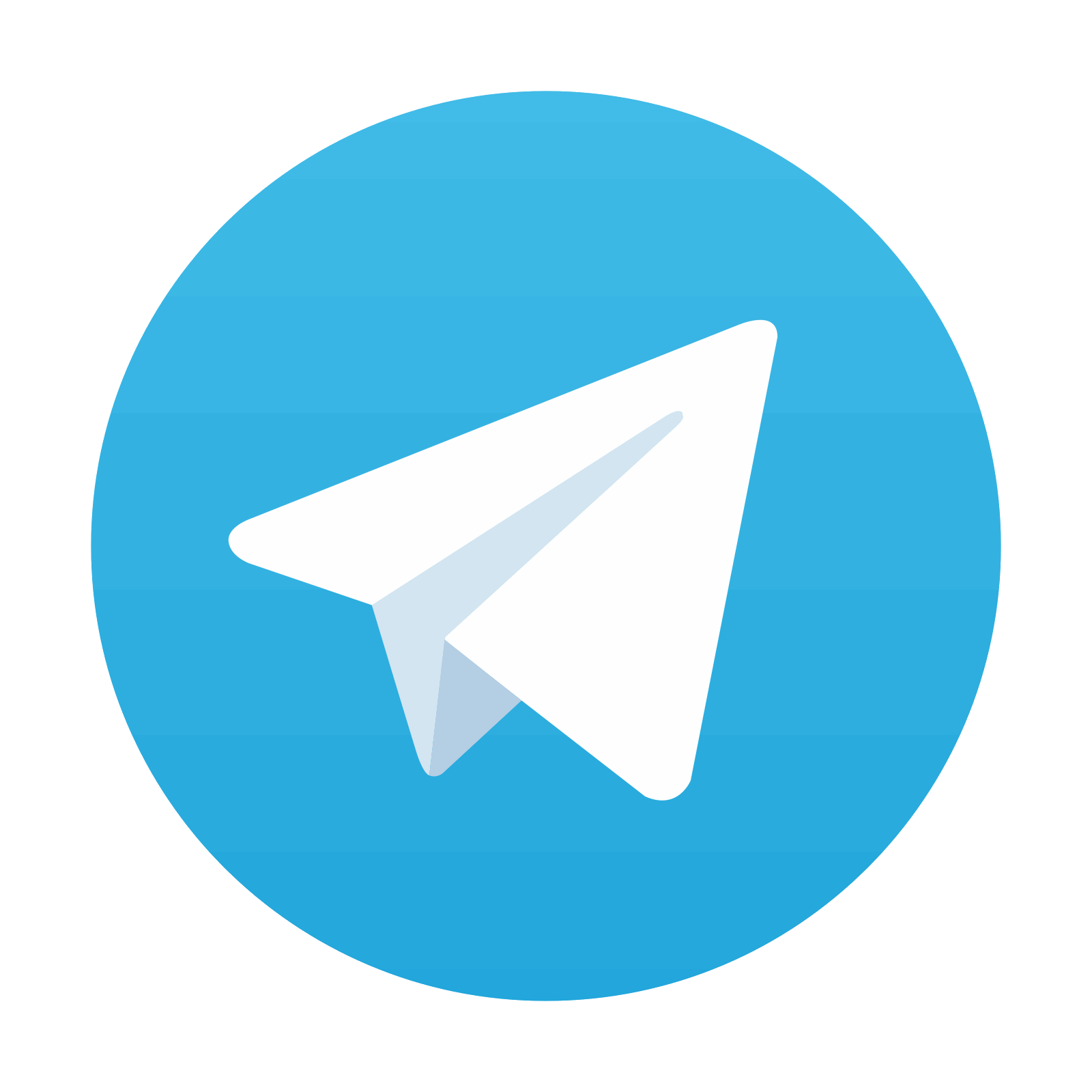
Stay updated, free articles. Join our Telegram channel

Full access? Get Clinical Tree
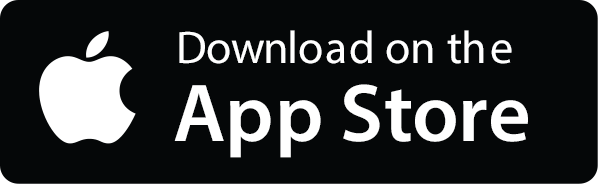
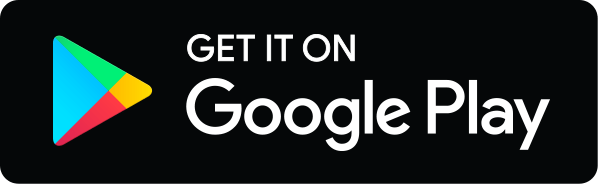