Fig. 14.1
Pathobiology of pulmonary vascular remodeling in pulmonary hypertension. The pulmonary artery is normally characterized by a single endothelial monolayer, a smooth muscle medial layer, and an adventitial layer. As can be seen, the normally patent vessel becomes compromised due to fibrotic remodeling in all three layers (Figure modified from Nicholas Morrell, Cambridge UK, by kind permission)
The lung vasculature is subject to change from a large number of sources that originate in the lung or may derive from outside of the lung. These range from air pollution (Grunig et al. 2014), to airway disease (Alagappan et al. 2013), autoimmune disease (Colvin et al. 2013; Muller-Ladner et al. 2009), to ingestion of drugs (Seferian et al. 2013), or infection (Kolosionek et al. 2010). One biological theme common to most of these is lung inflammation, whether chronic or acute (Tuder et al. 2013). As will be discussed below, fibrocytes are cells in the circulating blood that can directly and indirectly influence process of wound healing and fibrosis. Given the central underpinning of inflammatory cells and processes in PVR, controlling the fibrocyte represents a potentially powerful therapeutic paradigm. Next, we summarize the last 20 years of research into fibrocytes, with particular focus on their putative contributions to PVR and the question of their “stemness.”
14.3 Fibrocytes
Fibrocytes are peripheral blood mononuclear cells (PBMCs) of hematopoietic origin that have been identified as key players at sites of tissue injury and fibrosis in a wide range of disease settings. Since the original identification of fibrocytes in 1994, there has been a steady growth of findings leading to a better understanding of their functional roles. The subsequent sections temporally map the studies that first established the identity of fibrocytes and the observations that have placed fibrocyte cell subsets as important controllers of a myriad of functions related to fibrosis. Finally, we weigh the evidence for and against the possibility that fibrocytes act as stem or progenitor cells, specifically in the context of PVR.
14.3.1 Discovery and Phenotypic Description
Landmark studies conducted by Bucala et al., using a model system of wound healing, led to the first identification of circulating fibrocytes in 1994 (Bucala et al. 1994). This novel subpopulation of leukocytes emerged after a few weeks in culture with high serum and was characterized as expressing CD34, CD11b, CD18, collagen I, collagen III, and vimentin. Morphologically, fibrocytes appeared as adherent, spindle-shaped cells reminiscent of primary fibroblasts in culture. They were localized to regions of scar formation and were suspected of being important modulators in pathological fibrotic responses (Bucala et al. 1994). This study and other initial observations of fibrocytes included them being referred to as “blood-borne fibroblasts” and “fibroblast-like cells” (Quan et al. 2004). Collectively, these data reinforced earlier studies in 1969 by Stirling and Kakkar, who used cannulation and diffusion chamber techniques to demonstrate that collagen-producing cells were derivatives of circulating blood and not elements dislodged from the walls of blood vessels (Stirling and Kakkar 1969). Interestingly, Bucala et al. speculated that fibrocytes could be required early on in wound healing and/or could contribute to excessive scar formation (Bucala et al. 1994).
It is well established that disturbances (infection, trauma, etc.) in tissues elicit wound repair responses from the host. Injury leads to release of chemokines and the recruitment of diverse cell populations to the site of injury. The wound repair response can be divided into four phases: (1) clot formation, (2) inflammation, (3) cell migration and proliferation, and (4) remodeling and resolution (Cordeiro and Jacinto 2013). Neutrophils along with monocytes are the first key players to appear at the site of injury during the inflammatory phase. Their function is to remove debris, clot, or pathogens that might be present in the wound (Cordeiro and Jacinto 2013). Through specific signal transduction processes, monocytes are able to mature into diverse sets of macrophages as well as fibrocytes, and in turn are able to secrete chemotactic cytokines and growth factors (Aggarwal et al. 2014). Through activation of context-specific gene programs, macrophages work to promote immunity, activate fibroblasts, augment cell proliferation, and to stimulate angiogenesis (Molawi and Sieweke 2013). In skin, the epithelium is responsible for covering the wound in the subsequent proliferative phase and activated fibroblasts produce collagen actively along with other matrix proteins, while new matrix is remodeled in the following maturation phase (Kendall and Feghali-Bostwick 2014). The newly organized matrix, or granulation tissue, provides tensile strength to the site of inflammation and the integrity of the injured tissue is restored more or less to the original state. It should be noted that stem cell niches in the lung tissue itself, while normally low in turnover rate, are activated to proliferate in response to injury (Wansleeben et al. 2013). Although the specific details regarding the identity and locations of putative lung stem cells have been a challenging task to date, it is likely that both lung-resident and non-lung-resident stem and progenitor cells contribute to injury responses (Weiss et al. 2008). Since the first functional studies of circulating fibrocytes that showed their presence in exudate fluid (Quan et al. 2004), and their rapid recruitment to injury sites and production of collagen (Bucala et al. 1994), fibrocytes have been investigated in numerous aspects of tissue repair.
Peripheral blood fibrocytes express CD34 cell surface antigen (Quan et al. 2004), a marker common to hematopoietic stem cells of both myeloid and lymphoid lineage (Bucala et al. 1994; Brown et al. 1991). CD34 expression often decreases with passing time in culture or when hematopoietic cells enter into tissue, a finding confirmed in culture and in vivo (Bucala et al. 1994; Aiba and Tagami 1997). The presence of collagen and its co-expression with unique hematologic markers combined with morphology is usually the minimum criteria to characterize fibrocytes (Bucala et al. 1994; Chesney et al. 1997). Fibrocytes have been found to express a wide variety of cell surface proteins (Pilling et al. 2009) and immune receptors (Crawford et al. 2012), for which a large number of putative functions can be deduced. It was the discovery of the class II major histocompatibility molecule (MHC) HLA-DR being expressed by fibrocytes that led to studies investigating their ability to present antigens (Chesney et al. 1997). Studies in human and mice PBMC led to the affirmation that fibrocytes express the necessary catalog of cell surface proteins requisite for antigen presentation and they readily migrate to lymph nodes and sensitize T lymphocytes (Chesney et al. 1997). Similar results have been found in a model of hepatotoxic injury (Kisseleva et al. 2011) and thus position fibrocytes as key bone marrow-derived cells capable of linking innate and acquired immune systems. Future studies that interrogate other fibrocyte surface markers should provide functional context for their phenotypic expression.
14.3.2 Roles in ECM Biology and Inflammation
Since their initial discovery, more than 300 papers have investigated functional aspects of fibrocyte biology, with a strong emphasis on the ECM remodeling properties of fibrocytes. Fibrocytes differ significantly from the resident tissue fibroblast with respect to the level of expressions of various collagen types (I, III, V, and VI), matrix proteoglycans, and hyaluronan (Bellini and Mattoli 2007; Pilling et al. 2009). Following an initial differentiation from monocytic precursor(s), transforming growth factor beta (Abe et al. 2001) and endothelin-1 (Schmidt et al. 2003) can induce further differentiation into fibroblast and myofibroblast-like cells. Collagen I, III, and V are fibrillar collagens, while collagen VI is non-fibrillar (Myllyharju and Kivirikko 2004). The collagens type I, III, and V can be assembled differently and comprise distinct structural components of the ECM (Ushiki 2002). Collagen VI, on the other hand, forms a network of beaded filaments (Myllyharju and Kivirikko 2004). Comparison of cultured human fibrocytes to cultured human fibroblasts showed significantly lower expressions of mRNA encoding the chains for collagen type I, III, and V in fibrocytes, but expressed comparable levels of mRNA-encoding collagen type VI (Bianchetti et al. 2012). Fibrocytes express lower levels of mRNA-encoding proteoglycans (PGs) such as decorin, but transcribe high levels of mRNA-encoding PGs like perlecan and versican (Bianchetti et al. 2012). Additionally, fibrocytes express higher levels of mRNA encoding for hyaluronan synthase 2. Fibroblast and fibrocytes are both able to incorporate the collagen and PGs actively secreted into the matrix (surrounding as well as underlying), with fibrocytes predominantly producing the proteins associated with the membrane (Bianchetti et al. 2012). However, fibrocytes produce collagens that are deposited into the matrix at a much lower capacity than do fibroblasts (Bianchetti et al. 2012). Collagen VI, hyaluronan, versican, and perlecan modulate cell–to–cell interaction as well as cell–to–matrix interactions (Wu et al. 2005; Hirose et al. 2001; Howell and Doane 1998; Myllyharju and Kivirikko 2001; Iozzo 1998). Perlecan is known to interact with other matrix components, bind growth factors, regulate permeability of the basement membranes, and promote angiogenesis (Zhang et al. 2004). Moreover, the PGs assist in maintaining tissue integrity by regulating stress transfer between the cytoskeleton, cell membrane, and the matrix (Iozzo 1998; Al-Jamal and Ludwig 2001). Fibrocytes, by virtue of their ability to secrete high levels of perlecan, versican, hyaluronan, and collagen type VI (relatively high levels in comparison to other ECM proteins), are primary modulators in responses to inflammation and tissue stabilization during wound healing process (Mattoli et al. 2009; Bellini and Mattoli 2007; Mousavi et al. 2005; Madsen et al. 2007; Wienke et al. 2003).
Fibrocytes adhere to fibronectin through integrin β1 on their cell surface and to immobilized matrices. Blocking the binding of integrin β1 decreases the adhesion significantly but not entirely (Bianchetti et al. 2012). Phenotypic analysis led to the revelation that fibrocytes express other collagen receptors, in addition to integrin β1. Through a series of blocking experiments, one such receptor involved in adhesion to collagen was the mannose receptor Endo180. Endo180 is not only responsible for adhesion of cells to collagen, but also promotes the uptake of collagen and collagen fragments by alternatively activated macrophages and hepatic stellate cells (Engelholm et al. 2003; Behrendt et al. 2000). The bound collagen is transported to the late endosome and then routed to the lysosome for degradation. Fibrocytes were able to internalize fragments of collagen type I more efficiently than non-denatured collagen type I or intact collagen VI, and collagen uptake is inhibited when excess amounts of uPA is present (Bianchetti et al. 2012).
Besides direct effects on ECM protein turnover, fibrocytes play important roles in chronic inflammation that impact the matrix. Fibrocytes secrete interleukin (IL)-6, IL-8, chemokine (C-C) motif ligand 2 (CCL2, also known as monocyte chemoattractant protein-1, or MCP-1), CCL3, as well as intercellular adhesion molecule-1 (ICAM-1) in response to interleukin-1 (Chesney et al. 1998). Thus, fibrocytes, upon entry into injured tissue, amplify and modify subsequent immune responses. Such responses can greatly influence tissue remodeling. For example, fibrocytes from patients with asthma respond to Th2 cytokines by producing collagen (Bellini et al. 2012). IL-33, an IL-1 cytokine family member, enhances proliferation of fibrocytes and augments their output of IL-13 and IL-5 (Hayashi et al. 2014), both of which are Th2 cytokines implicated in asthma pathobiology. IL-33 also induces fibrocyte MMP-9 activity, which in turn may activate latent transforming growth factor beta (TGF-beta), a pleiotropic growth factor and controller of matrix biology and fibrosis (Hayashi et al. 2014). In addition to crosstalk with innate immune cells, fibrocytes communicate with lymphocytes. Regulatory T lymphocytes (Tregs) can inhibit the recruitment of fibrocytes, which decreases the extent of tissue remodeling in the murine model of lung fibrosis by inducible TGF-beta over-expression (Peng et al. 2014). This recent finding is of potentially major importance since deficiency of Tregs appears to mechanistically underpin the development of PVR in both pulmonary hypertension (Tamosiuniene et al. 2011) and IPF (Kotsianidis et al. 2009). Intriguingly, fibrocytes have also been shown to contribute to other (later) stages of the inflammatory response, including resolution. Recently, it was shown that circulating fibrocytes stabilize blood vessels in vitro and in vivo during angiogenesis (Li et al. 2014). The mechanism appeared to be twofold in that fibrocytes inhibited angiogenesis and prevented regression of existing vessels (Li et al. 2014). Thus, the paradigm that fibrocytes are pro-antigenic (Grieb et al. 2011) may now need to be nuanced to include antagonistic functions depending on context. Such a concept may extend to other functions of fibrocytes, as tumor-associated fibrocytes suppress immune cell functions via interferon gamma and tumor necrosis factor (Kraman et al. 2010).
Taken collectively, these data indicate that fibrocytes are endowed with a great degree of phenotypic plasticity that influences, and is influenced, by local cues within the ECM and from cells at sites of injured ECM. Future studies will need to parse these cues and specifically determine what controls fibrocyte differentiation and phenotypic specialization.
14.3.3 Differentiation
Fibrocytes are a bone marrow-derived cell population that express both hematopoietic and stromal cell markers. As discussed above, fibrocytes are key modulators in wound healing, angiogenesis, and pathological fibrosis. Much of what is known about fibrocyte differentiation comes from culture of peripheral blood. The effect of serum on the differentiation and function of fibrocytes is quite pronounced. In the Bucala study, 20 % serum-containing media was used and fibrocytes emerged from such culture conditions in weeks. In contrast, recent studies conducted by Curnow et al., using in vitro conditions where serum is either present or absent, fibrocytes could be cultured in as few as 3 days in the absence of serum (Curnow et al. 2010). The study further indicated that the cells differentiated under serum-free and serum-containing conditions are indeed both fibrocytes, but possess distinct properties that could possibly relate to their proposed functions in vivo (Pilling et al. 2007). These data are in line with studies showing that serum amyloid p (SAP), a pentraxin family member, inhibits fibrocyte differentiation (Pilling et al. 2003). C reactive protein and SAP are liver-derived pattern recognition molecules that help to facilitate clearance of pathogens and cell debris (Hutchinson et al. 2000). Injections of SAP inhibit inflammation and fibrosis in murine models of pulmonary fibrosis (Murray et al. 2010, 2011; Pilling et al. 2007); however, in mice genetically lacking SAP, inflammation and fibrosis are relentless, indicating that a key endogenous function of SAP may be the promotion of fibrocyte differentiation to resolve inflammation and fibrosis (Pilling and Gomer 2014).
Fibrocytes are able to differentiate from CD14+ monocytes as well as from CD14− PBMC (Geissmann et al. 2008). Curnow et al. provide evidence that efficient generation of fibrocytes occurs from PBMC in serum-free conditions, while fibrocytes in the serum-containing conditions differentiate more efficiently from the CD14+ monocyte population. Regardless, both types of fibrocytes express the same range of markers (Curnow et al. 2010). This finding raises the possibility that the differentiated cells represent distinct types of fibrocyte that arise from different precursor cells and are present at different frequencies with the subpopulations of CD+ monocytes and PBMCs. A number of signaling molecules, including toll-like receptor ligands (Maharjan et al. 2010), semaphorins (Gan et al. 2011), as well as the glycosaminoglycan hyaluronic acid (Maharjan et al. 2011), can affect fibrocyte differentiation. Additionally, it was determined that a high number of gene pathways relating to lipid metabolism were significantly enhanced in serum-containing fibrocyte cultures. This finding led to the deduction that fibrocytes differentiated in serum possess multipotent properties and are able to differentiate to other mesenchymal cell lineages such as adipocytes, as supported by Hong et al. (2005). Whether fibrocytes differentiated in serum-free media retain a similar degree of multipotency is yet to be determined. It is also unclear exactly what micro-environmental cues in the lung constitute the critical paracrine signals and fibrocyte-matrix/fibrocyte-heterotypic cell interactions that might help to explain the putative roles for fibrocytes in PVR.
Fibrocytes have been established as a cell population that can differentiate into myofibroblast-like (Hong et al. 2007) and adipocyte-like cells (Hong et al. 2005). Fibrocytes possess the potential to differentiate into other mesenchymal lineage cells such as osteoblasts and chondrocytes (Choi et al. 2010). When cultured in osteogenic media in a manner similar to osteo-precursor cells, fibrocytes differentiated into osteoblast-like cells (Choi et al. 2010). Similarly, fibrocytes as well as mesenchymal stem cells (MSCs), when cultured in chondrogenic media, differentiated into highly similar chondrocyte-like cells (Choi et al. 2010). The results suggest that fibrocytes have the capacity to differentiate into different mesenchymal lineage cells. This raises the possibility that fibrocytes could be used for regenerative therapy not only with respect to osteo-related (bone) or chondro-related (articular cartilage) repair, but perhaps to remodel lung tissue compromised by PVR. These results, although tantalizing, have been challenged by some with regard to both terminology and methodology (Weiss et al. 2008). One of the primary reasons for such concerns is the difficulty in the unequivocal identification and fate mapping of fibrocytes, stem cells, and progenitor cells in tissues, especially the lung.
14.3.4 Tracking Fibrocytes
Fibrocytes are defined to be of hematopoietic origin and derived from monocyte lineage cells due to the expression of specific monocyte surface markers CD11b and the leukocyte marker CD45 (Bellini and Mattoli 2007; Reilkoff et al. 2011). The marker CD45 is currently considered the most specific in determining and characterizing cell lines of hematopoietic origin. As mentioned before regarding CD34, the expression of CD45 marker is not a reliable enough condition to distinguish between cells of hematopoietic and non-hematopoietic origin. CD45 is expressed on later stages of hematopoietic cell development (Zambidis et al. 2005), and hence is incapable (used in isolation) of completely identifying these cells. A problem arises when fibrocytes, during the course of differentiation, lose their expression of markers of their hematopoietic origins (Mori et al. 2005; Phillips et al. 2004), and it then becomes challenging to distinguish them from the fibroblasts that are residents in the tissue of interest. The complex cell membrane protein expression pattern, the transient nature of their expression, and the requirement to co-identify a mesenchymal (intracellular) marker, all contribute to the controversial nature of fibrocyte fate tracing. There have been studies that investigated the involvement of bone-marrow-derived cells that are able to produce collagen in response to tissue injury (Mori et al. 2005; Fathke et al. 2004; Barisic‐Dujmovic et al. 2010), but have produced results unable to distinguish the true lineage of these cells. This is due to the methods used to track the mesenchymal cells as well as that the hematopoietic cells are similar to resident cells in the models designed (Suga et al. 2014). To effectively be able to determine the presence and the long-term fate of hematopoietic cells, Suga et al. proposed to distinguish hematopoietic cells from non-hematopoietic cells by utilizing Vav 1 gene expression (Suga et al. 2014) and shying away from previous mouse models that targeted either selected lineage cells or specific differential stages (Adams et al. 1999). Vav 1 is a pan-hematopoietic marker that is found expressed by hematopoietic cells and no other tissue (Bustelo et al. 1993; Moores et al. 2000), and whose presence is advantageous in differentiating between cells of hematopoietic origin and non-hematopoietic lineages. Avoiding reliance on the surface markers previously used to identify fibrocytes (Bellini and Mattoli 2007; Reilkoff et al. 2011), Suga et al. used the Vav 1 gene to irreversibly label the cells of hematopoietic origin with Green Fluorescent Protein (GFP) and concomitantly label the non-hematopoietic cells with red fluorescent protein (RFP) (Suga et al. 2014). While examining the influx of different cell types during an injury response, they were able to obtain evidence confirming the existence (presence) as well as the prevalence of the cells over the time span of wound repair. Interestingly, using this novel double transgenic approach, two populations of col I+ cells were found: CD45+/CD11b+ and CD45−/CD11b− cells. The former were found to be fibrocytes and to express alpha-smooth muscle actin (SMA) in early areas of wound healing.
With regard to PVR and fibrocyte tracking, a few studies deserve mention. First, hypoxia-induced pulmonary hypertension in bone marrow-transplanted mice was associated with increased CD45+/GFP+ (donor derived)/collagen I+ lung fibrocytes (Nikam et al. 2010). Use of the clinically approved vasodilatory prostacyclin analog treprostinil (Seferian and Simonneau 2013), dramatically lowered the fibrocyte numbers in the lung, attenuated some of the PVR, but did not improve right heart function (Nikam et al. 2010). In a rat model of irreversible pulmonary hypertension, sex mismatched donor marrow-derived GFP+ cells were observed in areas of PVR and fibrosis (Spees et al. 2008). Many of these cells adopted fibroblast and myofibroblast morphologies, and a small number appeared as bronchial epithelial cells. Whether these cells were fibrocytes or MSCs, as had been earlier reported (Kotton et al. 2001; Ortiz et al. 2003), was not determined from these studies.
14.4 Are Fibrocytes Stem Cells, Progenitor Cells, or Neither and Are they Pro-fibrotic or Pro-resolution?
A bona fide adult stem cell should fulfill important criteria in order to meet the consensus definition (Weiss et al. 2008). They must have the capacity for self-renewal. Generally, adult stem cells have the multipotent capacity to differentiate into cells of the parent tissue. MSCs are stromal-derived cells that self-renew and demonstrate the capacity to differentiate into a variety of cell lineages. The minimal criteria for MSC, as developed by the MSC Committee of the International Society for Cellular Therapy, include (1) plastic adherence in standard tissue culture conditions; (2) expression of CD73, CD90, CD105; (3) no expression of CD11b, CD14, CD19, CD34, CD45, CD79alpha, or HLA-DR; and (4) differentiation in vitro to osteoblasts, adipocytes, and chondroblasts (Dominici et al. 2006). As we have presented above, fibrocytes are a subset of circulating leukocytes that co-express mesenchymal proteins such as collagen or vimentin and home to regions of tissue injury, including the injured lung. Currently, there is no evidence that fibrocytes, despite their ability to differentiate into a number of cell types in response to cytokines, are stem cells. Although they can proliferate, it remains unclear what capacity for self-renewal they may have. Although they fulfill criteria #1 and #4 above for MSC, they do not express CD90 and do/can express CD11b, CD34, CD45, and HLA-DR; as such, they are not MSC. If fibrocytes do not fulfill the criteria for true stem cells, what are they? We suggest that fibrocytes are a population of bone marrow-derived cells endowed with unique abilities to be mobilized in response to tissue injury, home to that injury, and to participate in complex heterotypic interactions that are context-dependent. They are likely derived from CD14+ monocytes, but may also be derived from CD14− cells. A progenitor cell is defined as any proliferative cell that has the capacity to differentiate into different cell lineages within a given tissue. As discussed above, fibrocytes can differentiate into a variety of lung tissue cells. It is conceivable that fibrocytes may be able to differentiate into cell types, given the variable expression patterns of cell surface molecules. It is appropriate then to term fibrocytes as mesenchymal progenitor cells, as some authors have done (Bellini and Mattoli 2007).
It remains to be seen whether fibrocytes and other bone marrow-derived progenitor-like cells are pro-fibrotic or pro-resolution cells. It seems likely that the term fibrocyte encompasses one or more cell types derived from the bone marrow that are capable of rapid gene expression change and the adoption of a wide variety of unique phenotypes. At sites of PVR, they appear to play both direct and/or paracrine roles in pro-fibrotic or pro-resolution pathways depending on complex and poorly understood contextual cues (Fig. 14.2). Future studies will need to specifically address the following unresolved questions, among others. First, the term fibrocyte appears to mean many things to many investigators. Given the simple two-part requirement of expression of a hematopoietic marker and a mesenchymal marker, a large number of cells with distinct expression could be called fibrocytes. For example, a bone marrow-derived cell could express either CD45, CD34, or CD11b and could co-express either procollagen, SMA, or vimentin. This computes to 23 = 8 combinations and assumes any cell uniquely expresses only one hematopoietic and one mesenchymal marker. It is therefore clear that a fibrocyte could be an accurate term for dozens of potentially unique cell types with specialized functions. Going forward, the field should exercise caution in the reporting and evaluation of studies involving fibrocytes, being clear on exactly what phenotypes (as much as is possible) are under investigation. Second, there is no evidence that fibrocytes serve as stem cells in any tissue injury investigated to date. Rather, it seems their primary role is to influence the tissue injury site in a paracrine manner towards either a pro-fibrotic process or perhaps a pro-resolution mode. Studies are critically needed that detail what types of fibrocytes operate at sites of tissue injury, what signals are they specifically responding to, and what are the kinetics of their arrival, modulation of biological processes, and apoptosis or emigration out of the site. Third, studies in animal models are needed that test the ability of pharmacologic or immunology agents to shape the fibrocyte response to injury. These studies will be extraordinarily difficult in terms of cell tracking and the dissection of micro-environmental cues that contextualize the fibrocyte response. The successful pursuit of these critically important studies should eventually yield new therapies that control the fibrocyte phenotype to achieve the appropriate kinetics of appropriate resolution of inflammation and the amelioration of PVR.
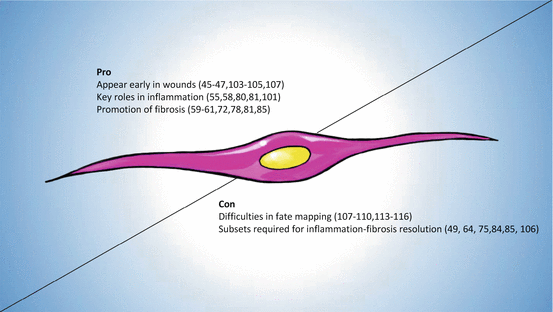
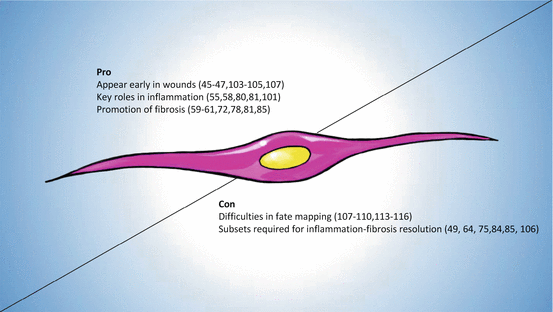
Fig. 14.2
Are fibrocytes pro-fibrotic or pro-resolution of fibrosis? Fibrocyte with classic spindle-shaped morphology, with references supporting roles for and against inflammatory fibrotic remodeling
References
Abe R, Donnelly SC, Peng T, Bucala R, Metz CN (2001) Peripheral blood fibrocytes: differentiation pathway and migration to wound sites. J Immunol 166(12):7556–7562PubMed
Adams J, Harris A, Strasser A, Ogilvy S, Cory S (1999) Transgenic models of lymphoid neoplasia and development of a pan-hematopoietic vector. Oncogene 18(38):5268–5277PubMed
Aggarwal NR, King LS, D’Alessio FR (2014) Diverse macrophage populations mediate acute lung inflammation and resolution. Am J Physiol Lung Cell Mol Physiol 306(8):L709–L725. doi:10.1152/ajplung.00341.2013 PubMedCentralPubMed
Aiba S, Tagami H (1997) Inverse correlation between CD34 expression and proline-4-hydroxylase immunoreactivity on spindle cells noted in hypertrophic scars and keloids. J Cutan Pathol 24(2):65–69PubMed
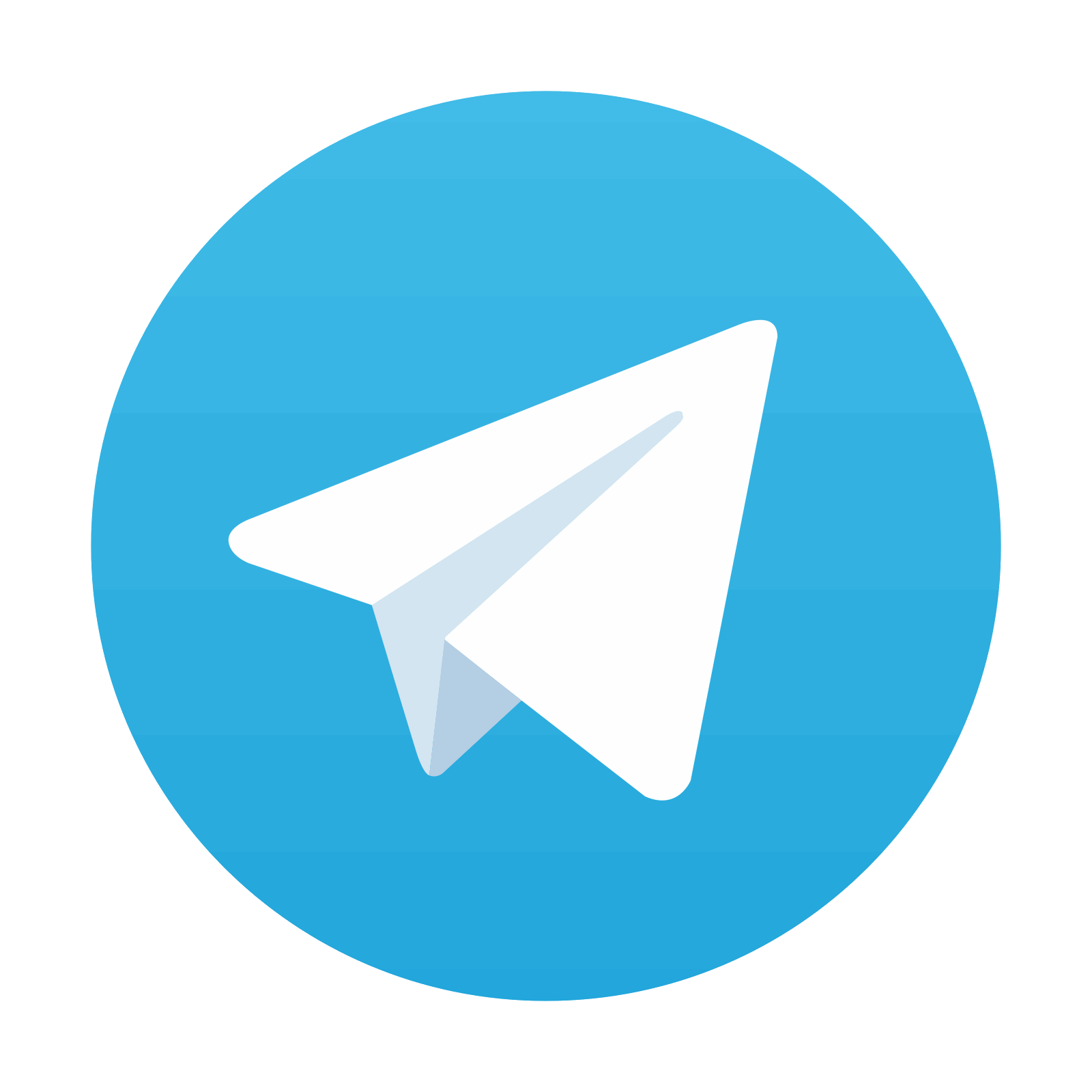
Stay updated, free articles. Join our Telegram channel

Full access? Get Clinical Tree
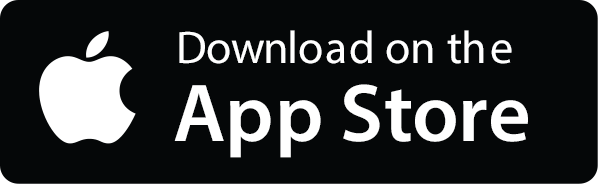
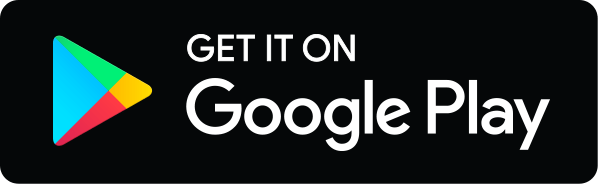