Coronary computed tomography angiography (CTA) has important prognostic value. Additionally, quantitative CTA (QCT) provides a more detailed accurate assessment of coronary artery disease (CAD) on CTA. Potentially, a risk score incorporating all quantitative stenosis parameters allows accurate risk stratification. Therefore, the purpose of this study was to determine if an automatic quantitative assessment of CAD using QCT combined into a CTA risk score allows risk stratification of patients. In 300 patients, QCT was performed to automatically detect and quantify all lesions in the coronary tree. Using QCT, a novel CTA risk score was calculated based on plaque extent, severity, composition, and location on a segment basis. During follow-up, the composite end point of all-cause mortality, revascularization, and nonfatal infarction was recorded. In total, 10% of patients experienced an event during a median follow-up of 2.14 years. The CTA risk score was significantly higher in patients with an event (12.5 [interquartile range 8.6 to 16.4] vs 1.7 [interquartile range 0 to 8.4], p <0.001). In 127 patients with obstructive CAD (≥50% stenosis), 27 events were recorded, all in patients with a high CTA risk score. In conclusion, the present study demonstrated that a fully automatic QCT analysis of CAD is feasible and can be applied for risk stratification of patients with suspected CAD. Furthermore, a novel CTA risk score incorporating location, severity, and composition of coronary lesion was developed. This score may improve risk stratification but needs to be confirmed in larger studies.
The aim of the present study was to evaluate patients with suspected coronary artery disease (CAD) undergoing computed tomography angiography (CTA) and (1) perform a fully automatic quantitative assessment of coronary CTA data sets to assess the location, severity and composition of CAD and (2) to incorporate all these variables into 1 risk score. Further aims were (3) to assess the value of this integrated score for risk stratification and (4) to compare the risk classification according to this new score compared with existing risk scores.
Methods
The population consisted of 300 patients referred for the evaluation of typical or atypical chest pain or dyspnea. Patients with previous percutaneous coronary intervention or coronary artery bypass graft were excluded. Clinical data were prospectively entered into the departmental Cardiology Information System (EPD-Vision; Leiden University Medical Center, Leiden, the Netherlands) and retrospectively analyzed. The Institutional Review Board of the Leiden University Medical Center approved this retrospective evaluation of clinically collected data and waived the need for written informed consent.
Patients were scanned either with a 64-slice computed tomography scanner (Aquilion 64; Toshiba Medical Systems, Otowara, Japan) or a 320-row volumetric scanner (Aquilion ONE; Toshiba Medical Systems). Coronary CTA was performed according to standard clinical practice as previously described. Only patients with clinical diagnostic image quality of the coronary CTA were included.
Quantitative CTA (QCT) was performed in 5 automatic steps, as depicted in Figure 1 .
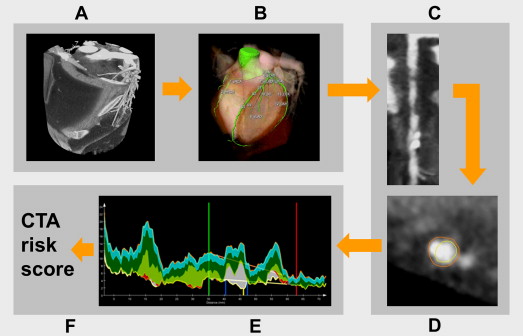
(1) The coronary tree was automatically extracted from the coronary CTA data set. Using a tree labeling algorithm, the segments of the coronary tree were automatically labeled according the American Heart Association 17-segment model. (2) Curved multiplanar reformations of each coronary vessel and side branch were created. (3) The lumen and vessel wall were automatically segmented using a previously validated software tool (QAngio CT Research Edition, version 1.3.6; Medis Medical Imaging Systems, Leiden, the Netherlands). (4) Coronary plaque constitution was assessed using a dedicated tissue characterization algorithm as previously described. This tissue characterization algorithm allows the differentiation into 4 different plaque types. Currently, it is unclear how this should be translated to the commonly used classification of noncalcified, partially calcified, and calcified plaque. For this analysis, the percentage ratio between dense calcium (DC) and necrotic core (NC) [DC/(NC + DC) × 100] was used to differentiate among partially calcified, noncalcified, and calcified plaques. Lesions with a ratio <10% were considered noncalcified plaque as well as lesions without NC or DC, and lesions with a ratio >75% were classified as calcified plaque. Coronary plaques with ratios ≥10% and ≤75% were classified as partially calcified plaque. (5) Coronary lesions were automatically detected. Within each segment, a regression analysis is performed on the lumen area graph to define a lumen reference line. A lesion is defined on the region surrounding the minimal lumen area where the lumen area is smaller than this reference. If needed, this lesion is enlarged to include calcified spots located near the minimal lumen area (within 50 mm and >1 mm 3 ). Lesions with negative stenosis degree and steep reference slopes (<−0.35 mm 2 /mm) were removed as well as short lesions (<1.5 mm), lesions in segments with small vessel and lumen areas (<8 mm 2 and <1.5 mm 2 , respectively), and distally located lesions (>150 mm from ostium).
The automatic lesion detection was confirmed by an experienced observer. The software allows the observer to override the automatic lesion detection. If needed, small adjustments were made.
A novel comprehensive risk score was created to combine the information on location, extent, severity, and composition of each coronary lesion. As described in Figure 2 , the score consists of 3 components for each segment: a segment location weight factor, a stenosis severity weight factor, and a plaque weight factor. (1) The location of each lesion is represented by a segment weight factor based on the Leaman score. A different set of weight factors is applied to left- or right-dominant coronary artery systems. (2) Stenosis severity was described by the stenosis severity weight factor. A previous meta-analysis reported a hazard ratio of 1.35 (95% confidence interval 1.09 to 1.67) for each significant stenosis in each segment of the coronary tree. Therefore, 1.4 was chosen as the weight factor for a significant stenosis (≥50% area stenosis). (3) In a study performed by Gaemperli et al, stratifying the diseased segments according to plaque composition, the investigators reported a hazard ratio of 1.21 (95% confidence interval 1.11 to 1.32) for segments with calcified plaques, 1.57 (95% confidence interval 1.38 to 1.79) for segments with partially calcified plaques, and 1.71 (95% confidence interval 1.14 to 2.56) for segments with noncalcified plaques. This was translated in the score by a weight factor of 1.2 for calcified plaque, 1.6 for partially calcified plaque, and 1.7 for noncalcified plaque.
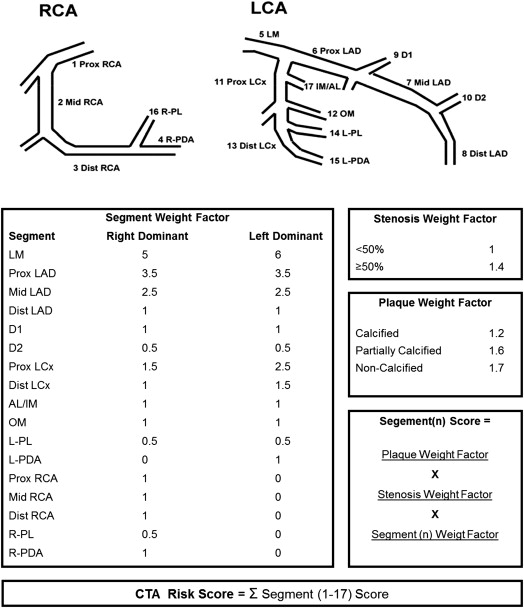
The CTA risk score is automatically calculated using QCT. When coronary plaque is absent (<30% area stenosis) the score is 0. When a stenosis is present, a score is given according to the location of the lesion in the coronary artery tree; this score is multiplied by the stenosis weight factor and multiplied by the plaque weight factor. The final score is calculated by summation of the individual segment scores (range 0 to 55; Figure 2 ).
In addition, the modified Duke prognostic CAD index was applied to the QCT results. The score consists of 6 categories; 1: <50% stenosis, 2: ≥2 stenoses 30% to 49% (including 1 artery with proximal disease or 1 vessel with 50% to 69% stenosis, 3: 2 stenoses 50% to 69% or 1 vessel with ≥70% stenosis, 4: 3 stenoses 50% to 69% or 2 vessels with ≥70% stenosis or proximal left anterior descending stenosis ≥70%, 5: 3 vessels ≥70% stenosis or 2 vessels ≥70% stenosis with proximal left anterior descending, and 6: left main stenosis ≥50%. Subsequently, the distribution of the novel CTA risk score within the Duke CAD categories was assessed.
Patient follow-up data were gathered using clinical visits or standardized telephone interviews. A composite end point was constructed using all-cause mortality, revascularization after 30 days after CTA image acquisition, and nonfatal myocardial infarction. This 30-day interval was used to exclude coronary CTA–driven events (referral for angiography mainly based on coronary CTA findings). Nonfatal myocardial infarction was defined based on criteria of typical chest pain, elevated cardiac enzyme levels, and typical electrocardiographic changes.
Continuous data are presented as mean ± SD if normally distributed or as median (interquartile range [IQR]) if non-normally distributed. Categorical data are presented as absolute number and percentage. First, the QCT parameters were compared between both patients groups (with vs without events). Second, both the novel CTA risk score and the modified Duke prognostic CAD index were compared between both groups. Third, the ability of the CTA risk score for risk stratification of patients was assessed. For this purpose, the CTA risk score was stratified into a low- and high-risk category based on receiver operating characteristic curve analysis, ensuring the highest negative predictive value. First, the distribution of the risk score in patients with and without obstructive CAD (≥50% area stenosis in QCT) was assessed and correlated to the occurrence of an event. In a similar fashion, the distribution within the Duke CAD categories was assessed. For this purpose, the Duke CAD categories were divided into 3 groups: mild CAD, defined as Duke CAD category 1; moderate CAD, defined as Duke CAD category 2 to 3; and severe CAD, defined by the 3 most severe categories. Fourth, to evaluate the independent predictive value of the CTA risk score, univariate and multivariate Cox regression analyses were performed. All baseline or univariate significant clinical variables were entered into the multivariate model. All statistical tests were 2-sided and a p value of <0.05 was considered statistically significant. All statistical analyses were performed with SPSS software (version 20.0; SPSS Inc., Chicago, Illinois).
Results
The patient population consisted of 300 patients referred for the evaluation of chest pain during the period January 2008 to May 2010. Baseline characteristics are summarized in Table 1 . The median follow-up duration was 2.14 years (IQR 1.07 to 3.48); 28 patients (9%) were lost to follow-up. During the follow-up period the composite end point occurred in 29 patients (event rate 10%); 25 patients (8%) underwent revascularization (23 percutaneous coronary intervention and 2 coronary artery bypass graft) after 30 days of CTA acquisition. Death occurred in 4 patients (1%). In patients with an event, mean age was higher and diabetes and hypertension were more often prevalent.
Variable | Total (300) | Event | p-Value | |
---|---|---|---|---|
No (N = 271) | Yes (N = 29) | |||
Age (years) | 55 ± 11.5 | 54 ± 11.6 | 60 ± 8.5 | <0.001 |
Men | 180 (60%) | 161 (59%) | 19 (66%) | 0.526 |
Diabetes Mellitus | 90 (30%) | 76 (28%) | 14 (48%) | 0.024 |
Hypertension † | 111 (37%) | 95 (35%) | 16 (55%) | 0.034 |
Hypercholesterolemia ‡ | 100 (33%) | 88 (32%) | 12 (41%) | 0.334 |
Smoker | 52 (17%) | 47 (17%) | 5 (17%) | 0.988 |
Obesity ∗ | 56 (19%) | 49 (18%) | 7 (24%) | 0.432 |
Calcium score | 146 ± 420 1 (0–86) | 118 ± 388 1 (0–54) | 407 ± 596 148 (30–514) | <0.001 |
∗ Defined as a body mass index of ≥30 Kg/m 2 .
† Defined as systolic blood pressure ≥140 mm Hg and/or diastolic blood pressure ≥90 mm Hg or the use of antihypertensive medication.
‡ Defined as serum total cholesterol ≥230 mg/dL or serum triglycerides ≥200 mg/dL or treatment with lipid lowering medication.
The results of the QCT lesion analysis on a patient basis are depicted in Table 2 . In patients with an event, significant obstructive lesions were more frequently observed. Furthermore, in patients with an event the mean number of partially calcified or calcified lesions was higher compared with patients without events. In the overall population, the median CTA risk score was 3.0 (IQR 0 to 9.8). The median CTA risk score was significantly higher in patients with an event compared with event-free patients (12.5 [IQR 8.6 to 16.4] vs 1.7 [IQR 0 to 8.4], p <0.001). Accordingly, in patients with a CTA risk score of 0, the event rate was <1% (1 of 130). Based on receiver operating characteristic curve analysis, a CTA risk score of 7 was defined as a cut-off value between low and high CTA risk score to ensure the highest negative predictive value. Figure 3 provides a patient example of the QCT analysis. The distribution of patients with a high and low CTA risk score between patients with and without obstructive CAD is depicted in Figure 4 . Of interest, in the 112 patients with obstructive CAD, all events occurred in patients with a high CTA risk score. The results of the modified Duke prognostic CAD index calculation based on the QCT results are summarized in Figure 5 . Most patients were categorized in Duke CAD category 1. Indeed, all events in patients within Duke CAD category 2 or 3 occurred in patients with a high CTA risk score. Only 1 event occurred in a patient with a low CTA risk score; this patient was classified in Duke CAD category 1. In the univariate Cox regression analysis ( Table 3 ), age and CTA risk score were significantly associated with the occurrence of an event. In the multivariate analysis, adjusted for significant baseline characteristics, the CTA risk score was independently associated with events.
Variable | Event | p-Value | |
---|---|---|---|
No (N = 271) | Yes (N = 29) | ||
No. of plaques ≥30% | 1.54 ± 2.14 | 3.83 ± 2.04 | <0.001 |
No. of plaques 30–50% | 0.82 ± 1.17 | 1.45 ± 1.12 | <0.001 |
No. of obstructive lesions ≥50% | 0.51 ± 0.87 | 1.83 ± 0.97 | <0.001 |
No. of severe lesions ≥70% | 0.17 ± 0.92 | 0.69 ± 1.07 | <0.001 |
No. of calcified lesions | 0.95 ± 1.67 | 2.66 ± 2.10 | <0.001 |
No. of partially calcified lesions | 0.31 ± 0.73 | 0.76 ± 0.87 | <0.001 |
No. of non-calcified lesions | 0.29 ± 0.60 | 0.41 ± 0.63 | 0.189 |
No. of narrowed coronary arteries | 0.51 ± 0.87 | 1.83 ± 0.97 | <0.001 |
Left main lesion | 6 (2%) | 4 (13%) | 0.001 |
Right coronary artery lesion | 45 (16%) | 11 (37%) | 0.005 |
Left anterior descending artery lesion | 63 (23%) | 24 (82%) | <0.001 |
Left circumflex artery lesion | 25 (9%) | 13 (44%) | <0.001 |
Lesions in proximal segments | 0.34 ± 0.68 | 1.07 ± 1.0 | <0.001 |
Lesions only in distal segments | 0.38 ± 0.89 | 1.31 ± 1.17 | <0.001 |
Percentage of proximal lesions | 52 ± 37 | 48 ± 37 | 0.961 |
