(a, b) The evolution of ECMO technology over time from large, complex systems to compact modern devices
Irrespective of the changes over the last several decades, ECMO consists of a small number of essential components: a pump, an oxygenator, a heat exchanger, cannula(s), tubing (circuit), and various monitoring components.
The Pump
Both roller and centrifugal pumps are still in use, although many centers have moved to centrifugal pumps because of their compact size, absence of a need for a bladder, and lower maintenance. Roller pumps move blood thru compression of the blood against the pump head housing, moving it ahead at fairly high pressures (as high as 350 mmHg) depending on tubing size. Advantages of roller pumps include the fact that forward flow can be calibrated and is not determined by downstream pressure and the delivery of blood flow is physiologic. Centrifugal pumps, in contrast, generate flow by a spinning rotor that creates suction and, consequently, a pressure gradient across the pump head. A downside is that flow is affected by downstream pressure (e.g., the patient’s vascular resistance); thus, an external flow probe is required to measure output. The success of centrifugal pumps has been facilitated by lower resistance of the newer hollow-fiber oxygenators. Higher rates of hemolysis and kidney injury have been reported with centrifugal pumps [31], but newer designs appear to have addressed this problem [32, 33]. The advantages and disadvantages of different types of pumps are beyond the scope of this brief review, and the reader is referred to several references as well as the ELSO Red Book [13] for further details.
The Oxygenator
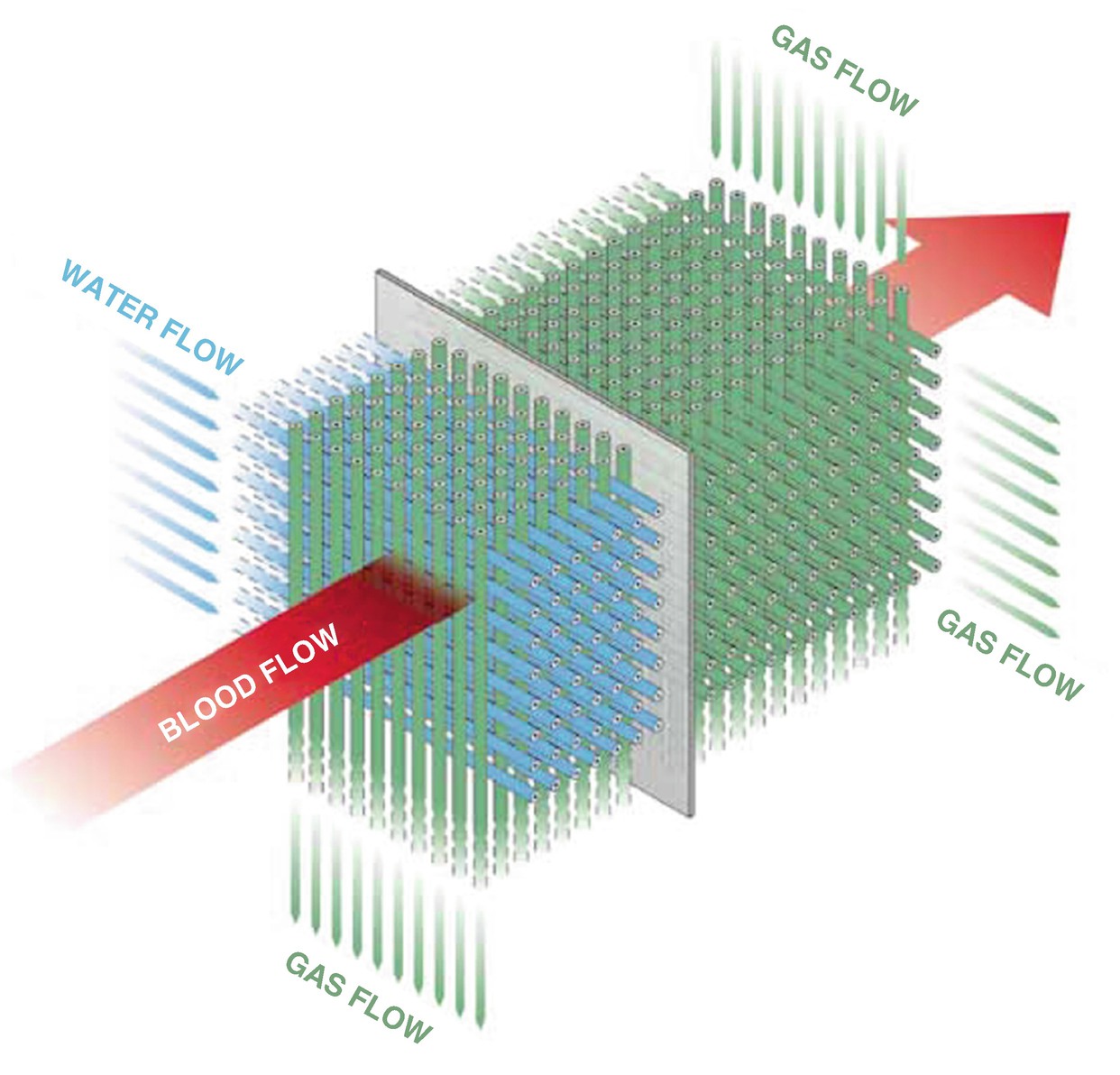
A schematic of the Maquet Quadrox oxygenator
Cannulas
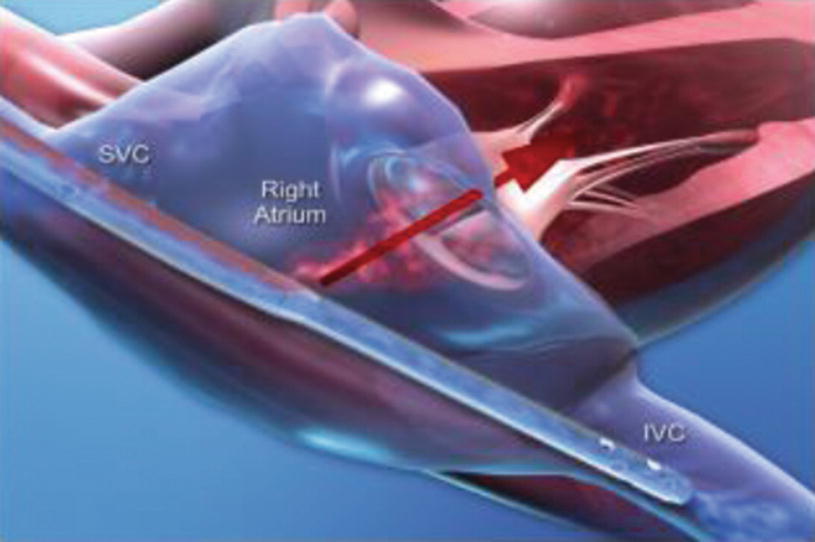
Avalon Bicaval VV ECMO Catheter
The Circuit
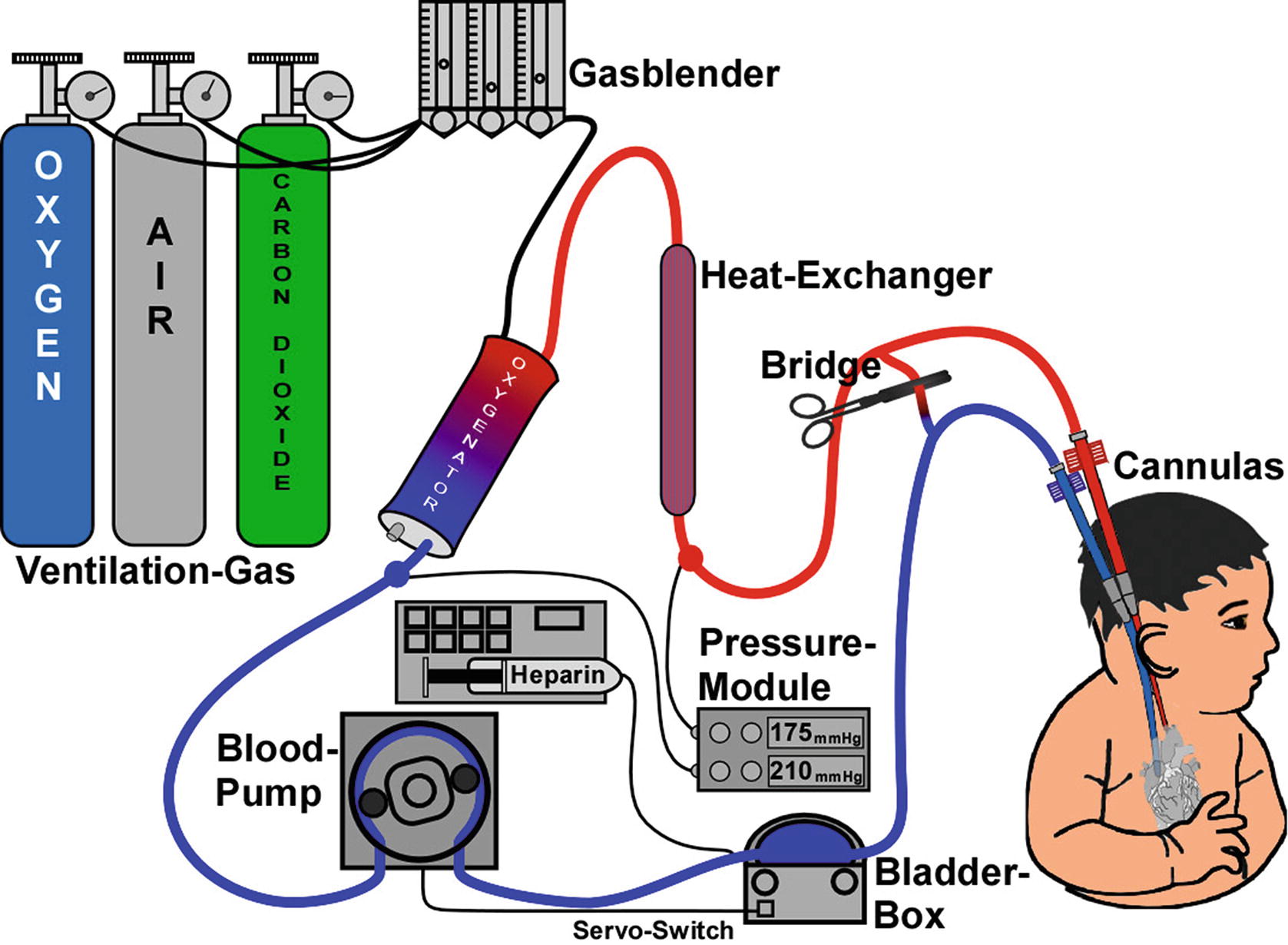
Standard ECMO circuit. (From https://commons.wikimedia.org/wiki/File:Ecmo_schema-1-.jpg [Jürgen Schaub])
Monitoring
Standard monitoring includes pump inlet pressure monitor, pre- and postoxygenator pressures, flow monitors, air bubble detector, and oxygen saturation/blood gas. Many centers also employ hemoglobin monitors. The newest ECMO devices have these monitors integrated into the circuit with display on a single LED screen.
Summary on the ECMO Machine
Previous ECMO devices were complicated and often relied on components from different manufacturers that were not necessarily designed for ECMO. The newer ECMO devices have become integrated and more compact, consequently requiring less “babysitting.” Monitors are integrated into the circuit such that pressures, flows, and other variables can be visualized continuously with integrated alarms for ease in monitoring and identification of problems. These advances have undoubtedly improved the safety of ECMO. Recent ELSO data suggest that outcomes with ECMO are improving, and this may in part reflect these technologic advances as well as improvements in other aspects of care [39].
ECMO for PARDS
Overview
- 1.
Is the trajectory of the lung injury such that the risks of ECMO are outweighed by the risks of continuing mechanical ventilatory support at potentially injurious settings?
- 2.
Is the lung injury recoverable or, if not, is lung transplantation feasible?
- 3.
Is there evidence of other organ failures and, if so, are the additional organ failures potentially recoverable?
- 4.
What are the family’s beliefs and considerations regarding the goals of care?
Possible indications and relative contraindications for ECMO
Indications | Relative contraindications |
---|---|
Severe refractory hypoxemia (e.g., P:F ratio <60–80, oxygen index >40) | Duration of mechanical ventilation >14–21 days before ECMO |
Toxic ventilator settings (e.g., Pplat >32, MAP >25–30) | Recent intracranial surgery or hemorrhage |
Refractory hypercarbia | Chronic illness with poor long-term prognosis |
Refractory air leaks |
Patient Populations
PARDS can result from a variety of insults and individual patient factors may greatly influence outcomes: primary diagnosis, comorbidities, age, and pre-ECMO physiologic variables. Primary diagnosis is among the most important ECMO prognostic factors [39]. According to the 2017 ELSO data, “other” is the largest category for pediatric respiratory cases, with “viral pneumonia” and “acute respiratory failure” more frequent than “ARDS.” A major weakness in the ELSO data is the precise definitions of each of these categories are unclear and it is probable that many patients in each of the ELSO respiratory diagnostic categories would also meet the definition of PARDS by the time ECMO is being considered. Survival statistics differ somewhat by diagnostic category. Survival is reported as 67% for aspiration pneumonia, 65% for viral pneumonia, 59% for bacterial pneumonia, 56% for ARDS, 55% for acute respiratory failure, and 52% for the “other” category [13]. As reported by Zabrocki and colleagues evaluating the ELSO 1993–2007 data, survival with ECMO ranged from a high of 83% for asthma and a low of 23% for fungal pneumonia [39].
Comorbidities also greatly influence outcomes. In Zabrocki’s review [39] of 3213 children from the 1993–2007 ELSO dataset, any comorbidity was associated with a decrease in survival from 57% to 33%, with “immune compromise” the most significant. Overall survival from respiratory failure for children with immune compromise placed on ECMO is 33–35% [39], but survival after hematopoietic stem cell transplant (HSCT) is dismal with one report of no survivors in 20 patients [40] and another reporting survival to hospital discharge in only 3/29 children [41]. Genetic abnormalities are also likely to impact survival, although it would depend on the type of genetic abnormality. In the specific case of Down syndrome, survival on ECMO is comparable to survival of children without trisomy 21 [42]. Renal failure increased the likelihood of mortality by a factor of 1.77 [43], although CRRT did not change survival if it was used for fluid overload (hemofiltration) rather than support of renal failure. At least two studies have demonstrated that congenital heart disease significantly decreased survival [44, 45], although survival with cardiomyopathy/myocarditis according to one meta-analysis was 63% [46]. Liver failure was also associated with very poor survival in the ELSO data (17%).
Age also appears to be a factor, although whether this reflects different underlying diagnoses and comorbidities or is actually a function of age is unclear. In Zabrocki’s data [39], children aged 10–18 had a significantly lower survival (50%) compared to infants (57%), toddlers (61%), and children (55%).
Duration of ventilatory support prior to ECMO remains a prognostic factor. Earlier data from Moler et al. [47], suggested that pre-ECMO ventilation for >7 days was the cut-off for successful ECMO, but more recent data support that patients ventilated up to 2 weeks had similar outcomes [48]. The number of patients ventilated for longer than 2 weeks is small, but it is notable that even after ventilation for >2 weeks survival was still 38% [39]. These data reflect duration of pre-ECMO ventilation as a single factor and it is likely primary diagnosis, comorbidities, or other factors affect these statistics. The data cannot tell us the reason for delayed ECMO, and it is probable that many of these children were ventilated for other reasons prior to their development of lung injury requiring ECMO support. Nonetheless, the upper limit of time on prior ventilation continues to be revised upward.
While our ability to predict outcome for the individual child with PARDS is limited, recent data from the prospective observational PARDIE study suggests oxygenation in the first 24 hours after initiation of mechanical ventilation is predictive of mortality [3]. In that study an OI > 16 (or OSI > 12.3) at 6 hours after initiation of mechanical ventilation predicted 34% mortality. Measures of ventilatory efficiency, including Vd/Vt [49] and ventilation index [50], may further add to predicting mortality risk and assist in the determination of if and when to initiate ECMO. While these factors clearly correlate with outcomes with conventional support, whether and to what degree these outcomes can be altered by early use of ECMO is unknown.
Ultimately, the decision to employ ECMO is a clinical one. Sometimes the decision is relatively straightforward because the child is failing despite high ventilator pressures or volumes that are clearly not sustainable. Other times it simply becomes a judgment as to which carries the lesser risk – and that judgment is going to hinge on the experience of the clinician and the ECMO center as much as objective measurements of failing lung function. The decision-making process promises to evolve in the coming years as the technology improves and ECMO becomes simpler, presumably safer, and more routine.
Conduct of ECMO for PARDS: VV ECMO
Both VV and VA ECMO can be used for respiratory support in PARDS. VV ECMO is increasingly favored for reasons previously discussed – fewer neurological complications, avoidance of sacrificing a major artery or jeopardizing an extremity, and improved survival [51, 52]. VV ECMO does not support the heart, but diminishing cardiac function generally improves with restoration of adequate oxygenation and normalization of acid/base balance. Thus, poor myocardial contractility or use of modest-dose vasoactives is not an absolute contraindication to using the veno-venous approach. Because of the recirculation and mixing inherent when venous blood from the oxygenator is returned to the venous system, however, management of VV ECMO differs somewhat from VA ECMO.
- 1.
Relative positions of the outflow and inflow venous cannulas: When two cannulas are used, taking blood from the IVC and returning it into the SVC results in demonstrable better SaO2 than vice versa. With the double-lumen catheters, the position of the outflow lumen toward the tricuspid valve in the right atrium is critical (Fig. 16.3).
- 2.
Size of the cannulas: Larger sizes enable higher flow rates with less negative venous drainage pressure and, consequently, less mixing.
- 3.
Intrathoracic pressure: High intrathoracic pressure (such as with pneumothorax, tamponade, or status asthmaticus) impedes venous return and increases recirculation, though it may also reduce pulmonary blood flow and drive more desaturated blood into the ECMO circuit.
- 4.
Blood flow: As ECMO blood flow increases, the venous limb of the circuit pulls in more deoxygenated blood (i.e., effective blood flow, which increases systemic oxygenation) and also more oxygenated blood (i.e., recirculation of blood that has already passed through the ECMO circuit). At some point, increasing ECMO blood flow further increases recirculation but does not improve systemic oxygenation.
Ultimately, the adequacy of the SaO2 can be judged by clinical effects (HR, BP, perfusion, urine output) or oxygen delivery (SaO2–SvO2), but arterial lactate may be the simplest and best single indicator of the adequacy of a given oxygen saturation.
- 1.
Increasing pump flow: Often flows higher than normally used in VA ECMO are needed, although recirculation usually increases proportionately and often reaches diminishing returns.
- 2.
Changing the catheter positions or adding an additional drainage catheter.
- 3.
Augmenting hemoglobin with transfusion to increase SvO2.
- 4.
Improving cardiac output with volume or inotropes (which may not necessarily improve SaO2 but may improve oxygen delivery) to increase SvO2.
- 5.
Using the lungs: Increase ventilator settings.
- 6.
Change to VA ECMO if the above are insufficient to achieve adequate oxygenation.
Weaning on VV ECMO as the lung improves is relatively straightforward. Both sweep gas and/or ECMO flows can be decreased and ventilator settings increased proportionately. Avoidance of high volumes or pressures on the ventilator remains important consideration and weaning ECMO should not be at the risk of inducing lung injury from the ventilator. Unlike with VA ECMO, pump flow rates can be maintained and sweep gas simply turned down and eventually to off as the ventilator (or spontaneous breathing) resumes the work of oxygenation and ventilation.
VA ECMO for PARDS
Despite the benefits of VV ECMO in PARDS, the anticipated or real need for cardiac support may mandate use of VA rather than VV ECMO. Assuming a functioning oxygenator, management of oxygenation in VA ECMO is straightforward and directly proportional to ECMO flow. Inadequate SaO2 is generally remedied by turning up the pump flow. It may, however, be necessary to decrease the sweep gas flow in order to maintain the desired PaCO2 because carbon dioxide removal is very efficient with current oxygenators. Inadequate ventilation generally responds to turning up the sweep gas flow, although at very low flow rates, it may be necessary to increase pump flow as well. Fortunately, with today’s ECMO setup, oxygen provision and carbon dioxide removal can be uncoupled.
Weaning on VA ECMO primarily involves turning down the pump flow. Lower flow rates increase the risk of clot/thrombosis, so anticoagulation may require closer attention. With use of an arterial–venous bridge (described previously), it may be possible to briefly “trial off” without disconnecting or removing cannulas as a means of assessing adequacy of cardiopulmonary function and the likelihood of successful separation from ECMO. While this strategy helps to minimize the risk of clot in the ECMO circuit, the distal portion of the circuit between patient and bridge and the cannulas remain at risk for clot.
Management of the Lungs
Management of mechanical ventilation while on ECMO remains controversial. The ELSO guideline states to “avoid fluid overload and damaging ventilator settings” [13]. For pediatric patients, the recommendations are use of higher PEEP (10–15 cmsH2O), low rates, low peak or plateau pressures (PIP < 28–30 cmH2O), and FiO2 < 50%. A meta-analysis of 9 adult studies suggested higher driving pressure (PIP-PEEP) was independently correlated with mortality [54]. The CAESAR study [22] notably maintained peak pressures <25 cmH2O. In a study in adults, Schmidt, et al., [55] reported higher PEEP levels improved survival. Some authors have advocated for extubation while on ECMO [56], particularly for patients awaiting lung transplant. Management will also be undoubtedly influenced by the type of ECMO support. In VV ECMO, utilization of native gas exchange is often necessary; thus, manipulation and utilization of the ventilator are more common than during VA ECMO during which lung “rest settings” are commonly deployed. This authors’ practice has been to maintain lung recruitment and utilization of the lungs during VA ECMO but not to the degree that would be injurious. Minimizing the deleterious effects of further lung injury caused by collapse is likely beneficial. There have been no randomized studies in children and ELSO data as well as an international survey demonstrates no agreement about the best approach to manage the lungs.
Anticoagulation
Cardiopulmonary bypass and, subsequently, ECMO only became possible with the discovery of heparin. Heparin is inexpensive, has relatively few side effects, has a short (30–60 minutes) half-life, and is easily reversed [57]. Unfractionated heparin remains the mainstay of anticoagulation for both adult and pediatric ECMO, but heparin is far from a perfect anticoagulant. Heparin activity relies on antithrombin III (ATIII), the level of which is variable in neonates (due to ineffective synthesis) and which can fall over time on ECMO due both to ineffective production and dilution related to underreplacement with ATIII directly or with FFP, which contains ATIII. Additionally, heparin can induce development of antibodies that result in bleeding and thrombosis (heparin-induced thrombocytopenia, or HIT).
There is little consensus on how to monitor anticoagulation [58–61]. The activated clotting time (ACT) is time-honored, rapid, and requires little blood, but some studies have suggested that measuring the direct effect of heparin on thrombin by measuring activated factor ten (Xa) levels is more predictive of the degree of anticoagulation [62, 63]. Unfortunately, some hospitals do not measure Xa routinely, its measurement requires a larger blood sample, and the turn-around time for the assay may be prolonged such that “real-time” interventions are limited when using Xa assay as the primary source for decision-making. Utilization of Xa assay only evaluates the coagulation cascade devoid of cellular elements. Thromboelastography (TEG) and rotational thromboelastography (ROTEM) offer a more complete assessment of anticoagulation as well as fibrinolysis and can be done at the bedside [64]. At present, however, few studies have looked at the predictive value of TEG or ROTEM in ECMO. While strict adherence to an anticoagulation protocol has been shown to decrease the incidence of complications [65], what constitutes the best protocol for both anticoagulant administration and monitoring remains to be determined. In clinical practice, most ECMO centers utilize some combination of point of care testing (ACT) with Xa measurement and TEG/ROTEM in circumstances when ACT and Xa alone are equivocal in the setting of uncertainty.
Direct thrombin inhibitors such as bivalirudin show promise for use in ECMO. Anticoagulation is accomplished independent of ATIII and, as such, reduces the need for exogenous ATIII administration and may alleviate the variability seen with unfractionated heparin. Another advantage of bivalirudin is that it inhibits both circulating as well as clot-bound thrombin, whereas unfractionated heparin is not effective on existing clot. This may confer significant benefit, as ECMO circuit clot remains a significant complication during ECMO support. Monitoring of bivalirudin is done through measurement of aPTT rather than Xa. Utilization of ACT and TEG is still effective with bivalirudin as these assays reflect “whole blood” clotting. Data supporting bivalirudin use and superiority in pediatric ECMO are lacking, however, so its use remains center and clinician dependent. In the future, heparin-coated circuits and use of direct thrombin inhibitors may simplify anticoagulation on ECMO, but at present, anticoagulation on ECMO remains more of an art than a science.
ECMO Complications
Several problems are inherent with the use of ECMO. Bleeding and its counterpart, thrombosis, largely related to the exposure of blood to the nonbiologic surface of the ECMO circuit, are common and are associated with significant morbidity and mortality. ECMO patients are also at high risk for infection due to the large amount of foreign material and the need for frequent interruption in the circuit to draw labs and give blood products and medications. Mechanical problems from circuit leakage/rupture or cannula displacement have fortunately become less common but necessitate constant vigilance. Additionally, other organs can be adversely affected consequent to hemolysis, nonpulsatile flow (VA ECMO), or air or other embolic phenomenon from the circuit. A brief description of the more common problems follows.
Bleeding and Thrombosis
The most common problem in ECMO is bleeding, and this is followed closely by its antithesis, thrombosis. The BATE Study (Bleeding and Thrombosis in Children) was a prospective observational cohort study in 514 children that reported an incidence of all bleeding events just over 70%, with intraventricular hemorrhage (IVH) in 17% [59]. Thrombosis occurred in 37.5% of subjects, while 31% had thrombosis involving the ECMO circuit. Anticoagulation was variably managed in the study, with nearly all using heparin infusions. Methods of monitoring of anticoagulation were highly variable between practices, some monitoring only ACT, others following PTT, and still others Xa. The study documented large variations in use of plasma and ATIII, frequency of monitoring, and rates of thrombosis and bleeding across the 8 sites. Unfortunately, the study offered no conclusions regarding the optimal anticoagulation or monitoring regimen. This appears to be the current state of the science.
Infection
The presence of multiple indwelling catheters and need for frequent interruption of the ECMO circuit for blood draws, medication administration, and delivery of blood products places the child on ECMO at high risk for nosocomial infection. Rates for pediatric infection reported to the ELSO database are 20.8/1000 ECMO days [66], although a Canadian study suggested higher rates [67]. Coagulase-negative Staphylococcus, Pseudomonas, and Candida were the most common. Surprisingly, in at least one study, infection was not independently associated with mortality [68]. A recent study from the CPCCRN reported a 16.6% rate of infection with an average onset at 5.2 days [69]. The most common sites of infection included bloodstream (4.4%), urine (4.2%), respiratory (11%), and others (4.2%). The most common organisms in that study were Candida albicans, Staphylococcus aureus, Enterobacter cloacae, Pseudomonas aeruginosa, Escherichia coli, and Staphylococcus epidermidis. Recommendation for routine surveillance of both blood and urine for presence of infection is not strongly supported but is often performed given the consequences of infection and the inability to detect fever due to temperature regulation accomplished by the ECMO circuit.
Organ Dysfunction
It is often difficult to know if organ dysfunction is consequent to ECMO or preceded it. Some organ dysfunction, such as poor cardiac contractility, may actually improve with initiation of ECMO because the cause – hypoxia and/or acidosis – has been remedied. Renal dysfunction, however, may sometimes be precipitated by ECMO and may relate to nonpulsatile flow or hemolysis and high levels of free hemoglobin [70]. The most ominous complication is intracranial bleeding or stroke. This is more common with VA than VV ECMO and probably occurs more frequently than is realized [7]. A study by Lockie et al., showed that 16.4% of patients on ECMO had CT scan evidence of bleeding and/or stroke when imaging was performed [7]. Current recommendations suggest obtaining a CT or, preferably, MRI scan prior to discharge. Seizures are also a common complication of ECMO and may be the first indication of intracranial hemorrhage [7].
Cannula Problems
ECMO cannulae are associated with the same complications that occur with comparably sized central cannula placed for other indications. The newer double-lumen catheters used in VV ECMO, however, are more problematic. Because of the bicaval design, precise placement is critical. Unfortunately, the catheters are relatively stiff and placement incurs the risk of the catheter entering the right ventricle rather than the IVC, which can result in perforation and subsequent tamponade [27]. Placement utilizing fluoroscopy and/or echocardiology is strongly recommended. Problems with distal extremity ischemia are not uncommon with femoral artery catheters and often require bypass grafts or catheters to prevent loss of viability. Bleeding at cannula site is a common occurrence and often difficult to both quantify and remediate.
Neurological Complications
Central nervous system infarct and bleeding are the two most feared complications of ECMO. In a review of 2617 children with respiratory failure supported with ECMO, the overall incidence of CNS hemorrhage or infarct was 9.6%, with a significantly greater incidence in VA (11.8%) compared to VV (6%) ECMO [7]. A very interesting study in adults from the UK, however, demonstrated that VA ECMO was not an independent risk factor for intracranial hemorrhage (ICH) [71]. Furthermore, in that study, there was no significant difference in 6-month survival between patients with and without ICH. The ELSO data quote a 79% mortality with ICH on ECMO compared to only 38% without ICH [7]. This has prompted the suggestions that all patients should if possible have CNS imaging prior to ECMO and prior to hospital discharge.
Long-Term Outcomes on ECMO for PARDS
To quote Zabrocki et al., “Although the survival of pediatric patients with acute respiratory failure treated with extracorporeal membrane oxygenation has not changed, this treatment is currently offered to increasingly medically complex patients” [39]. The increasing complexity of patients supported with ECMO is but one factor that makes broad statements about long-term outcomes in ECMO difficult. The different indications for which ECMO is utilized in children – cardiac failure, respiratory failure, or eCPR – are additional factors. In general, multiple reports suggest that VV ECMO is associated with better long-term outcomes than VA [37–39, 72], but this may reflect the underlying reason for ECMO support rather than the ECMO method. Follow-up studies on infants and children surviving ECMO paint a mixed picture, although perhaps better than would be expected. In a review of over 50 studies of follow-up of children receiving ECMO for a range of diseases that included congenital diaphragmatic hernia to respiratory and post-op cardiac support, more than half of survivors did well [73]. Cognitive deficits ranged from 10% to 50%, behavior problems from 16% to 46%, and severe motor impairment was seen in 12%. Overall quality of life evaluated at school-age or adolescents ranged from 31% to 53% having a score more than 1 standard deviation below norms in their age groups. Clearly, the glass is either half-full or half-empty. Unfortunately, there exists almost only limited data in follow-up of children with PARDS. The need for follow-up studies in PARDS was identified as a major deficiency in the PALICC conference [74].
One argument for earlier or “more aggressive” use of ECMO in PARDS is the potential of better pulmonary outcomes by avoidance of injurious levels of positive pressure ventilation. While an attractive argument, there are almost no data on long-term pulmonary function in children with PARDS [74, 75]. Data on children hospitalized for RSV, some of whom would qualify as PARDS, suggest long-term effects on airway reactivity, but it is not clear that this is a consequence of the acute lung injury or a prior predisposition to wheeze [76, 77]. What little data there are in PARDS suggest that pulmonary outcomes are generally at least okay, with subtle abnormalities identified on pulmonary function testing but few limitations on exercise, etc. PICU patients do appear to be at significant risk for PTSD and generally lower quality of life, but the relationship of these findings to lung injury and its support in the PICU is difficult to distinguish from other causes [78]. This has to be considered in the context of the very real risk of CNS injury from bleeding, clot, or embolization during ECMO. Fortunately, these complications are considerably fewer with VV than VA ECMO and most children with PARDS can be adequately supported with VV ECMO. In a study of the California Patient Discharge Database [79], children who survive ECMO have a high rate of hospital readmission (62% in one study), neurological problems/developmental delay (7–9%), and late deaths (5%).
Conclusion
Unfortunately, similar to the determination that ventilator-induced lung injury is likely to outpace lung repair, determination that ECMO offers a better chance for long-term survival is a matter of clinical judgment. As evidenced by the high survival in status asthmaticus, children with clearly reversible lung disease without other comorbidities can do very well with ECMO. The decision to use ECMO becomes increasingly difficult in the chronically ill child (e.g., stem cell transplant) or child with other organ failures where often it seems the choice is between the slim chance offered with ECMO versus the near-certainty of death without ECMO. Against this backdrop is the improving technology and experience making ECMO more readily available, simpler, and likely more successful. For the foreseeable future, the use of ECMO for PARDS will remain largely a clinical decision.

Full access? Get Clinical Tree
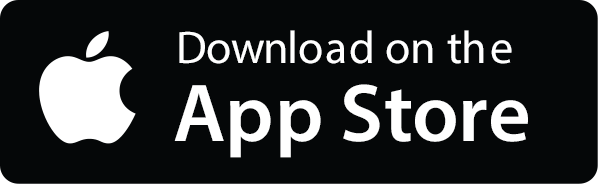
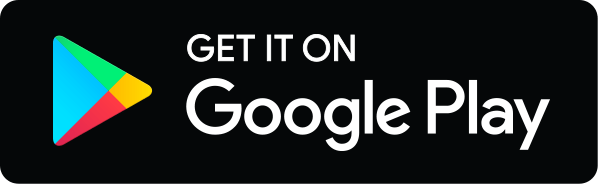