Background
In biologic systems, the arrest of circulating cells is mediated by adhesion molecules projecting their active binding domain above the cell surface to enhance bond formation and tether strength. Similarly, molecular spacers are used for ligands on particle-based molecular imaging agents. The aim of this study was to evaluate the influence of tether length for targeting ligands on ultrasound molecular imaging agents.
Methods
Microbubbles bearing biotin at the end of variable-length polyethylene glycol spacer arms (MB 2000 and MB 3400 ) were prepared. To assess in vivo attachment efficiency to endothelial counterligands that vary in their distance from the endothelial cell surface, contrast-enhanced ultrasound (CEU) molecular imaging of tumor necrosis factor–α–induced P-selectin (long distance) or intercellular adhesion molecule–2 (short distance) was performed with each agent in murine hind limbs. To assess the influence of the glycocalyx on microbubble attachment, CEU molecular imaging of intercellular adhesion molecule–2 was performed after degradation of the glycocalyx.
Results
CEU molecular imaging targeted to P-selectin showed signal enhancement above control agent for MB 2000 and MB 3400 , the degree of which was significantly higher for MB 3400 compared with MB 2000 . CEU molecular imaging targeted to intercellular adhesion molecule–2 showed low overall signal for all agents and signal enhancement above control for MB 3400 only. Glycocalyx degradation increased signal for MB 3400 and MB 2000 .
Conclusions
Microbubble targeting to endothelial ligands is influenced by (1) the tether length of the ligand, (2) the degree to which the endothelial target is projected from the cell surface, and (3) the status of the glycocalyx. These considerations are important for designing targeted imaging probes and understanding potential obstacles to molecular imaging.
Contrast-enhanced ultrasound (CEU) molecular imaging has been developed in recent years for the noninvasive assessment of pathophysiologic changes that are present on a molecular level in atherosclerosis, myocardial ischemia, angiogenesis, thrombus formation, and heart transplant rejection. CEU molecular imaging relies on the selective retention of targeted microbubbles within diseased tissue. Microbubble contrast agents are gas-filled, lipid-shelled or albumin-shelled structures that range from 1 to 4 μm in diameter and are confined to the vascular compartment, thus restricting the potential targets to disease markers present on the endothelial cell surface. Targeting of lipid shelled microbubbles is achieved by conjugation of antibodies or other specific ligands to the microbubble shell surface via functionalized lipids. Targeted retention of microbubbles in a vessel under any given shear condition depends on a number of factors, including (1) ligand density on the microbubble surface, (2) ligand-specific bond kinetics, and (3) site density of the molecular target. Similar to the case with leukocyte and platelet adhesion molecules that have their binding domains projected away from the cell surface to enhance bond formation, the active ligand on targeted microbubbles is often placed at the end of polyethylene glycol (PEG) multimers that project the ligand away from the shell surface and beyond any PEG brush that has been added to the shell surface to stabilize microbubbles.
The aim of this study was to assess whether the length of the functionalized PEG molecules used to conjugate antibodies to the microbubble shell surface influences targeting efficiency. The importance of this issue is underscored by the desire to target not only long adhesion molecules that project their binding moiety far away from the endothelial cell surface and beyond the glycocalyx but also smaller molecules that reside closer to the endothelial cell surface. We therefore compared the targeting efficiency of microbubbles with different-length functionalized PEG molecules to adhesion molecules with a long-distance (P-selectin) and short-distance (intercellular adhesion molecule [ICAM]–2) projection from the endothelial surface, with and without modification of the glycocalyx.
Methods
Microbubble Preparation
Lipid-shelled decafluorobutane microbubbles were prepared by sonication of a gas-saturated aqueous suspension of distearoylphosphatidylcholine (2 mg/mL) and polyoxyethylene-40-stearate (1 mg/mL). For ligand conjugation, functionalized PEG-lipid conjugates containing biotin at the PEG N-terminus were added to the aqueous suspension at concentrations corresponding to equal molar ratios of 50:10:1 (distearoylphosphatidylcholine/polyoxyethylene-40-stearate/functionalized PEG). The PEG length was varied by using 1,2-distearoyl-sn-glycero-phosphoethanolamine-N-[biotinyl(PEG)-2000] (Avanti Polar Lipids, Alabaster, AL), 1,2-distearoyl-sn-glycero-phosphoethanolamine-N-[biotinyl(PEG)-3400] (Creative PEG Works, Winston-Salem, NC), or 1,2-distearoyl-sn-glycero-phosphoethanolamine-N-[biotinyl(PEG)-10000] (Nanocs Inc., Boston, MA) Thus, microbubbles with different-sized PEG-biotin moieties (MB PEG2000 , MB PEG3400 , and MB PEG10000 ) were prepared with PEG multimers of 45, 80, and 225, respectively.
Monoclonal antibodies were conjugated to the microbubble surface as previously described using a streptavidin-biotin link. Rat antimouse monoclonal immunoglobulin G1 against either P-selectin (Rb40.34) or ICAM-2 (mLC2/4) were conjugated to the microbubble surface to produce P-selectin-targeted (MB PEG2000-PSel , MB PEG3400-PSel , and MB PEG10000-PSel ) or ICAM-2-targeted (MB PEG2000-ICAM , MB PEG3400-ICAM , and MB PEG10000-ICAM ) microbubbles, each with different-length PEG-biotin moieties. Isotype control antibodies (R3-34; BD Bioscience, San Jose, CA) were conjugated to the surfaces of microbubbles with randomly selected PEG spacer–length moieties to produce control microbubbles (MB Ctr ). Microbubble concentration, size, and surface area were measured by electrozone sensing (Multisizer III; Beckman Coulter, Brea, CA).
Biotin and Antibody Density on the Microbubble Surface
To quantify the number of PEG spacer moieties and antibodies on the microbubble surface by quantitative fluorescence, aliquots of 1 × 10 8 for MB PEG2000 , MB PEG3400 , and MB PEG10000 were washed and incubated with 30 μg fluorescein isothiocyanate (FITC)–labeled streptavidin (Leinco Technologies, St. Louis, MO). Excess FITC-streptavidin was removed with three cycles of flotation centrifugation. Microbubble concentration and mean surface area were measured, and the microbubbles were destroyed by prolonged application of pressure at 100 mm Hg. The concentration of fluorescently labeled streptavidin was measured using a Gemini XPS fluorescence microplate reader (Molecular Devices, Sunnyvale, CA) and compared with a concentration-intensity reference standard to derive the density per surface area. For determination of the number of antibodies bound per square micrometer of microbubble surface, a known concentration of biotinylated immunoglobulin G was labeled with FITC. Labeled antibodies were conjugated to the different microbubble preparations, and quantified as described for FITC-streptavidin. All experiments were performed in triplicate.
Animal Preparation
All experiments were performed in accordance with Swiss federal legislation and were approved by the local animal care and use committee. Male wild-type C57BL/6 mice aged 8 to 10 weeks were anaesthetized with 1.5% inhaled isoflurane. Body temperature was maintained at 37°C with a heating pad. A jugular vein was cannulated for administration of microbubbles.
CEU Imaging
Ultrasound imaging (Sequoia Acuson C512; Siemens Medical Systems USA Inc., Mountain View, CA) was performed with a high-frequency linear-array probe (15L8) held in place by a railed gantry system. The adductor muscle of the mouse hind limb was imaged in short axis. Anatomic images of the hind limb were acquired with fundamental imaging at 14 MHz. CEU was performed with power modulation and pulse inversion (Contrast Pulse Sequence) imaging at a centerline frequency of 7 MHz. The gain settings were adjusted just below visible tissue speckle and held constant.
In vivo circulation half-life of microbubble preparations with different length PEG spacer moieties were compared using low–mechanical index ultrasound imaging. Nontargeted MB PEG2000 , MB PEG3400 , and MB PEG10000 (1 × 10 7 each) were injected intravenously as a bolus in random order. Thirty seconds after the bolus injection, single image frames of the proximal hind limb were obtained at a mechanical index of 0.2 at 15-sec intervals for 10 min. It has previously been shown that these imaging parameters do not result in microbubble destruction when imaging the mouse hind limb. On digitized image sequences, acoustic intensity from a region of interest over the hind limb adductor muscle was derived over time after removal of logarithmic signal compression.
For in vivo assessment of attachment efficiency of microbubbles targeted to P-selectin with different-length PEG spacer moieties, a mouse model of murine hind limb inflammation was used ( n = 16). CEU molecular imaging of the hind limb was performed 45 min after intramuscular injection of tumor necrosis factor (TNF)–α (250 ng). MB PEG2000-PSel , MB PEG3400-PSel , MB PEG10000-PSel , and MB Ctr (1 × 10 7 each) were injected intravenously as a bolus in random order. Ultrasound imaging was paused for 8 min after each injection. Imaging was then resumed at a mechanical index of 0.87. The first acquired image frame was used to derive the total amount of microbubbles present in tissue. The microbubbles in the ultrasound beam were then destroyed with several (>10) image frames. Several image frames at a long pulsing interval (10 sec) acquired after microbubble destruction were then acquired to measure signal attributable to freely circulating microbubbles. Data were log-linear converted, and frames representing freely circulating microbubbles were digitally subtracted from the first image frame to derive signal from attached microbubbles alone.
For in vivo assessment of the attachment efficiency of microbubbles targeted to ICAM-2, imaging was performed as described above in murine hind limbs ( n = 16), without induction of inflammation, because ICAM-2 has been shown to be constitutively expressed on endothelial cells, with the level of expression not influenced by cytokines. In an additional 16 mice, molecular imaging was performed 60 min after intravenous injection of 32 IU of Streptomyces hyaluronidase (Sigma-Aldrich, St. Louis, MO), which was performed to reduce microvascular endothelial glycocalyx thickness.
Statistical Analysis
Data were analyzed using GraphPad Prism version 5.0d (GraphPad Software, Inc., La Jolla, CA). Data are expressed as mean ± SEM unless stated otherwise. One-way analysis of variance with Tukey’s post hoc test was used for multiple comparisons of normally distributed variables. Friedman’s repeated-measures analysis of variance with Dunn’s post hoc test was used to compare variables not normally distributed. Two-sided P values < .05 were considered statistically significant.
Results
Microbubble Shell Characteristics
Because microbubble acoustic signal is influenced by size, microbubble diameter was assessed for the different agents according to PEG spacer length. The microbubble agents did not differ in terms of their mean diameters or their size distributions ( Figure 1 ), with 10 ± 6%, 8 ± 4%, and 8 ± 5% of MB PEG2000 , MB PEG3400 , and MB PEG10000 , respectively, having diameters > 5 μm ( P = NS for multiple comparison).
Fluorescent labeling with streptavidin showed similar numbers of biotin molecules on the microbubble surface for MB PEG2000 and MB PEG3400 but a significantly lower number of biotin molecules for MB PEG10000 ( Table 1 ). As a result, surface density for antibody that was coupled via biotin was similar for MB PEG2000 and MB PEG3400 but was more than an order of magnitude lower for MB PEG10000 .
PEG spacer length | PEG-biotin surface density (μm −2 ) | Antibody surface density (μm −2 ) |
---|---|---|
PEG 2000 | 12,463 ± 1,834 | 4,052 ± 1,480 |
PEG 3400 | 14,799 ± 5,567 | 3,703 ± 1,762 |
PEG 10000 | 2,173 ± 2,349 | 176 ± 120 |
In Vivo Circulation Time
Because the duration of recirculation could potentially affect the extent of agent attachment, microbubble circulation life span was assessed in vivo using nondestructive low–mechanical index ultrasound imaging. The time intensity profile and circulation half-life were similar for MB PEG2000 , MB PEG3400 , and MB PEG10000 ( Figure 2 ).
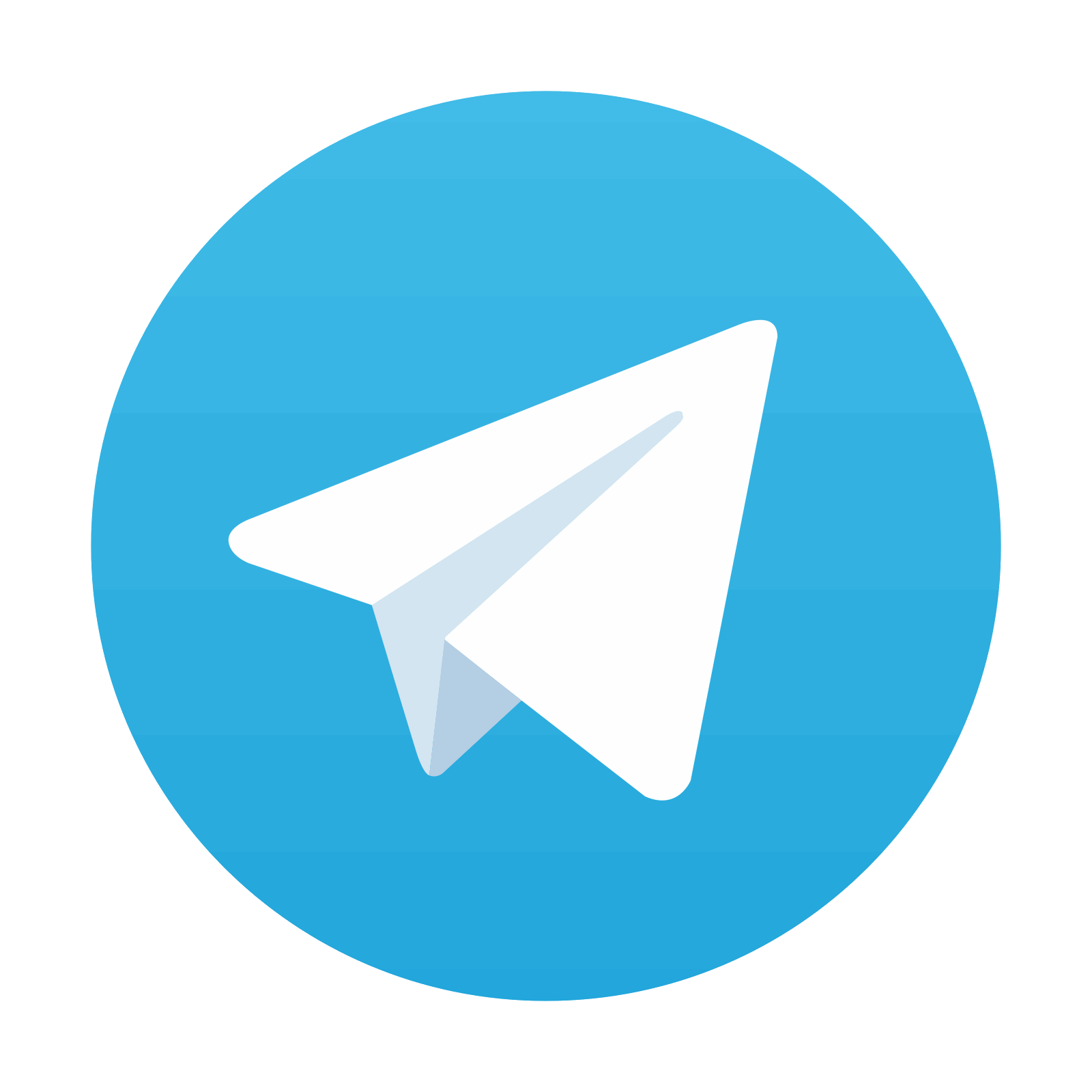
Stay updated, free articles. Join our Telegram channel

Full access? Get Clinical Tree
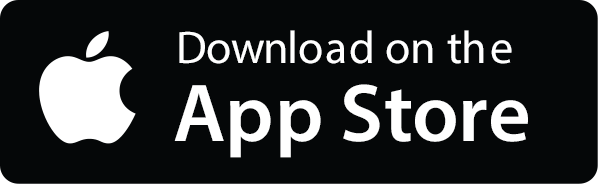
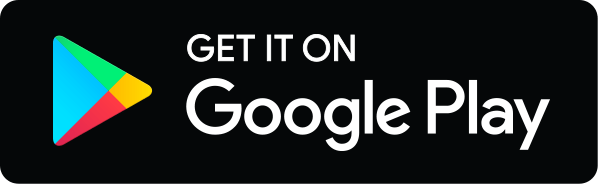
