Kawasaki disease (KD) is a form of systemic vasculitis that causes chronic changes in arterial walls, including pulmonary arteries. The aim of this study was to test the hypothesis that pulmonary arterial wall properties and hemodynamics are abnormal after the resolution of KD. Pulmonary arterial input impedance was measured during cardiac catheterization (4.8 ± 4.5 years after disease onset) in 47 consecutive patients (mean age 7.8 ± 5.7 years) with KD and coronary artery lesions (CALs) in the acute phase and 42 control patients (mean age 6.7 ± 4.6 years). Patients with KD were subdivided into 2 groups: 28 with persistent CALs and 19 with regressed CALs. There were no significant differences in characteristic impedance and peripheral vascular resistance between patients with KD and controls. Compared with controls, patients with persistent CALs had significantly lower pulmonary arterial compliance, suggesting increased wall stiffness of the peripheral pulmonary vascular bed (p <0.05, analysis of variance). Patients with persistent CALs also exhibited increased wave reflection compared with other groups (p <0.05, analysis of variance). In conclusion, unlike patients with regressed CALs, patients with persistent CALs have abnormal mechanical properties and hemodynamics of the pulmonary artery after KD. Together with previous reports of abnormal properties of coronary and systemic arteries, these data suggest that KD vasculitis causes chronic changes in arterial wall properties in the entire arterial system to varying degrees and extent. The fate of these abnormalities in the pulmonary bed and other arterial systems and their potential adverse effects must be monitored in long-term follow-up.
Kawasaki disease (KD) is an acute inflammatory syndrome that causes systemic vasculitis. The coronary arteries appear to be most vulnerable to this inflammatory insult, which results in arterial wall destruction and consequent aneurysmal dilation in some affected patients. Importantly, mounting evidence suggests that in addition to the coronary arteries, the systemic arterial bed also exhibits structural and functional abnormalities years after KD, particularly in patients who had developed coronary artery lesions (CALs) during the acute phase, regardless of the persistence or regression of CALs thereafter. Notably, studies on autopsy cases of KD have shown that structural changes similar to those observed in the coronary and systemic arteries are also observed in the pulmonary arteries, raising the possibility that pulmonary arterial wall properties may be altered, and thereby pulmonary hemodynamics may be affected, in patients with KD. Pulmonary arterial wall properties and hemodynamics after KD have not yet been examined. The present study was conducted to test our hypothesis that pulmonary arterial wall properties are abnormal and that these are associated with abnormal pulmonary arterial hemodynamics after KD. For this purpose, we used input impedance analysis, which integrates functional and structural changes in vascular system and provides comprehensive information about the state of the vascular bed, including both frequency-independent static and frequency-dependent dynamic components.
Methods
We prospectively enrolled 47 patients with KD who underwent cardiac catheterization and coronary angiography. These patients had developed CALs, evidenced by echocardiography (coronary arteries with diameters ≥4 mm), during the acute phase. The patients were divided into 2 groups on the basis of angiographic findings: 28 patients with persistent CALs and 19 patients with regressed CALs. Data were compared with those from subjects in the control group, which consisted of 42 patients who had ventricular septal defects with negligible shunting (pulmonary/systemic blood flow ratio <1.1) but no evidence of vasculitis. Catheterization had been performed for ventricular septal defects until 1999 to check for deformity of aortic valves and its relation to ventricular septal defect, according to our institutional protocol at that time. Written informed consent was obtained from the parents of all patients, and the procedures followed the Hospital regulations of Saitama Medical University regarding 1) contract business control, 2) prevention of unfair research activities, 3) conflict of interest control, and 4) intellectual property.
Cardiac catheterization was performed according to the same anesthesia protocol for all subjects (premedication with intramuscular pethidine and atropine and sedation with continuous infusion of sodium thiamylal during catheterization). The pressure and flow velocities of the main pulmonary artery were simultaneously measured using a high-fidelity Doppler flow velocity probe tipped on a 0.014-inch guidewire (Volcano Corporation, San Diego, California). The measurements were stabilized by placing the guidewire inside a balloon-tipped catheter. Pressure and flow velocity signals were displayed in real time and stored for subsequent off-line analysis after digital conversion at 500 Hz.
Two to 4 consecutive steady-state beats during exhalation were signal averaged and used for impedance analysis. The flow signals were converted to flow volume data using cardiac output derived from aortic volume flow by echocardiography. Impedance moduli were calculated by Fourier analysis for pressure and flow data. Pulmonary vascular resistance was calculated by dividing the difference between mean pulmonary artery pressure and pulmonary capillary wedge pressure by cardiac index. Cardiac index was calculated by normalizing cardiac output to the body surface area. The characteristic impedance (Zc) was calculated by averaging all impedance moduli from 3 to 10 Hz, which provided an index for the arterial stiffness of the proximal pulmonary artery. Pulmonary artery compliance (Cp) was calculated on the basis of the method reported by Liu et al as Cp = stroke volume index/K(Ps − Pd), where Ps is pulmonary arterial pressure at the incisura, Pd is pulmonary arterial diastolic pressure, and K is an area index obtained by dividing the total area under the pulmonary arterial pressure curve by the diastolic area. While Zc denotes arterial wall stiffness of the proximal pulmonary artery, Cp indicates the stiffness of the more distal part of the arteries. The pulsatile wave is composed of forward and backward waves, the latter produced by a wave reflection at heterogenous areas of the pulmonary vascular bed. Information regarding wave reflections was obtained by calculating reflection coefficient as the difference between minimum and maximum input impedance moduli from 3 to 10 Hz.
All data are expressed as mean ± SD. Differences among the 3 groups were tested using analysis of variance with post hoc analysis by Bonferroni correction. Multivariate regression analysis was performed to determine the difference in Cp among the 3 groups after adjusting for age and blood pressure. For all statistical analyses performed, differences were considered significant at p <0.05.
Results
Patient characteristics for each group are listed in Table 1 . The mean age of patients with persistent CALs was significantly higher than that of patients in the other 2 groups (p <0.05). The mean febrile period during the acute phase was significantly longer in the persistent CAL group than that in the regressed CAL group. The mean follow-up period was about 5 years for the 2 KD groups.
Variable | Patients With CALs | Controls | |
---|---|---|---|
Persistent | Regressed | ||
Age (years) | 8.8 ± 5.5 (0.7–19) ⁎ , † | 5.6 ± 5.3 (1.1–19) | 6.7 ± 4.6 (0.5–21) |
Fever stage interval (days) | 9.2 ± 5.5 (3–16) ⁎ | 6.1 ± 4.5 (3–15) | |
Course (years) | 5.0 ± 4.7 (1–16) | 4.8 ± 4.3 (1–14) |
⁎ p <0.05 versus patients with regressed CALs;
Table 2 lists the data on pulmonary impedance and hemodynamics. The mean values of heart rate, cardiac index, and systolic blood pressure were similar in all the 3 groups. Zc and pulmonary vascular resistance were also similar among the 3 groups. However, Cp was significantly lower in patients with persistent CALs than in the other groups. The results were similar when age and blood pressure, which are known to affect Cp, were taken into account in multivariate analysis (Cp = 0.004 × pulmonary artery pressure + 0.08 × age −0.1 × group + 0.07; p <0.05), indicating that the stiffness of the peripheral pulmonary arterial wall was increased after KD in those with persistent CALs but not in those with regressed CALs. In addition, pulmonary arterial wave reflections were significantly augmented after KD in patients with CALs compared to those with regressed CALs and controls, as indicated by the significantly higher value of the reflection coefficient in patients with persistent CALs than in other groups.
Variable | Patients With CALs | Controls | |
---|---|---|---|
Persistent | Regressed | ||
Heart rate (beats/min) | 108 ± 18 | 111 ± 16 | 108 ± 19 |
Cardiac index (L/min/m 2 ) | 4.3 ± 0.8 | 4.2 ± 0.7 | 4.6 ± 0.7 |
Systolic blood pressure (mm Hg) | 99 ± 14 | 95 ± 14 | 98 ± 13 |
Characteristic impedance (dyne · s · cm −5 · m 2 ) | 49 ± 28 | 44 ± 16 | 45 ± 22 |
Pulmonary vascular resistance (dyne · s · cm –5 · m 2 ) | 287 ± 117 | 274 ± 108 | 247 ± 80 |
Compliance (ml/mm Hg/m 2 ) | 5.2 ± 2.5 ⁎ , † | 8.4 ± 4.0 | 8.4 ± 5.4 |
Reflection coefficient (dyne · s · cm –5 · m 2 ) | 50 ± 38 ⁎ , † | 37 ± 26 | 36 ± 24 |
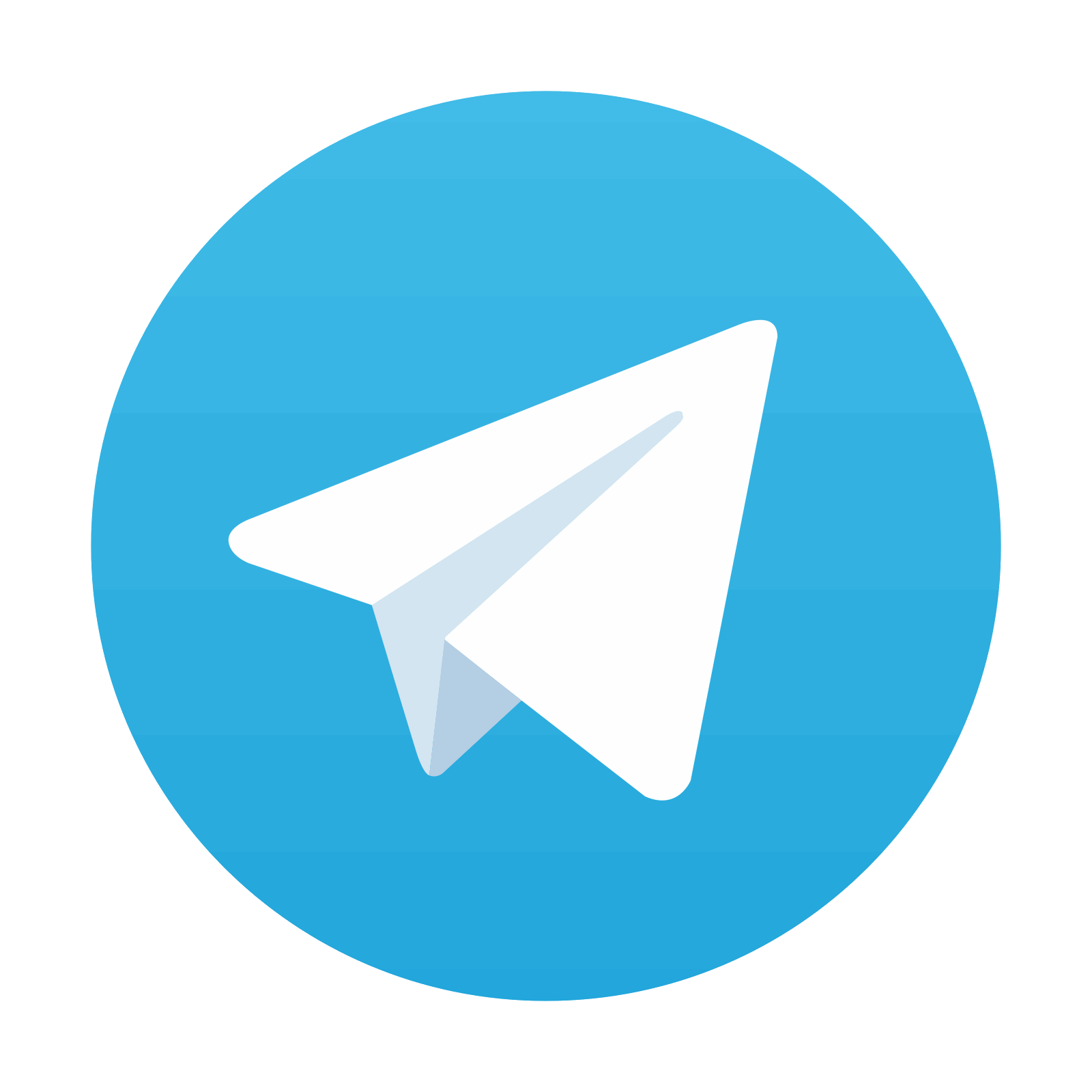
Stay updated, free articles. Join our Telegram channel

Full access? Get Clinical Tree
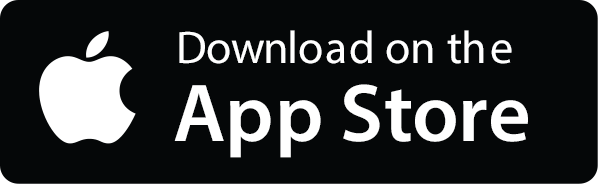
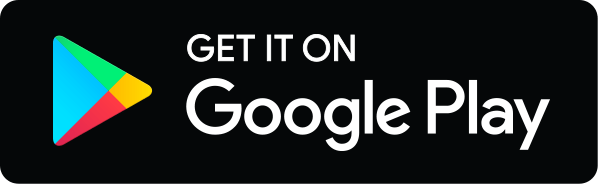
