Fig. 11.1

Fig. 11.2

Fig. 11.3

Fig. 11.4

Fig. 11.5

Fig. 11.6
11.1.2 Primary Extracardiac Fontan Operation with Fenestration

Fig. 11.7

Fig. 11.8

Fig. 11.9
11.1.3 Primary Lateral Tunnel Fontan Operation with or without Fenestration

Fig. 11.10

Fig. 11.11

Fig. 11.12

Fig. 11.13

Fig. 11.14

Fig. 11.15

Fig. 11.16
11.2 1½ Ventricular Repair

Fig. 11.17

Fig. 11.18

Fig. 11.19

Fig. 11.20
Perhaps the most successful application of this strategy is for Group A patients, who have small but functioning right ventricles with distinct inlet, body, and outlet portions (Fig. 11.17). In general, this operation can be used for patients with pulmonary stenosis/atresia and intact ventricular septum who have a functioning right ventricle that is at least 40% of normal. Some surgeons leave an atrial fenestration, but the right-sided bypass in the form of the bidirectional Glenn shunt obviates the need for this strategy, ensuring 100% (or near 100%) systemic oxygen saturation.
Group B patients (Fig. 11.18) are those with a poorly functional right ventricle, as seen in some patients with advanced Ebstein’s anomaly of the tricuspid valve. Unloading the right ventricle in this situation shifts the Starling curve to the left, which may improve function.
Figure 11.19 shows the 1½ ventricular repair as applied to Group C, patients with a left SCV that drain to the dome of the left atrium, causing cyanosis. Intra-atrial baffles are prone to obstruction and arrhythmias, and this strategy allows left SCV flow without ligation. The hemodynamic result is favorable unless there is a left pulmonary artery stenosis distal to the entry of the anastomosis of the SCV and pulmonary artery, which results in intractable headaches and systemic venous hypertension. Care must be taken with this anastomosis as it tends to be difficult owing to the location that is often well into the left side of the mediastinum.
Perhaps the most interesting application of the 1½ ventricular repair is in Group D, patients with congenitally corrected transposition of the great arteries (ccTGA; Fig. 11.20). This strategy is discussed and illustrated in Chap. 10 for those patients with ccTGA and pulmonary stenosis, with or without ventricular septal defect (VSD). The idea is to construct a physiologic repair (right ventricle to aortic continuity) without having to resort to a conduit from the left ventricle to the pulmonary artery, which complicates the repair by coronary artery problems and conduit changes over time. The operation is performed by VSD closure (if present), pulmonary artery valvotomy/subpulmonic resection, and right atrial unloading by a bidirectional Glenn shunt to lower the developed pressure in the left ventricle. This helps by keeping the left ventricular pressure sub-systemic for optimal coronary artery flow and helps to stabilize the interventricular septum. Stabilizing the septum prevents right ventricular distention and help to avoid tricuspid regurgitation on the basis of papillary muscle stretching. This strategy actually works. The second application of the 1½ ventricular repair for patients with ccTGA is in double switch operations when the surgeon feels that the atrial baffle is too complex to include the SCV flow. Under these circumstances, a pericardial baffle is constructed to direct ICV flow to the tricuspid valve and right ventricle, which is to become the pulmonary ventricle after the arterial switch operation. The SCV flow is resolved by a bidirectional Glenn shunt, thereby completing the 1½ ventricular repair.

Fig. 11.21

Fig. 11.22

Fig. 11.23
11.3 Fontan Conversion and Arrhythmia Surgery
Management of patients with failing Fontan circulation has been the focus of significant scientific and clinical inquiry over the past decade, owing to the realization that the obligatory high venous pressure that characterizes this unique physiology has resulted in protein-losing enteropathy, plastic bronchitis, liver failure, liver cancer, atrial arrhythmias, pathway obstructions, valvar insufficiency, aneurysm formation, ventricular dysfunction, and decreased functional class. This litany of potential complications becomes more significant and more likely as the patient ages. As a result, one could expect that most, if not all, patients with Fontan physiology will have to undergo therapeutic interventions that include intense medical therapy, pacemaker placement, Fontan conversion, and eventually, cardiac transplantation. This chapter centers around good candidates for Fontan conversion, which was introduced in 1994 for select patients with failing Fontan physiology and atrial arrhythmias. The operation comprises conversion to extracardiac connections of vena cava to pulmonary artery, arrhythmia surgery, and pacemaker placement. The benefits of this operation have been confirmed by many clinical groups worldwide.
Patients have done best when there was preservation of ventricular function and absence of protein-losing enteropathy. The long-term effects of this operation are to improve functional status, treat debilitating arrhythmias, repair pathway obstructions, repair valves, and delay cardiac transplantation. For the most part, this operation has been highly effective. Over the years, we have been confronted with many impediments that were resolved intraoperatively. These techniques are noted in this chapter.
11.3.1 General Principles
From the onset of the operation, Fontan conversion is attended by potential complications relating to multiple sternotomies and the realization that an unwanted intracavitary entry can cause hemodynamic compromise and instability that can very quickly result in cardiovascular collapse. The driving force for cardiac output is controlled by venous pressure to the pulmonary artery. Any instability in this regard, even if effectively controlled, can result in renal failure, ventricular dysfunction, and ischemic brain injury. Patients with giant right atria that are adhered to the underside of the sternum may require peripheral cannulation and partial bypass before resternotomy. If femoral cannulation is employed, every effort should be made to convert to aortobicaval cannulation with femoral vessel repair to avoid limb ischemia, swelling, and compartment syndrome.
Once successful mediastinal reentry is performed, strategic and careful dissection is carried out to ensure hemostasis. Both thoracic cavities must be entered, with lysis of pulmonary adhesions to enable effective tube thoracostomy during the closing stages of the operation. The required optimal exposure is usually facilitated by self-retaining mammary retractors for hemostasis. Excessive postoperative bleeding after the Fontan operation is to be avoided. Once comprehensive dissection is achieved, aortobicaval cardiopulmonary bypass is instituted, with slow systemic cooling.
At this point in the operation, the surgeon must implement the preoperative plan to accomplish all the parts of the operation in the proper order. Every patient needs a period of aortic cross-clamping and cardioplegic arrest, if for no other reason than to perform the atrial septectomy. Other considerations include valve repair, pathway revisions, aneurysm resection, pulmonary artery augmentation, and left-sided maze procedures. The following are some of the characteristics of the Fontan conversion operation.
11.3.2 Uncomplicated Fontan Conversion with Modified Right Atrial Maze Procedure and Epicardial Pacemaker Lead Placement

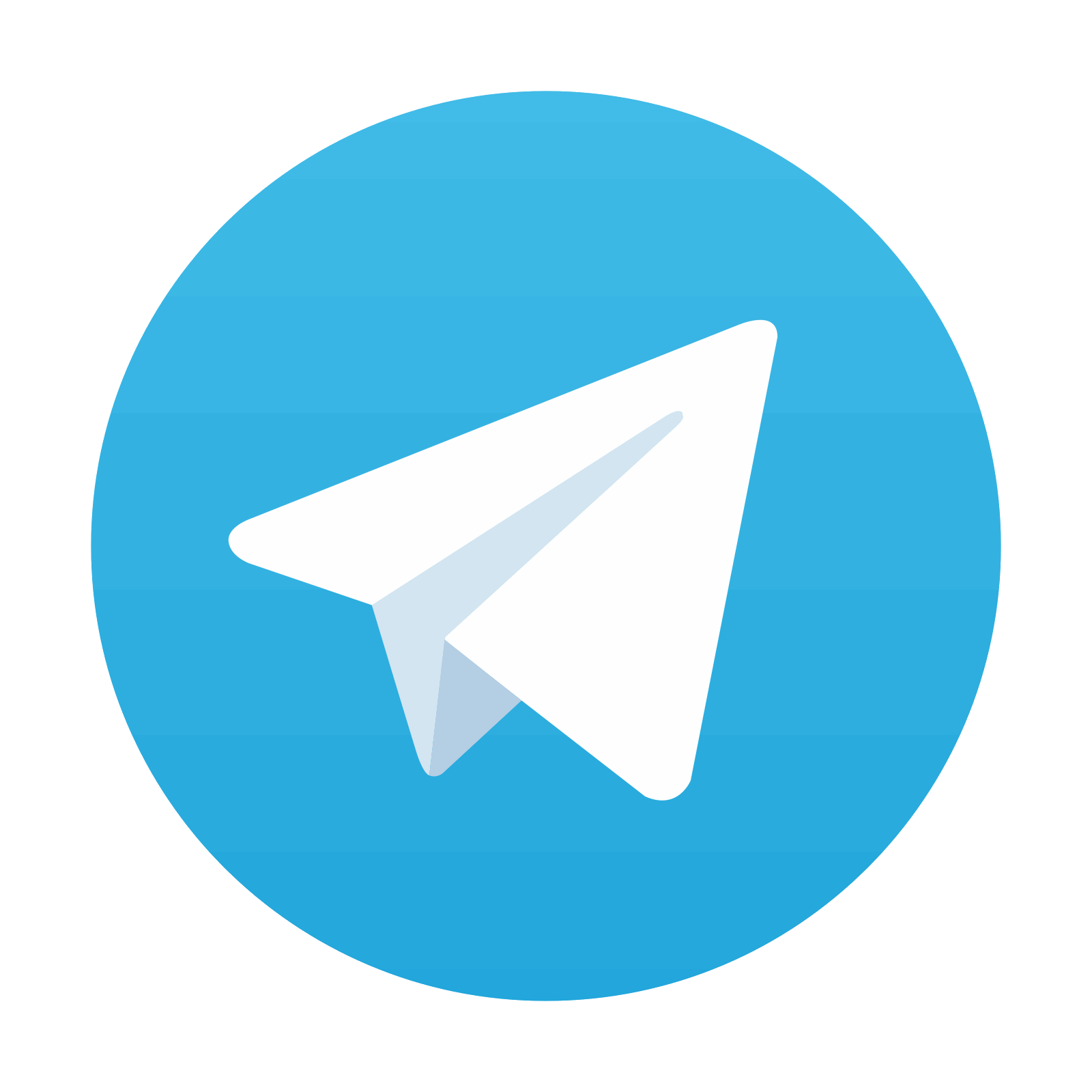
Stay updated, free articles. Join our Telegram channel

Full access? Get Clinical Tree
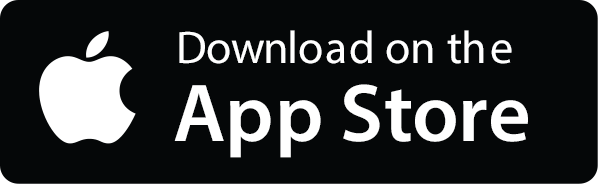
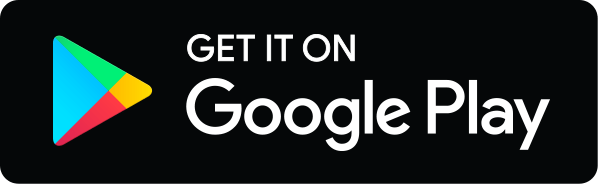