Abstract
Extracorporeal membrane oxygenation (ECMO) is an established modality of extracorporeal life support (ECLS) for patients with severe, refractory cardiac and/or respiratory failure. ECMO evolved as an extension of the cardiopulmonary bypass technology developed in the 1950s and has now been used in a wide variety of patients. This chapter will examine the principles of ECMO, the indications and contraindications, management during ECMO, and outcomes. Highlighted will be the use of ECMO in pediatric cardiac failure.
Key Words
ECMO, venoarterial ECMO, ECLS, pump, anticoagulation
Extracorporeal membrane oxygenation (ECMO) is an established modality of extracorporeal life support (ECLS) for patients with severe, refractory cardiac and/or respiratory failure. ECMO evolved as an extension of the cardiopulmonary bypass (CPB) technology developed in the 1950s. The first use of ECMO in the intensive care unit was reported in 1972 in an adult with posttraumatic acute respiratory distress syndrome. This was followed by the successful use of extracorporeal oxygenation in 1973 following surgical repair of tetralogy of Fallot and in 1975 in a newborn with respiratory failure secondary to meconium aspiration syndrome. Randomized controlled trials conducted in the 1980s showed superior outcomes in neonates with respiratory failure supported with ECMO compared with those supported with conventional mechanical ventilation. Following these early successes, neonatal respiratory failure represented the main indication for ECMO in the pediatric age for the first 30 years that the technology was available. However, the last 15 years have seen a dramatic increase in the use of ECMO for primary cardiac indications in neonates and older children alike. The Extracorporeal Life Support Organization (ELSO) registry reported a total of 7243 neonatal cardiac ECLS cases and 9479 pediatric cardiac ECLS cases from inception in 1989 to December 31, 2015, of which 2849 neonatal cardiac cases and 3850 pediatric cardiac cases were reported between 2009 and 2015. During the period from 2009 to 2015, yearly numbers of cardiac ECLS cases reported to the ELSO registry ranged from 310 to 470 for neonates, and from 439 to 673 for older infants and children. Health care providers caring for children with cardiac disease should be knowledgeable about indications, management, and complications associated with ECMO because it is both a common and important treatment modality offered to these children.
General Indications and Contraindications
Cardiac indications for ECMO support include perioperative support and support for nonsurgical conditions. Increasingly in recent years ECMO has been used to support children with cardiac arrest failing to respond to conventional cardiopulmonary resuscitation (ECPR) ( Box 39.1 ).
Indications
- 1.
Refractory cardiogenic shock
Acute myocarditis
Cardiomyopathy
Cardiac dysfunction in severe sepsis
- 2.
Postoperative refractory cardiac failure
Failure to wean from cardiopulmonary bypass
Postoperative low cardiac output syndrome
Refractory cardiac arrhythmias
Pulmonary hypertension
- 3.
Cardiac arrest refractory to conventional cardiopulmonary resuscitation
- 4.
Procedural support
- 5.
Bridge to lung or heart transplantation or ventricular assist device
Relative Contraindications
- 1.
End-stage primary disease with poor prognosis
- 2.
Severe neurologic injury or intracranial hemorrhage
- 3.
Uncontrolled visceral bleeding
- 4.
Prematurity (<34 weeks’ gestation)
- 5.
Small size (<2 kg)
- 6.
Family or patient directive limiting ECMO use
ECMO, Extracorporeal membrane oxygenation.
Perioperative Support
Indications for ECMO support surrounding cardiac surgery include preoperative stabilization, failure to wean a patient from CPB, postoperative low cardiac output syndrome (LCOS), and postoperative cardiopulmonary arrest (CPA).
Preoperative stabilization may be required in infants with profound cyanosis, cardiogenic shock, or severe pulmonary hypertension (e.g., infants with d-transposition of the great arteries).
ECMO use for failure to wean from CPB typically entails direct cannulation of the right atrium and the aorta via the median sternotomy (central cannulation). Outcomes of patients who fail to wean from CPB have been shown to be worse than outcomes of those who are cannulated onto ECMO for LCOS or CPA occurring after a period of stability. Reported rates of mortality reported after postcardiotomy ECMO in infants and children range between 29% and 61%. Some of the risk factors for mortality in patients supported on ECMO postoperatively are peak serum lactate level within 24 hours of ECMO support initiation, renal failure requiring hemodialysis during the ECMO course, sepsis, increased blood transfusion requirements, and ECMO duration.
Residual cardiac defects need to be considered as potential cause for postoperative low cardiac output. Transesophageal echocardiography, direct cardiac chamber pressure, and oxygen saturation measurements can assist in diagnosing residual cardiac defects. An intraoperative review of preoperative data and imaging can also be helpful.
Determining contraindications for perioperative ECMO support in infants and children who undergo cardiac surgery is a complex process and varies widely among centers providing cardiac surgical care. Some historically absolute contraindications have evolved into relative contraindications in recent years, including prematurity, pre-ECMO neurologic injury, multisystem organ failure, and severe coagulopathy. Heparinization of the circuit may worsen hemorrhage (e.g., intracranial hemorrhage, uncontrolled visceral bleeding), and thus ECMO may be deleterious in these cases. Weight less than 2 kg can pose technical challenges to ECMO cannulation and support. When health care teams are considering ECMO support, it is important to understand that ECMO is only a support modality and does not offer any therapeutic benefit. Thus children who benefit from perioperative ECMO support have a reversible reason for their acute refractory heart failure.
The mean duration of cardiac postoperative ECMO course was 6 days in neonates and 7 days in pediatric patients reported to the ELSO registry between 2009 and 2016. Longer ECMO runs have been associated with higher mortality. Patients unable to separate from ECMO after 5 to 9 days possibly have a nonreversible cause for cardiac failure and should be considered candidates for cardiac transplantation. Specialized care teams of surgeons, cardiologists, intensivists, nurses, and other providers need to thoughtfully evaluate options, consider patient and family values, and assess regularly the risks and benefits of continued extracorporeal support if there is no improvement in the patient’s condition.
Support for Nonsurgical Conditions
Nonsurgical cardiac conditions supported with ECMO include myocarditis and cardiomyopathy, refractory pulmonary hypertension, intractable arrhythmias with hemodynamic compromise (including from toxic ingestion), septic shock, cardiac trauma, and posttransplantation rejection.
Myocarditis and Cardiomyopathy.
Neonates and children with acute myocarditis supported on ECMO have rates of survival to hospital discharge of 50% and 76%, respectively. ECMO for acute myocarditis is used as bridge to recovery, ventricular assist device (VAD), or transplantation. Although the use of VADs for acute myocarditis has increased in recent years, ECMO is still the support mode of choice for those patients presenting with fulminant acute myocarditis with severe cardiogenic shock or CPA that does not allow for sternotomy and VAD placement.
Pulmonary Hypertension.
Abnormal pulmonary vascular development in children with congenital heart disease and certain types of cardiac lesions (e.g., obstructed total anomalous pulmonary venous connection, truncus arteriosus, aortic origin of pulmonary artery) place postoperative patients at risk for acute pulmonary hypertension with pulmonary hypertensive crises and associated acute right ventricular failure. The American Heart Association (AHA) and American Thoracic Society (ATS) 2015 guidelines recommend the use of mechanical cardiopulmonary support, including ECMO, for refractory pulmonary hypertensive crises in postoperative pediatric patients who undergo cardiac surgery (Class I; Level of Evidence B). Residual lesions (e.g., residual pulmonary venous obstruction) should be ruled out as causes for pulmonary hypertension.
Post–Cardiac Arrest Support
Use of ECMO for support of patients with CPA refractory to conventional CPR (ECPR) and of patients with profound cardiogenic shock following return of spontaneous circulation after CPA has been increasingly reported in the literature. The number of ECPR cases reported to the ELSO registry increased by 35% for neonates and 67% for children between 2009 and 2015, with a mean ECMO duration of 5 days. The AHA 2015 guidelines state that CPR with ECMO (ECPR) may be considered for infants and children with cardiac diagnoses who suffer in-hospital cardiac arrest in settings that allow “expertise, resources, and systems to optimize the use of ECMO during and after resuscitation,” with insufficient evidence to suggest for or against the routine use of ECPR for infants and children with noncardiac diagnoses who suffer in-hospital cardiac arrest (weak recommendation, very low-quality evidence). In large national and international registries, survival rates to hospital discharge range from 40% to 43%. In an analysis of the AHA Get With the Guidelines—Resuscitation (GWTG-R) registry, children with in-hospital CPR of 10 minutes or longer who underwent ECPR had improved survival to hospital discharge and survival with favorable neurologic outcomes (defined as Pediatric Cerebral Performance Category [PCPC] 1 to 3 or unchanged from admission), compared with children with in-hospital CPR of 10 minutes or longer who underwent continued conventional CPR. Duration of CPR has been linked to mortality and neurologic injury after cardiac arrest in several studies, possibly due to decline in quality and effectiveness over time. As such, many centers have implemented rapid-deployment ECMO or ECPR programs, to limit CPR duration and minimize no-flow or low-flow states. With creation of portable ECMO consoles and circuits and improved ultrasonography to facilitate cannula insertion, expansion of these programs in recent years has led to deployment strategies not only in intensive care units and operating rooms, but also in emergency departments and out-of-hospital settings. Pediatric as well as adult ECPR studies suggest that the risk of mortality increases with longer CPR duration, as does the risk of poor neurologic outcomes. On the contrary, even prolonged CPR events longer than 1 hour can be associated with favorable neurologic outcomes. These issues illustrate that maintaining good quality of CPR while awaiting ECMO cannulation is essential to achieving good outcomes after ECPR.
Physiology of Blood Flow and Oxygenation During Extracorporeal Membrane Oxygenation
Biventricular Circulation
Pediatric cardiac ECMO patients have varying degrees of univentricular or biventricular failure, pulmonary hypertension, or pulmonary parenchymal disease. Thus as many as 97% of these patients are supported with venoarterial ECMO, in which venous blood is drained from the right atrium and returned to the aorta, thus providing both cardiac and respiratory support. In contrast, in venovenous ECMO venous blood is drained from the venous circulation and returned to the right atrium. Thus in venovenous ECMO, normal cardiac function is essential. The total systemic blood flow (cardiac output) represents the sum of the extracorporeal flow and the native cardiac output. As opposed to CPB, in which all blood return to the heart is drained through cannulas in the superior and inferior vena cavae, in venoarterial ECMO, only ≈80% of venous return to the heart is drained through the circuit. As long as the heart can pump even small amounts of blood and the aortic valve continues to open, the pulse pressure (difference between the systolic and the diastolic blood pressures) is maintained at ≈10 mm Hg. Severe heart failure without ventricular contractility can be complicated by accumulation of blood from bronchial and thebesian venous flow in the left chambers and subsequent increased pressure in the left ventricle, left atrium, and pulmonary circulation. When this problem is not addressed by an atrial septostomy or placement of a drainage cannula in the left atrium or pulmonary artery, there is increased risk for left ventricle distention, pulmonary edema, and pulmonary hemorrhage. Furthermore, stagnation of blood in the cardiac chambers and pulmonary circulation can lead to clot formation despite anticoagulation.
During venoarterial ECMO, pump flow and systemic vascular resistance are adjusted to maintain age-appropriate cardiac output and mean arterial pressure. Any increase in left ventricular afterload potentially impacting myocardial recovery can be mitigated by reducing systemic vascular resistance or by decreasing preload while maintaining coronary blood flow during venoarterial ECMO support.
In the postoperative cardiac patient requiring ECMO support the goal is to maintain lung inflation and function so that decannulation is not impeded by respiratory failure at the time of cardiac recovery. This may require higher levels of positive end-expiratory pressure and peak inspiratory pressure or alternate modes of ventilation such as high-frequency ventilation or airway pressure release ventilation (see Chapter 23 ). The oxygenator fraction of inspired oxygen (FiO 2 ) and sweep flow rates are adjusted to maintain normal oxygenation and normal PCO 2 .
The Univentricular Circulation
ECMO has been used in single-ventricle circulations at all surgical stages. Generally the ECMO flow required for patients with single-ventricle physiology is higher than for patients with biventricular physiology, in an effort to maintain the systemic and the pulmonary parallel circulations. As such, patients with Blalock-Taussig (BT) shunt may require extracorporeal flows of 150 to 200 mL/kg/min. The BT shunt is typically maintained open. Standard-sized shunts (3.5 to 4 mm) usually provide enough limitation to pulmonary blood flow that increasing ECMO flow rate most often provides adequate systemic blood flow. Occasionally the shunt needs to be surgically constricted, but this could lead to shunt thrombosis, and diminished flow through the shunt risks pulmonary parenchymal ischemia and potential irreversible lung injury. The position of the right common carotid cannula needs to be checked serially to avoid migration of the tip into the shunt and potential loss of extracorporeal cardiac output. Successful ECMO support in superior cavopulmonary (Glenn) and Fontan circulations has also been reported; these patients often require multisite venous cannulation to provide adequate venous decompression and pump flow.
Equipment
Typical venoarterial and single lumen venovenous circuits ( Fig. 39.1 ) consist of a venous cannula, which drains blood from the right atrium, tubing connecting this cannula to a blood pump, an oxygenator, a heat exchanger, and then returned via the arterial cannula to the aorta in venoarterial ECMO and to the right atrium via the arterial limb of the cannula in venovenous ECMO. An optional venous reservoir can be inserted between the drainage cannula and the pump to serve as a compliance chamber and to allow for noninvasive pressure measurements. Contemporary pumps are mostly centrifugal ( Fig. 39.2 ) , although roller pumps continue to be used successfully, especially in small neonates and infants ( Table 39.1 ). Centrifugal blood pumps are axisymmetric pumps that use an impeller assembly to produce a hydrodynamic pressure gradient through rotational kinetic energy. Roller blood pumps are peristaltic blood pumps that use compression of a circular segment of tubing with positive displacement of fluid. Most contemporary oxygenators use hollow fibers, with extracapillary blood flow and intracapillary gas flow. The sweep gas is the gas applied to the gas phase of the membrane lung. The sweep gas inlet oxygen fraction can range from 0.21 to 1.0 and is controlled by a gas blender. The heat exchanger transfers heat between the blood phase and a fully separated water phase and allows for blood flow returning to the patient to be warmed at a preset temperature. Modern oxygenators may have integrated heat exchangers. A bridge is an optional segment of tubing inserted between the drainage (venous) and the reinfusion (arterial) limbs of the circuit, close to the cannula connections, and can be opened when the venous and arterial ECMO cannulas inserted in the patient are clamped during a weaning trial. Although the clamping isolates the patient from the circuit, opening the bridge allows recirculation at the circuit level, thus allowing the circuit to be viable and the patient returned to ECMO support in case of weaning failure.


Roller Pump | Centrifugal Pump | |
---|---|---|
Pump mechanism | Positive displacement | Centrifugal force |
Pump inflow | Passive drainage | Active drainage |
Pump occlusion | Occlusive pump | Nonocclusive pump |
Retrograde flow | Not possible | Possible |
Pump flow | Predictable | Varies |
Factors affecting pump flow | Pump RPM Displacement volume | Pump RPM Preload and afterload |
Risk of tubing rupture with outflow obstruction | Present | Not present |
Hemolysis | Present | Present |
The ECMO circuit typically contains pump inlet, preoxygenator or premembrane, and postoxygenator or postmembrane pressure monitors ( Fig. 39.3 ). The pressure gradient between the inlet and the outlet of the oxygenator is usually followed as an indicator for oxygenator clot formation. The ECMO circuit may also contain access ports for medication and blood product administration, for connection of ultrafiltration (including hemodialysis) devices, and for blood sampling for laboratory tests. An important safety feature that helps detection and prevention of air embolization is a bubble sensor that is typically placed on the return or reinfusion side of the circuit. These devices shut down the ECMO pump when air bubbles are detected. Modern ECMO circuits can be equipped with noninvasive devices to continuously measure and display measurements such as blood flow rate, hemoglobin concentration, hematocrit, PO 2 , PCO 2 , and mixed venous saturation (SvO 2 ). Surface modification or coating (e.g., poly-2-methoxyethylacrylate, heparin, albumin), is applied to most ECMO circuits to improve biocompatibility and reduce thrombogenesis. Common technical problems encountered with ECMO circuits and potential solutions are presented in Table 39.2 .

Problem | Comments and Possible Solutions |
---|---|
|
|
|
|
|
|
| |
|
|
| |
|
|
| |
|
|
ECMO equipment, including circuits, surgical cart and instruments, cannulas, and connectors should all be stored in easily accessible locations. A mobile ECMO cannulation cart containing cannulas and equipment that can be rapidly mobilized to the bedside at the time of ECMO team activation can facilitate safe and rapid ECMO deployment.
Extracorporeal Membrane Oxygenation Cannulation and Initial Management
ECMO cannulation for failure to separate from CPB or in the immediate postoperative period is typically transthoracic. The arterial cannula in the ascending aorta may be already in place from CPB, and the venous cannula is inserted directly into the right atrium (usually through the right atrial appendage. If possible, cannulas should be selected that are large enough to support 150 mL/kg/min flow with a pump inlet pressure of more than 20 mm Hg (for centrifugal pump ECMO) and an outlet pressure of less than 200 mm Hg . Patients who cannot be successfully weaned from CPB often transition directly from CPB to ECMO and are usually completely heparinized (for their CPB circuit). This can result in a period of bleeding related to anticoagulation that can make the first few hours of ECMO challenging for the care team. In many cases, particularly with the coated and miniaturized circuits currently available, it is common to stop the heparin in these patients and perform the initial several hours of ECMO without anticoagulation to manage the bleeding. In the more common scenario, ECMO is initiated after a period off the CPB circuit, and during this time the surgical team is often able to achieve some control of bleeding by administering protamine to counteract the heparin in the CPB circuit. Achieving hemostasis in the operating room before implementing ECMO is extremely helpful. In these cases a bolus of 75 to 100 U/kg of unfractionated heparin (UFH) can be administered before ECMO initiation, although many surgical teams may still elect to perform the first several hours of postoperative ECMO support without heparin to reduce the likelihood of significant bleeding during the ECMO run. After hemostasis is achieved, all patients on ECMO require anticoagulation with heparin (or another agent if the patient has heparin sensitivity such as documented heparin-induced thrombosis and thrombocytopenia). Anticoagulation strategies are discussed in more detail later. If a prolonged ECMO course is anticipated, de novo neck cannulation or conversion from transthoracic to neck cannulation via the right common carotid artery and right internal jugular vein may be considered in an effort to minimize risk of infection with significantly delayed chest closure.
Following ECMO cannulation, correct position of the ECMO cannulas can be confirmed by x-ray examination, fluoroscopy, or echocardiography ( Figs. 39.4 and 39.5 ). Radiographic confirmation of cannula position in patients cannulated through the neck is helpful and particularly important when cannulation is for venovenous ECMO or when there are issues with venous drainage in venoarterial ECMO. In general the tip of the venous cannula should be in the mid–right atrium or even down to the caval-atrial junction (just where the inferior vena cava [IVC] enters the right atrium), and the tip of the arterial cannula should be in the common carotid artery or in the aortic arch at the entrance of the innominate artery. It is important that the aortic cannula (when placed through the neck) is not inserted too far into the ascending aorta, because damage to the aortic valve from arterial cannulas positioned just above or at the valve level has been reported. If venous drainage is adequate, then it can be presumed that the venous cannula is in an adequate position. There are times that the tip of the venous cannula (when inserted from the neck) can lie against the eustachian valve at the floor of the right atrium, and this may cause intermittent obstruction to flow, requiring the cannula to be pulled back a centimeter or. If the cannula is across the tricuspid valve (often this is confirmed by echocardiography), flows may be adequate, but the cannula will need to be pulled back into the right atrium before the patient can be weaned from ECMO. For femoral venoarterial cannulations the tip of the venous cannula should extend to the mid–right atrium, and the tip of the arterial cannula should extend to the mid–descending aorta. ECMO providers should be familiar with their institution’s cannula types (i.e., radiopaque to the tip or not) to correctly determine when examining radiographs where each cannula ends. In a single-center pediatric study published in 2009, echocardiography was found to be superior to chest radiographs for evaluating ECMO cannula position. When there is concern about cannula position, it is prudent to discuss those concerns with the surgical team. Most cardiac cases require venoarterial ECMO. In the rare instances of respiratory failure in a cardiac patient, venovenous ECMO may be appropriate. In these patients, correct catheter placement is essential and can be guided by fluoroscopy and/or echocardiogram (see Fig. 39.5 )


Rapid-Deployment Extracorporeal Membrane Oxygenation
Institutions that use ECMO as the primary mechanical support device for emergent circulatory support have developed rapid-deployment strategies to decrease the time required to initiate ECMO support. One or two circuits primed with crystalloid are maintained sterile for a set period of time (usually no longer than 1 month), ready for use in the case of ECMO cannulation during CPR or ECPR. Older children (usually above 20 kg) can be placed on ECMO emergently using the crystalloid-primed circuit, with subsequent typed and cross-matched blood transfusion to reestablish normal hemoglobin concentration if needed. Younger children may require a blood-primed circuit primed with type O, Rh-negative blood to reduce hemodilution. Availability of surgeons, in-house intensivists, and surgical team and equipment are requirements for successful and timely ECPR cannulation. High-quality CPR needs to be maintained for the entire duration of the cardiac arrest, with minimal interruptions for cannula placement, in an effort to avoid hypoxic injury. Team training through multidisciplinary simulations has been shown to decrease time from onset of cardiac arrest to time on ECMO in real patients in a pediatric cardiac intensive care unit.
Critical Care Management During Extracorporeal Membrane Oxygenation Support
Extracorporeal Membrane Oxygenation Teams: a Multidisciplinary Approach
ECMO is a complex, high-risk, and resource-intensive process with unpredictable resource use. Cardiac ECMO centers maintain well-trained multidisciplinary teams, with a core set of cardiac surgeons, intensivists, ECMO specialists, nurses, and perfusionists, as well as consultants from areas such as cardiology, pharmacy, nutrition, transfusion medicine, hematology, pulmonology, and nephrology. Multidisciplinary ECMO training using medical simulation is gaining ground in many cardiac ECMO centers after growing evidence for improved technical skills and team performance.
Neurologic Monitoring and Neuroprotection
Critically ill neonatal and pediatric cardiac ECMO patients are at high risk for neurologic injury surrounding their ECMO course. As many as 14% to 36% of ECMO patients suffer acute neurologic injury surrounding their ECMO course, including hypoxic-ischemic injury, thromboembolic stroke, and intracranial hemorrhage. Neurologic injury during ECMO is associated with an 89% increase in risk of mortality.
The mechanisms of neurologic injury in ECMO patients are not yet well understood. Profound cardiopulmonary failure and/or cardiac arrest lead to hypoxic-ischemic injury before ECMO, rendering the brain vulnerable. The profound inflammatory state associated with critical illness is then compounded by exposure to the foreign surfaces of the extracorporeal circuit, which triggers a profound global innate immune response by activating the contact and complement systems and provokes perturbations in proinflammatory and prothrombotic pathways implicated in the pathogenesis of neurologic injury during ECMO. The device-induced proinflammatory state and endothelial cell dysfunction increase thrombin generation and platelet activation. Within the patient the device contributes to coagulation factor consumption, platelet exhaustion, and reduced platelet aggregation potential. Added to that is the need for systemic anticoagulation to prevent circuit thrombus formation, creating the common paradox of thrombotic events in the device with simultaneous bleeding in the patient. Thus patients are at risk for both thromboembolic and hemorrhagic intracerebral complications.
A recent systematic review of the literature found that neuromonitoring methods during ECMO are limited and supported primarily by grade 3B-4 evidence from small, single-center studies (median n = 28, interquartile range [IQR], 18-49). Daily transfontanellar cranial ultrasonography for infants with open fontanels has entered routine clinical practice. Near-infrared spectroscopy–based cerebral oximetry and electroencephalography (EEG) are used in many centers. The American Clinical Neurophysiology Society recommends the use of critical care continuous EEG in children on ECMO support. A recent prospective quality improvement project conducted in a single quaternary care pediatric center showed that electrographic seizures occurred in 18% of consecutive neonates and children on ECMO support, were more common in patients with LCOS, and were associated with higher mortality and unfavorable neurologic outcome at hospital discharge. Other neuromonitoring methods, including transcranial Doppler ultrasonography and the serial monitoring or plasma brain injury biomarkers are being investigated and showing early promising results for detection of impending neurologic injury during ECMO and for prediction of unfavorable neurologic outcomes.
The best neurologic monitoring strategy is to minimize the use of sedatives and neuromuscular blocking agents. Limiting sedation when possible (e.g., in the absence of severe pulmonary hypertensive crises) has many other advantages, including spontaneous breathing and ability to interact with family and staff.
Respiratory Support
Respiratory management of neonatal and pediatric cardiac patients on ECMO is aimed at maintaining the lungs open and avoiding ventilator-induced lung injury, while taking into consideration cardiopulmonary and device-pulmonary interactions. In general the goal for neonatal and pediatric cardiac patients on ECMO is to avoid atelectasis, mobilize secretions, and, if present, support resolution of pulmonary edema due to capillary leak after large-volume transfusions, post CPB, or post cardiac arrest. Pressure control ventilation is used with moderately high positive end-expiratory pressure of approximately 10 cm H 2 O, rate of 10 breaths/min, and pressures adjusted to achieve 6 to 8 mL/kg tidal volume, limiting peak inspiratory pressures to 18 to 20 cm H 2 O. Limiting sedation and allowing for spontaneous respirations with pressure support or extubation on ECMO are becoming more common, especially in older children and adolescents, and may allow for improved cardiopulmonary interactions.
Nutrition
There are few published articles on optimal nutrition during ECMO. Generally a combination of enteral and parenteral nutrition is provided based on the patient’s nutritional requirements. The benefits of enteral nutrition have been well documented in critical illness at all ages, with recent data suggesting that early parenteral nutrition may be detrimental in critically ill children. Enteral nutrition has been shown to be feasible during venoarterial ECMO in neonates and children, potentially rendering benefits and cost savings. Some of the reported barriers for uninterrupted enteral nutrition during ECMO are ongoing vasopressor requirement, fasting for therapeutic or diagnostic procedures, and high gastric residual volumes. None of the neonatal, pediatric, or adult studies of nutrition during ECMO have reported complications related to early initiation of enteral nutrition in these patients. Antacids are commonly used for prophylaxis against acute gastritis.
Renal Supportive Therapy
Acute kidney injury is present at ECMO initiation in 60% to 74% of neonatal and pediatric patients and present by 48 hours of ECMO support in 86% to 93% of patients. Renal support therapy, most commonly in the form of slow continuous ultrafiltration and continuous venovenous hemofiltration, is used in a majority of neonatal and pediatric ECMO centers.
In neonates and children with critical cardiac disease, acute kidney injury may precede ECMO cannulation due to low cardiac output states, cardiac arrest, or exposure to nephrotoxic drugs. Acute kidney injury represents the most common indication for renal support therapy in cardiac ECMO centers and can worsen during the ECMO course if diuresis or hemofiltration are employed aggressively in an effort to augment fluid clearance and advance the patient toward decannulation. Although fluid overload and acute kidney injury are associated with increased duration of ECMO support and with increased mortality before hospital discharge, controversy remains as to the optimal rate of fluid removal and the optimal timing of initiation of renal support therapy during ECMO. If patients have adequate support on ECMO and can diurese adequately using their kidneys (with reasonable diuretic stimulation), then it may not be necessary to use forms of filtration. When there is impaired kidney function (usually heralded by decreased urine output despite adequate ECMO flow and often associated with rising levels of creatinine and blood urea nitrogen), then other forms of fluid management need to be considered. In some patients there is diffuse capillary leak resulting in third spacing, and in these patients, urine output may not be adequate despite reasonable ECMO flows. Often these patients have normalizing lactates (suggesting appropriate systemic oxygen delivery from the ECMO circuit) and a low central venous pressure. As long as it is possible to maintain flows in these patients, the capillary leak should recover, and as they begin to mobilize their third-spaced fluid, their urine output should recover and respond to diuretics. In some institutions these patients are managed with peritoneal drainage catheters (simply to drain the ascites and take any potential “pressure” off the renal vessels). When ascites reaches the point that an abdominal “compartment syndrome” is present (which can be measured by an elevated bladder pressure), then insertion of a peritoneal drain is a good idea. Patients who have true renal failure with low urine output, rising creatinine level, and high central venous pressures will often require some other form of filtration and are good candidates for continuous ultrafiltration while on ECMO. The decision making around the best management of low urine output requires multidisciplinary collaboration because often these patients present with a variety of reasons for low urine output that may require differing management strategies—one “solution” does not necessarily fit all circumstances.
Infection Risk and Surveillance
The prevalence of hospital-acquired infections during ECMO support is estimated at 10% to 12%, higher than in non-ECMO critically ill patients. Meticulous attention to hand hygiene and adherence to hospital-acquired infection prevention bundles is required to reduce risk of infection in ECMO patients. Although there are no data to support the notion that antibiotic prophylaxis beyond the pericannulation period (24 to 48 hours post cannulation) reduces the risk of infections acquired during ECMO, prolonged courses of antibiotics are commonly used to provide infection prophylaxis in many ECMO programs. Antibiotics commonly used for infection prophylaxis include cefazolin and vancomycin. Rarely antifungal agents are used as part of antibiotic prophylaxis. Use of aminoglycoside antibiotics for infection prophylaxis has been shown to be associated with increased incidence of sensorineural deafness in ECMO survivors and should be avoided when possible. Care of the skin to prevent pressure ulcers and preserving mobility of joints are important aspects of ECMO patient care.
Anticoagulation and Blood Product Management
Upon initiation of ECMO support, exposure of blood to the foreign surfaces of the circuit triggers an inflammatory response with activation of the coagulation pathway and blood elements (leukocytes, platelets) and thrombin activation, leading to a hypercoagulable state in the device. Anticoagulation to maintain patency of the circuit therefore needs to be balanced with the risk of bleeding within the patient. Despite accumulating experience with anticoagulation management and ever-improving biocompatibility of ECMO circuits, hemorrhage and thrombosis remain important complications during ECMO support and can be life threatening.
ECMO centers report large variability in anticoagulation monitoring and management. In an international survey conducted in 2012, 97% of respondents reported using activated clotting times (ACTs) to monitor UFH anticoagulation. Typically heparin infusion rates range from 10 to 40 U/kg/h and are adjusted for ACT targets of 180 to 220 seconds. Studies have shown, however, that ACT is an indirect and often inaccurate reflection of heparin effect and can be outside the target range for many reasons. Therefore currently most centers also monitor activated partial thromboplastin time (aPTT) (94%), anti-factor Xa (aFXa) (65%), thromboelastography (TEG) (43%), and antithrombin activity (82%), at varying time intervals. In addition, fibrinogen, D-dimers and platelet counts are measured serially to provide a more complete assessment of the patient’s coagulation status. aFXa is a reflection of actual heparin levels, and a target range is usually 0.3 to 0.7 to ensure adequate heparinization. Concomitant antithrombin III (AT III) levels can indicate the appropriate substrate for heparin to function, and when AT III levels are low, additional AT III (either as fresh frozen plasma or as a commercially available product [Thrombate III]) can be provided. Monitoring aFXa and AT III levels with adjustments as necessary has correlated with outstanding outcomes with respect to coagulation management in ECMO patients and is currently our preferred anticoagulation protocol.
ACT has several limitations; it is a global test of whole blood coagulation response, it has limited reproducibility, and results differ for different devices. ACT can be influenced by factors such as the presence of heparin, platelet count, platelet dysfunction, hyperfibrinogenemia or hypofibrinogenemia, other coagulation factor deficiencies, hypothermia, or hemodilution. In addition, ACT has poor correlation with aPTT and aFXa during neonatal and pediatric ECMO, and some have proposed that aFXa may be preferable as a marker for degree of anticoagulation during ECMO. In a single-center review comparing an anticoagulation protocol primarily driven by ACT versus aFXa and that included TEG and antithrombin measurements, Northrop et al. demonstrated significant reductions in blood product transfusions, cannula and surgical site bleeding, as well as improved circuit life with the aFXa-driven protocol.
Antithrombin measurement and administration have become common practices in neonatal and pediatric ECMO, despite the lack of robust evidence on efficacy and safety of both pooled and recombinant formulations. Antithrombin pharmacokinetics and pharmacodynamics during ECMO are poorly described. Data from single-center studies suggest that, in selected populations, antithrombin replacement can decrease exposure to blood products and improve efficiency of anticoagulation with unfractionated heparin (UFH) at least temporarily. There is still significant debate and controversy, however, regarding the safety of antithrombin administration, as well as contradictory reports of adverse events associated with antithrombin administration that will require further prospective study.
Alternatives to UFH, including direct thrombin inhibitors such as bivalirudin and argatroban, are being increasingly used in ECMO patients of all ages; the use of these agents in pediatrics patients has been reported in those who develop heparin resistance or heparin-induced thrombocytopenia. Some adult ECMO centers use bivalirudin as the first agent for anticoagulation, with significantly simplified anticoagulation management protocols.
Postoperative neonatal and pediatric cardiac patients on ECMO are at high risk for bleeding. Minor local bleeding such as bleeding at the cannulation site can be controlled with local measures, correction of coagulopathy via plasma, cryoprecipitate, or platelet transfusions and reducing the level of anticoagulation used. Massive bleeding requires prompt blood product administration, including platelets for a goal of 75,000 to 100,000/mm 3 , and administration of antifibrinolytics such as epsilon-aminocaproic acid. UFH infusion rate can be decreased or interrupted in the contemporary era of surface-modified ECMO circuits in cases of severe bleeding, although the risks and benefits of doing so must be discussed at the bedside, taking into careful consideration the cause of bleeding, thrombus burden in the circuit, and each institution’s circuit specification. The use of activated factor VII to control bleeding on ECMO has been reported from some centers. Access to a fresh primed ECMO circuit should be readily available when ECMO bleeding management requires stopping anticoagulation and administration of prothrombotic agents.
Even in the absence of active bleeding, red blood cells and platelets may suffer shear injury during ECMO, with subsequent hemolytic anemia and thrombocytopenia. Thus transfusion of red blood cells may be needed to maintain hemoglobin concentrations that optimize oxygen delivery (typically 10 g/dL), and platelet transfusions may be needed to maintain platelet counts deemed safe for avoiding spontaneous hemorrhage in the face of ongoing anticoagulation (typically 75,000 to 100,000/mm 3 ). Plasma or cryoprecipitate is administered for goal fibrinogen concentrations of 100 mg/dL, or 150 mg/dL before surgical procedures.
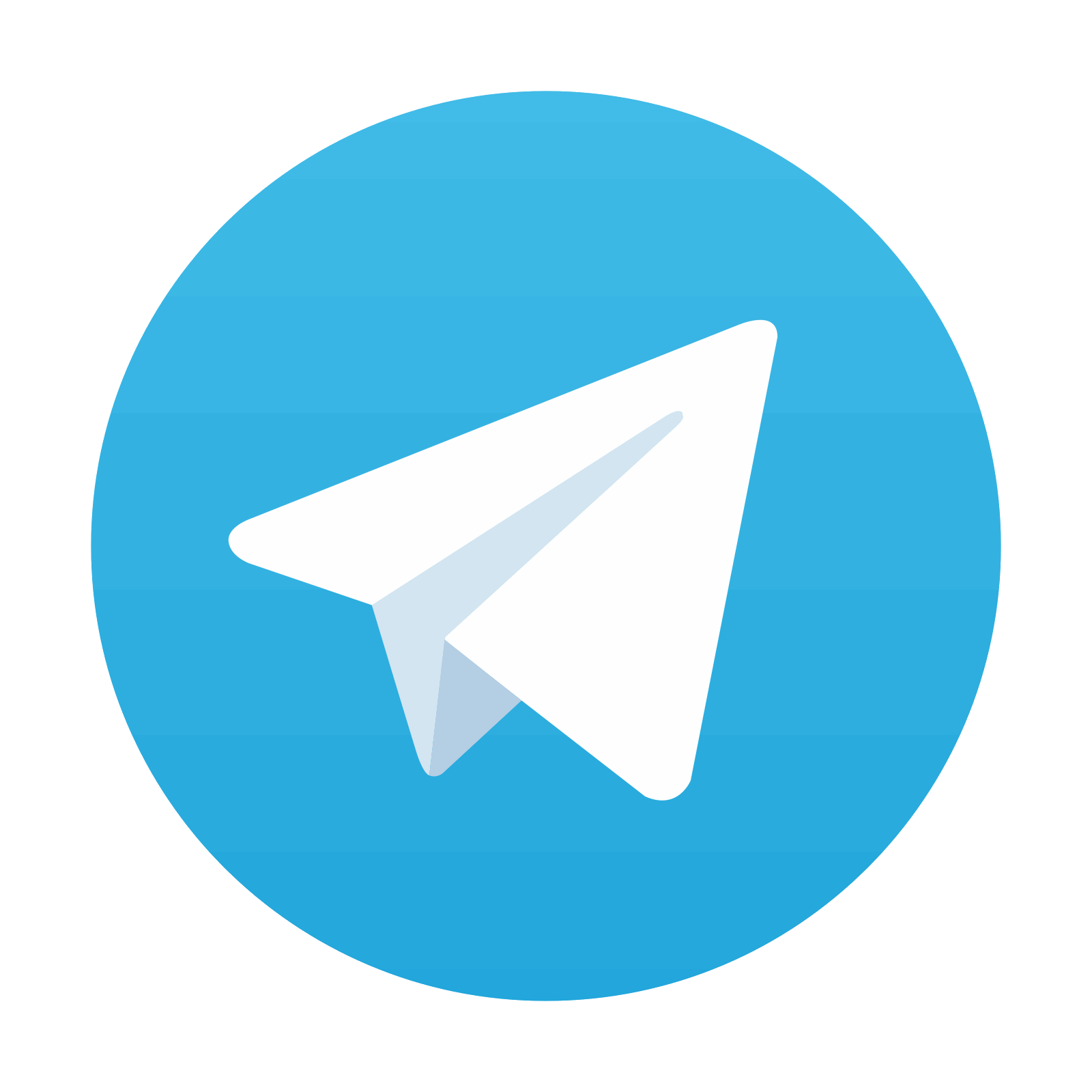
Stay updated, free articles. Join our Telegram channel

Full access? Get Clinical Tree
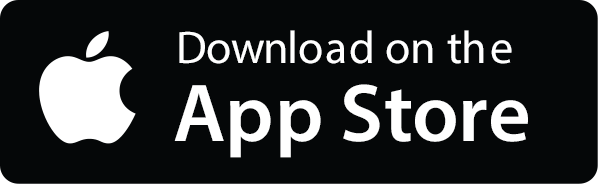
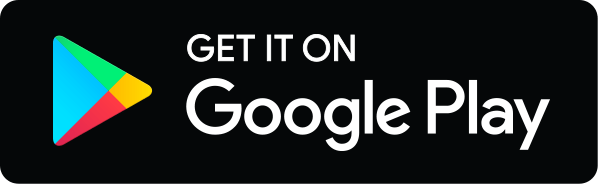