Background
Cardiac magnetic resonance imaging (CMR) is the gold standard for the quantification of global and regional myocardial function and can detect subclinical myocardial dysfunction in anthracycline-induced cardiomyopathy. The aim of this study was to ascertain reliable echocardiographic parameters that can be used for the early identification of cancer therapeutics–related cardiac dysfunction, compared with CMR.
Methods
Fifty-seven pediatric cancer survivors, 10 to 42 years of age, with cumulative anthracycline doses ≥ 200 mg/m 2 , were studied with transthoracic echocardiography and CMR 2.4 to 26.9 years after chemotherapy.
Results
Three-dimensional echocardiography had the highest sensitivity in identifying subjects with CMR-derived ejection fractions < 55%. Subjects with end-systolic volume index values > 29 mL/m 2 were more likely to have CMR-derived ejection fractions < 55%. Three-dimensional speckle-tracking echocardiographic peak global longitudinal strain magnitude < −17.5% best identified subjects with abnormal peak midwall longitudinal strain magnitude by CMR. A decrease in early atrial myocardial velocity of <10 cm/sec at the interventricular septum also identified subjects with lower average peak midwall longitudinal strain and peak midwall circumferential strain magnitudes by CMR.
Conclusions
Three-dimensional echocardiographic ejection fraction < 55%, end-systolic volume index > 29 mL/m 2 , three-dimensional speckle-tracking echocardiographic peak global longitudinal strain magnitude < −17.5%, and a decrease in early atrial myocardial velocity at the interventricular septum of <10 cm/sec by Doppler tissue imaging are the most sensitive transthoracic echocardiographic parameters to identify subjects with subclinical myocardial dysfunction by CMR.
Potentially cardiotoxic cancer therapy, including the use of anthracyclines, continues to be a cornerstone in the treatment of a variety of childhood cancers. Echocardiography is the most widely used imaging modality for the serial evaluation of left ventricular (LV) structure and function in patients exposed to anthracycline therapy. Unfortunately, traditional echocardiographic measures of global systolic function, including M-mode-derived shortening fraction and two-dimensional (2D) echocardiographic assessment of ejection fraction (EF) using the biplane method of disks (modified Simpson’s rule), typically remain within the normal range until late in the course of the disease. Furthermore, compared with CMR, they do not achieve the desired levels of sensitivity and specificity in individual patients.
Modern echocardiographic techniques, including three-dimensional (3D) echocardiography (3DE), Doppler tissue imaging, and speckle-tracking echocardiography (STE), have not been routinely incorporated in the early identification of anthracycline-induced cardiotoxicity in survivors of childhood cancer. Recent consensus guidelines by the American Society of Echocardiography and the European Association of Cardiovascular Imaging advocate the use of standard transthoracic echocardiographic techniques in addition to 2D strain and 3D imaging acquisition for the diagnosis of cancer therapeutics–related cardiac dysfunction (CTRCD) in adult patients during and after cancer therapy.
Cardiac magnetic resonance imaging (CMR) is the gold standard for the quantification of global and regional myocardial function and can detect subclinical myocardial dysfunction in the setting of a wide variety of myocardial disease processes, including anthracycline-induced cardiomyopathy. Early CMR features of CTRCD include low normal global LV systolic function with an increase in end-systolic volume and reductions in myocardial deformation parameters, including low midwall peak circumferential strain (ε cc ) and midwall peak longitudinal strain (ε ll ) magnitudes. The use of CMR is constrained by its availability and cost compared with transthoracic echocardiography (TTE), which limits its utility as a first-line technique for routine clinical surveillance of ventricular dysfunction. Limited data are available to formulate evidence-based recommendations for early echocardiographic diagnosis of CTRCD in pediatric patients with cancer exposed to anthracyclines. Accordingly, the objective of this study was to establish the best transthoracic echocardiographic indicators of early CTRCD and to ascertain the diagnostic performance of TTE-derived systolic, diastolic, and myocardial deformation parameters compared with CMR.
Methods
Study Population
For this single-center study, 57 eligible subjects were identified and prospectively enrolled through a registry of pediatric cancer survivors treated with anthracyclines between 1985 and January 2011. All subjects in the registry are followed at Connecticut Children’s Medical Center with yearly physical examinations and periodic echocardiography on the basis of age at treatment, radiation dose, and cumulative anthracycline dose. Inclusion criteria for this study are shown in Figure 1 . Age > 9 years was selected to avoid need for general anesthesia or sedation. Informed consent was obtained at the time of enrollment. The medical records of all enrolled subjects were reviewed to identify known risk factors associated with cardiotoxicity. Conversions to isotoxic equivalents of anthracycline antibiotics were performed to calculate total cumulative dose. This study was approved by the institutional review board at Connecticut Children’s Medical Center.

Echocardiography
Echocardiographic assessments were performed using a Philips iE33 echocardiographic system. TTE was performed for the evaluation of systolic and diastolic function, including 2D and 3D imaging, M-mode, spectral and tissue Doppler parameters of diastolic function, and measurements of ventricular afterload (end-systolic wall stress) and contractility (stress/velocity index), using American Society of Echocardiography guidelines.
Echocardiographic Image Analysis
Advanced postprocessing of echocardiographic images was performed on Xcelera version 3.11 (Philips Medical Systems, Best, The Netherlands) and QLAB version 9.0 (Philips Medical Systems). Systolic parameters were entered in the Boston Children’s Hospital database for calculation of Z scores. By entering height and weight, pediatric Z scores are automatically calculated. This database uses normative data obtained from 305 normal subjects. Data derived from these normal subjects are used to determine the normal range for each of the echocardiographic parameters on the basis of age, sex, and body surface area. Diastolic parameters and tissue Doppler indices were compared with published normative data in children. Two-dimensional myocardial strain analysis was performed on standard short-axis transthoracic echocardiographic images of the midventricle (at the level of the papillary muscles) and two- and four-chamber apical views. Sector size and depth were adjusted to achieve optimal visualization of the entire LV myocardium at the highest possible frame rate. Two-dimensional peak global circumferential strain (GCS) and global longitudinal strain (GLS) magnitudes were analyzed offline using vendor-independent clinical speckle-tracking echocardiographic software (2D Cardiac Performance Analysis version 1.2; TomTec Imaging Systems, Munich, Germany). Three-dimensional GCS and GLS were analyzed using 4D LV-Analysis version 3.1 (TomTec Imaging Systems) according to the American Society of Echocardiography’s 16-segment model for chamber quantification. To assess interobserver variability of strain analyses, a second expert reader performed the same analysis in a subset of eight randomly selected subjects in a blinded fashion (O.H.T.-S., S.W.). Similar to other investigators, we found radial strain (as measured by speckle-tracking in the short-axis parasternal view) to be unreliable, and therefore it was not included in the analysis. Normative 2D and 3D STE-derived GCS and GLS values were obtained in 12 age- and gender-matched control subjects (mean age, 22 ± 3 years). Three separate measurements of all systolic, diastolic, and myocardial deformation parameters were obtained and averaged for analysis.
CMR Techniques
Subjects were imaged on a 1.5-T (GE CV software version 16.0/M4; GE Medical Systems, Milwaukee, WI) using the following protocol: (1) standard multislice, multiphase cine imaging was performed using a steady-state free precession acquisition technique (fast imaging employing steady-state acquisition) in the two-chamber, four-chamber, and contiguous short-axis planes (steady-state free precession imaging parameters as follows: echo time, min full; repetition time, 3.4 msec; flip angle, 45°; bandwidth, 125; image matrix, 224 × 224; views per segment, 16), and (2) tagged cine images were acquired in the apical four-chamber and three short-axis planes (basal, midcavity, and apical) with an electrocardiographically triggered segmented k-space fast gradient echo sequence with spatial modulation of magnetization in orthogonal planes (grid tag spacing, 7–8 mm; field of view, 30–32 × 25–26 cm 2 ; slice thickness, 7–8 mm; flip angle, 12°; echo time, 3 msec; repetition time, 4.2 msec; views per segment, 6). CMR and TTE were performed on the same day.
CMR Image Analysis
Ventricular volumes, ventricular mass, and EF were assessed via standard planimetric techniques with semiautomated computer software (QMASS version 6.1.5; Medis Medical Imaging Systems, Leiden, The Netherlands) by an experienced reader (O.H.T.-S.). Ventricular analysis calculations, including EF, stroke volume, end-diastolic volume, LV mass/volume ratio, and end-systolic fiber stress (ESFS) were performed. ESFS was calculated as follows: ESFS = 1.35 × P × bm × 2 h (expressed in grams per square centimeter), where P is end-systolic pressure, D is end-systolic LV internal diameter (in centimeters), h is end-systolic posterior wall thickness, and bm is the midwall minor semiaxis, expressed as h /ln(0.5 D + h ) − ln(0.5 D ). To assess interobserver variability of volumetric analyses, a second expert (K.N.H.) performed the same analysis on subsets of 10 subjects. Tagged images were analyzed with the harmonic phase (HARP; Diagnosoft, Palo Alto, CA) technique for calculation of LV midwall peak circumferential and longitudinal strain magnitudes. Regional changes in ε cc were performed at the midventricular level only, given the limited reproducibility of the basal and apical slices. Midwall strain data were exported to a spreadsheet program for offline analysis. To assess interobserver variability of HARP strain analyses, a second expert reader performed the same analysis in a subset of 10 randomly selected subjects in a blinded fashion (O.H.T.-S., K.N.H.). HARP-based strain values were considered abnormal if >1.5 SDs below the mean (normal ε cc , −19.5 ± 2.1; normal ε ll , −17.3 ± 1.4) on the basis of values obtained in 18 age- and gender-matched normal control subjects.
Statistical Analysis
Subject values were combined in a single study database. Group differences were compared using t tests for continuously distributed variables, such as echocardiographic parameters of systolic function and total cumulative anthracycline dose, or χ 2 and Fisher exact tests for categorical variables such as gender and previous shortening fraction < 29%. The screening performance of EF by each echocardiographic modality (area-length, 3DE, and Simpson’s) and all echocardiographic parameters of systolic function were compared with EF < 55% by CMR. Multiple logistic regression analysis was used to identify the most important echocardiographic predictor of CMR-derived EF < 55%. Receiver operating characteristic (ROC) curve analysis was used to determine the echocardiographic parameters with the greatest areas under the curve (AUCs) to detect EF < 55% and significant reductions in peak midwall circumferential strain (ε cc < −16.3) and longitudinal strain (ε ll < −15.2) magnitude by CMR. The best cutoff values were defined as the points with the highest sums of sensitivity and specificity. All tests were two tailed, and P values < .05 were considered to indicate statistically significant differences. SPSS software (IBM, Armonk, NY) was used for statistical analysis.
Results
Study Population
Baseline characteristics of study subjects are shown in Table 1 . The mean value for baseline LVEF identified retrospectively through our registry for the study cohort was 68% (range, 45%–79%) by TTE. Only one subject presented with an EF < 55% at baseline. The most frequent cancer diagnoses in these subjects were acute myelogenous leukemia (18%), non-Hodgkin’s lymphoma (15%), and acute lymphoblastic leukemia (13%).
Variable | Value |
---|---|
Years since chemotherapy | 9 (2.4–26.9) |
Mean age at diagnosis (y) | 10 ± 5.2 |
Median age at visit (y) | 21 (10–42) |
Male/female | 38/19 |
Total cumulative dose anthracyclines (mg/m 2 ) | 316 (200–600) |
Height (cm) | 168.4 ± 11.7 |
Weight (kg) | 71.9 ± 16.8 |
BSA (m 2 ) | 1.8 ± 0.25 |
BMI (kg/m 2 ) | 25 (19–45) |
Heart rate (beats/min) | 68.2 ± 13.0 |
Systolic blood pressure (mm Hg) | 118 (87–148) |
Diastolic blood pressure (mm Hg) | 67 (46–87) |
Cancer diagnosis | |
Leukemia | 22 (38%) |
Solid tumor | 18 (32%) |
Lymphoma | 17 (30%) |
Volumetric Analysis and Assessment of Global Systolic Function
CMR
Twenty-four subjects (43%) had EFs < 55% by CMR ( Table 2 ). Of those, 22 subjects had EFs between 50% and 54%, and two had EFs of 49%. Seventeen of the 38 male subjects (45%) had EFs < 55%, compared with seven of the 19 female subjects (37%). Although a mass/volume ratio Z score < 2 was present in only one subject (5%), ESFS was elevated in 54 subjects (95%). ESFS was inversely correlated with mass/volume ratio ( r = −0.41, P = .001) and positively associated with end-systolic volume index (ESVI) ( r = 0.32, P = .01). Global right ventricular systolic dysfunction was present in three male subjects (8%). Two of these subjects also had right ventricular end-diastolic volume Z scores > 2.
CMR parameter | Mean ± SD | P | Z score | Abnormal ∗ |
---|---|---|---|---|
LVEF (%) | ||||
Male ( n = 38) | 55.8 ± 3.9 | .34 | −1.80 ± 0.85 | 17 (45%) |
Female ( n = 19) | 56.9 ± 4.7 | −148 ± 0.97 | 7 (37%) | |
LVEDV (mL/m 2 ) | ||||
Male ( n = 38) | 85.5 ± 15.3 | <.0001 | 0.21 ± 1.1 | 2 (5%) |
Female ( n = 19) | 70.6 ± 8.6 | −0.66 ± 0.75 | 0 (0%) | |
LVESV (mL/m 2 ) | ||||
Male ( n = 38) | 38.0 ± 8.5 | .018 | ||
Female ( n = 19) | 32.0 ± 9.3 | |||
LVSV (mL) | ||||
Male ( n = 38) | 89.6 ± 17.7 | <.0001 | ||
Female ( n = 19) | 67.4 ± 9.4 | |||
LV mass (g/m 2 ) | ||||
Male ( n = 38) | 65.2 ± 10.9 | <.0001 | 0.19 ± 1.4 | 1 (3%) |
Female ( n = 19) | 49.5 ± 5.4 | −0.36 ± 0.79 | 0 (0%) | |
LV mass/volume ratio (g/mL) | ||||
Male ( n = 38) | 0.77 ± 0.13 | .05 | −0.10 ± 1.4 | 0 (0%) |
Female ( n = 19) | 0.71 ± 0.07 | 0.26 ± 0.87 | 1 (5%) | |
RVEDV (mL/m 2 ) | ||||
Male ( n = 38) | 84.9 ± 15.5 | <.0001 | −0.03 ± 1.2 | 2 (5%) |
Female ( n = 19) | 69.2 ± 7.9 | −0.47 ± 0.55 | 0 (0%) | |
RVESV (mL/m 2 ) | ||||
Male ( n = 38) | 37.9 ± 9.9 | <.0001 | ||
Female ( n = 19) | 29.5 ± 5.2 | |||
RVSV (mL) | ||||
Male ( n = 38) | 88.9 ± 17.0 | <.0001 | ||
Female ( n = 19) | 66.2 ± 9.2 | |||
RVEF (%) | ||||
Male ( n = 38) | 55.9 ± 4.6 | .30 | 0.05 ± 1.4 | 3 (8%) |
Female ( n = 19) | 57.3 ± 4.4 | −0.56 ± 0.87 | 0 (0%) | |
ESFS (g/cm 2 ) | ||||
Male ( n = 38) | 126.2 ± 16.8 | .27 | 4.6 ± 1.5 | 36 (95%) |
Female ( n = 19) | 132.0 ± 22.2 | 5.2 ± 1.9 | 18 (95%) |
∗ Abnormal defined as EF < 55%, Z score < −2 for mass, mass/volume ratio, or Z score > 2 for EDV and ESFS.
Echocardiographic Parameters of Systolic Function
Echocardiographic parameters of global systolic function are shown in Table 3 and gender differences in Tables 4-6 . Three-dimensional echocardiographic measurement of EF had a sensitivity of 68% in identifying subjects with CMR-derived EFs < 55%, compared with 50% and 46% for quantitative analysis of 2D images by the area-length and Simpson’s biplane methods. When compared with CMR, 3DE would have failed to identify nine subjects with CMR-derived EFs < 55% compared with 12 subjects by the area-length method and 13 subjects by the Simpson’s biplane method. Bland-Altman analysis of EF by CMR compared with 2D echocardiographic (area-length and Simpson’s biplane) and 3D echocardiographic measurement of EF is shown in Figure 2 . ROC analysis of LV function by TTE identified subjects with CMR-derived EFs < 55% with AUCs of 0.77 (0.64–0.88; P = .001) for 2D area-length, 0.64 (0.48–0.80; P = .09) for 3DE, and 0.71 (0.56–0.86; P = .01) for Simpson’s biplane method.
Echocardiographic parameters | Mean ± SD | Z score | Abnormal ‡ |
---|---|---|---|
SF (%) | 32.0 ± 3.9 | −0.16 ± 4.9 | 10 (17.5%) |
LV ET (sec) | 0.28 ± 0.03 | ||
LV MPI ∗ | 0.53 ± 0.10 | 55 (96.5%) | |
LVEF § (%) | 56.9 ± 4.8 | −1.1 ± 1.0 | 20 (35.1%) |
LVEF || (%) | 54.4 ± 4.1 | 27 (47.3%) | |
LVEF ¶ (%) | 57.4 ± 4.6 | 17 (29.8%) | |
ESWS (g/cm 3 ) | 64.3 ± 16.9 | 1.6 ± 0.49 | 21 (36.8%) |
ESFS (g/cm 2 ) | 117.3 ± 23.9 | 1.7 ± 0.46 | 16 (28.1%) |
VCFc (circ/sec) | 0.99 ± 0.43 | −0.18 ± 1.8 | 9 (15.8%) |
Stress-shortening index † | 0.49 ± 1.3 | 2 (3.5%) | |
Stress-velocity index ‡ | 0.61 ± 1.3 | 0 (0%) | |
LV mass | 96.8 ± 28.2 | −1.6 ± 2.1 | 28 (49.1%) |
LV mass/volume ratio | 0.77 ± 0.17 | −0.50 ± 1.1 | 4 (7.0%) |
∗ Normal LV MPI value = 0.35 ± 0.03.
† Stress-shortening index and stress-velocity index are Z scores.
‡ Abnormal defined as SF < 29%, EF < 55%, or Z score < −2 or > 2.
|| Three-dimensional echocardiography.
Parameter | Mean ± SD | P | Abnormal ∗ |
---|---|---|---|
LVEF (%) | |||
Male ( n = 34) | 54.1 ± 4.4 | .48 | 20 (59%) |
Female ( n = 15) | 55.0 ± 3.6 | 7 (47%) | |
LVEDV (mL/m 2 ) | |||
Male ( n = 34) | 53.1 ± 11.8 | .03 | |
Female ( n = 15) | 45.3 ± 10.2 | ||
LVESV (mL/m 2 ) | |||
Male ( n = 34) | 24.4 ± 6.2 | .04 | |
Female ( n = 15) | 20.4 ± 5.7 | ||
LVSV (mL) | |||
Male ( n = 34) | 54.3 ± 11.6 | <.0001 | |
Female ( n = 15) | 40.8 ± 7.1 |
Parameter | Mean ± SD | P | Abnormal ∗ |
---|---|---|---|
LVEF (%) | |||
Male ( n = 37) | 56.7 ± 4.6 | .08 | 15 (41%) |
Female ( n = 16) | 59.1 ± 4.1 | 2 (13%) | |
LVEDV (mL/m 2 ) | |||
Male ( n = 37) | 52.0 ± 12.6 | .002 | |
Female ( n = 16) | 41.0 ± 6.8 | ||
LVESV (mL/m 2 ) | |||
Male ( n = 37) | 22.5 ± 5.7 | <.0001 | |
Female ( n = 16) | 16.8 ± 3.3 | ||
LVSV (mL) | |||
Male ( n = 37) | 55.4 ± 13.3 | <.0001 | |
Female ( n = 16) | 40.5 ± 8.8 |
Parameter | Mean ± SD | P | Z score | Abnormal ∗ |
---|---|---|---|---|
LVEF (%) | ||||
Male ( n = 38) | 56.1 ± 5.2 | .07 | −1.23 ± 1.2 | 18 (47%) |
Female ( n = 19) | 58.5 ± 3.6 | −0.86 ± 0.7 | 2 (11%) | |
LVEDV (mL/m 2 ) | ||||
Male ( n = 38) | 73.6 ± 16.2 | .004 | −0.70 ± 1.4 | 1 (3%) |
Female ( n = 19) | 62.7 ± 11.1 | −1.37 ± 1.0 | 0 (0%) | |
LVESV (mL/m 2 ) | ||||
Male ( n = 38) | 32.3 ± 8.1 | <.0001 | ||
Female ( n = 19) | 25.8 ± 3.9 | |||
LVSV (mL) | ||||
Male ( n = 38) | 77.2 ± 18.9 | .006 | ||
Female ( n = 19) | 62.7 ± 16.5 | |||
LV mass (g/m 2 ) | ||||
Male ( n = 38) | 56.1 ± 14.5 | .003 | −1.3 ± 2.5 | 15 (39%) |
Female ( n = 19) | 47.1 ± 7.3 | −2.2 ± 0.8 | 13 (68%) | |
LV mass/volume ratio (g/mL) | ||||
Male ( n = 38) | 0.77 ± 0.17 | .83 | −0.54 ± 1.1 | 2 (5%) |
Female ( n = 19) | 0.77 ± 0.16 | −0.45 ± 1.1 | 2 (11%) | |
ESFS (g/cm 2 ) | ||||
Male ( n = 38) | 121.5 ± 25.9 | .06 | 1.61 ± 0.5 | 14 (37%) |
Female ( n = 19) | 108.9 ± 16.9 | 1.89 ± 0.3 | 2 (11%) |
∗ Abnormal defined as SF < 29%, EF < 55%, Z score < −2 for mass, mass/volume ratio, or Z score > 2 for EDV and ESFS.

End-Systolic Volume Analysis
Subjects in the two highest ESVI quartiles were more likely to have CMR-derived EFs < 55% ( Figure 3 ). Subjects with ESVI values > 29 mL/m 2 had 8 times the odds of having an EF < 55% by CMR (95% CI, 2.3–28.8; P < .001). Subjects in the highest ESVI quartile had lower CMR-derived EFs, higher end-diastolic volume index values, and higher left atrial volume index values. Mildly abnormal ESVI by Simpson’s biplane (32–38 mL/m 2 for male subjects and 25–32 mL/m 2 for female subjects) correctly identified four of seven female subjects and 12 of 17 male subjects with EFs < 55% by CMR.
Figures 4 and 5 demonstrate the correlations and Bland-Altman analysis of volumes by CMR compared with 2D echocardiography (area-length and Simpson’s biplane) and 3DE. Overall, 2D and 3D echocardiographic volumes were higher among male subjects, with no significant changes noted in parameters of global systolic function. Bland-Altman analysis demonstrated a mean difference of 5.78 ± 6.34 for 2D echocardiographic area-length compared with 14.95 ± 5.15 by Simpson’s biplane and 12.69 ± 6.91 for 3DE. ROC analysis of ESVI by the 2D area-length method identified subjects with CMR-derived EFs < 55% with an AUC of 0.91 (0.83–0.99; P < .001), compared with an AUC of 0.89 (0.79–0.98; P < .001) for Simpson’s biplane and 0.79 (0.66–0.93; P = .002) for 3DE.
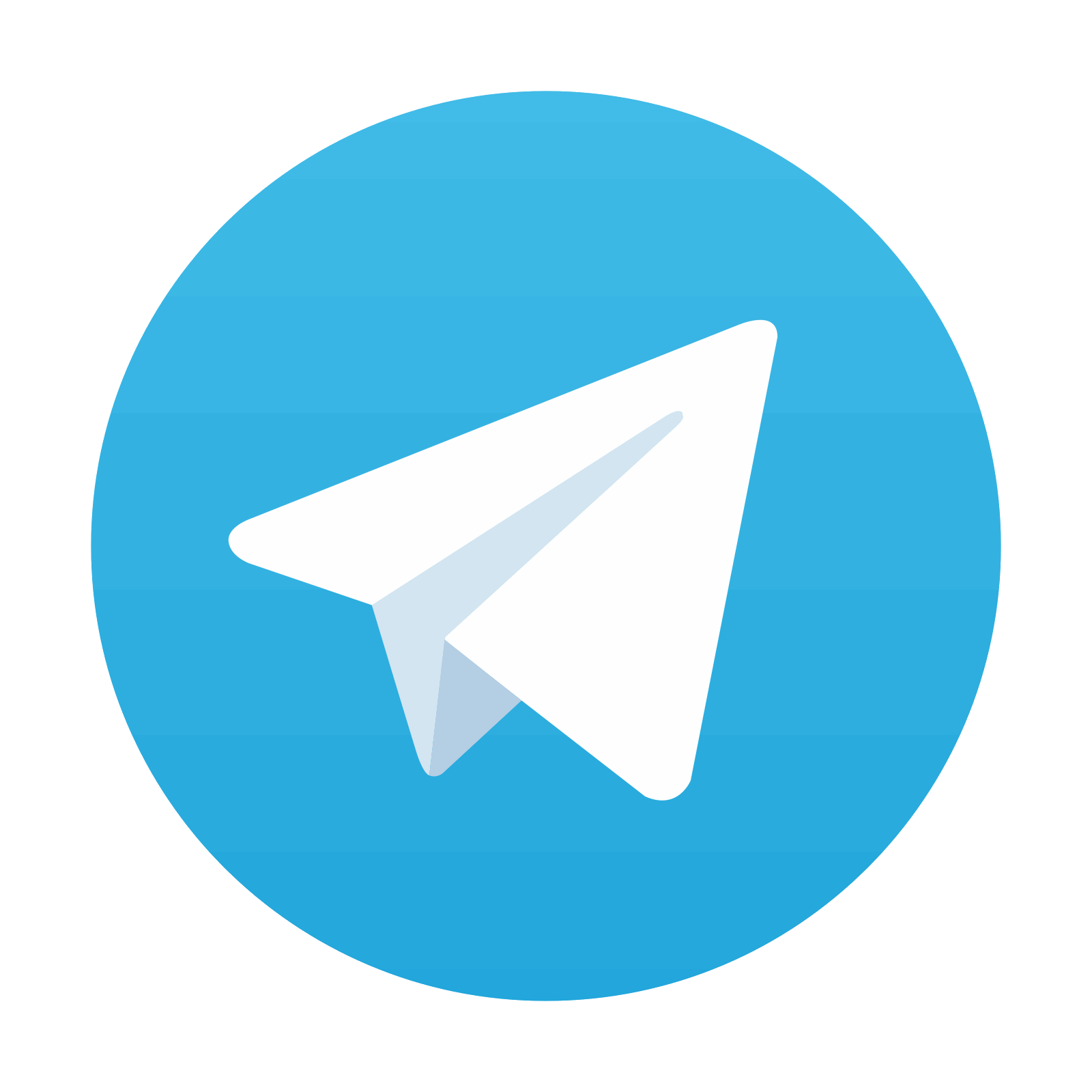
Stay updated, free articles. Join our Telegram channel

Full access? Get Clinical Tree
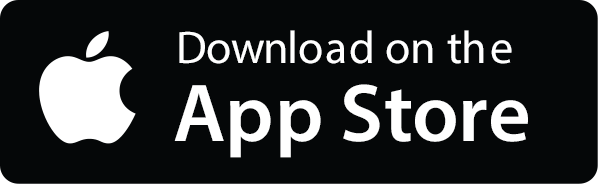
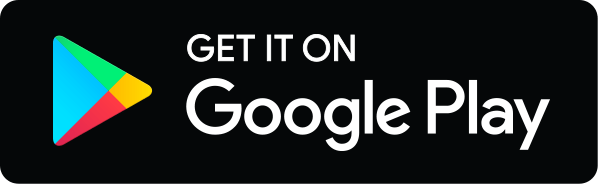
