Cardiopulmonary bypass (CPB) for support during cardiac surgery is unique because blood exposed to foreign, nonendothelial cell surfaces is collected and continuously recirculated throughout the body. This contact with synthetic surfaces within the perfusion circuit, as well as open tissue surfaces within the wound, triggers a defense reaction that involves at least five plasma protein systems and five types of circulating blood cells. This inflammatory response to CPB initiates a powerful thrombotic stimulus and the production, release, and circulation of vasoactive and cytotoxic substances that affect every organ and tissue within the body. Because of this, open-heart surgery (OHS) using CPB is essentially not possible without anticoagulation, usually with heparin; thus the inflammatory response to CPB involves the consequences of exposing heparinized blood to foreign surfaces, not lined with endothelial cells.
Although the inflammatory response has been well characterized, the development of a nonthrombogenic artificial surface that would allow heparin-free circulatory support has not yet occurred. This chapter summarizes the application of extracorporeal circulation in adult cardiac surgery, and is divided into three sections. The first section describes the components and operation of perfusion systems and related special topics. The issues of thrombosis and bleeding are addressed in the second section, whereas the humoral response to CPB, including the reaction of blood elements and the inflammatory response are presented later in this chapter. The final section deals with organ damage as a consequence of extracorporeal perfusion (ECP).
During CPB for cardiac surgery, blood is typically drained by gravity into the venous reservoir of the heart-lung machine via cannulas placed in the superior vena cava (SVC) and inferior vena cava (IVC) or a single cannula placed in the right atrium. Specialized cannulas can also be placed into the lower IVC through a femoral approach. Blood from the reservoir is then pumped through a hollow fiber oxygenator, and after appropriate gas exchange takes place, into the systemic arterial system through a cannula placed in the distal ascending aorta, the femoral artery, or the axillary artery (Fig. 13-1). This basic ECP system can be adapted to provide partial or total circulatory and respiratory support or partial support for the left or right heart or for the lungs separately.
The complete heart-lung machine includes many additional components (Fig. 13-2).1 Most manufacturers consolidate a hollow-fiber oxygenator, venous reservoir, and heat exchanger into a single unit. A microfilter-bubble trap is added to the arterial outflow. Depending on the operation, various suction systems can be used to return blood from the surgical field, cardiac chambers, and/or the aorta, directly back into the cardiotomy reservoir, through a microfilter and then into the venous reservoir. Increasing evidence of the potential harmful effects of returning fat and lipid particles from the field into directly into the circulation, have increasingly led surgeons to preferentially use a cell saver system to collect and wash shed blood within the surgical field, and return the blood to the patient or circuit as packed red cells. In addition to adjusting pump flow, partially occluding clamps on venous and arterial lines allow additional regulation of venous drainage and flow. Access ports for sampling and sensors for monitoring pressures, temperatures, oxygen saturation, blood gases, and pH are included within most CPB systems. A separate pump and circuit for the administration of cardioplegic solutions at controlled composition, rate, and temperature is usually included in the system. An ultrafilter can be easily added within the circuit for the removal of excess fluid, electrolytes, and some inflammatory mediators, or simply for hemoconcentration.
FIGURE 13-2
Diagram of a typical cardiopulmonary bypass circuit with vent, field suction, aortic root suction, and cardioplegic system. Blood is drained from a single “two-stage” catheter into the venous reservoir, which is part of the membrane oxygenator/heat exchanger unit. Venous blood exits the unit and is pumped through the heat exchanger and then the oxygenator. Arterialized blood exits the oxygenator and passes through a filter/bubble trap to the aortic cannula, which is usually placed in the ascending aorta. Blood aspirated from vents and suction systems enters a separate cardiotomy reservoir, which contains a microfilter, before entering the venous reservoir. The cardioplegic system is fed by a spur from the arterial line to which the cardioplegic solution is added and is pumped through a separate heat exchanger into the antegrade or retrograde catheters. Oxygenator gases and water for the heat exchanger are supplied by independent sources.

Venous blood usually enters the circuit by gravity or siphonage into a venous reservoir placed 40 to 70 cm below the level of the heart. The amount of drainage is determined by central venous pressure, the height differential and any resistance within the system (cannulas, tubing, and connectors). Successful drainage is dependent on a continuous column of blood or fluid and the absence of air within the system. Central venous pressure is determined by intravascular volume and venous compliance, which is influenced by medications, sympathetic tone, and anesthesia. Inadequate blood volume or excessive siphon pressure may cause compliant venous or atrial walls to collapse against cannular intake openings to produce “chattering” or “fluttering.” This phenomenon is corrected by adding volume to the system (circuit and/or patient), or partially occluding the venous line near the inlet to decrease the negative pressure.
Most venous cannulas are made out of flexible plastic, which may be stiffened with wire reinforcement to prevent kinking. Cannula tips may be straight or angled and often are constructed of thin, rigid plastic or metal. Cannula sizes are selected based on patient size and weight, anticipated flow rate, and an index of catheter flow characteristics and resistance (provided by the manufacturer), as well as size of the vessel to be cannulated. For an average adult with 60-cm negative siphon pressure, a 30-French cannula in the SVC, and 34 French in the IVC or a single 42-French cavoatrial catheter almost always provides excellent venous drainage. Thin metal tipped right angle cannulas allow placement of smaller diameter cannulas with equal flow characterisitcs, and assist with insertion directly into the vena cavae. Catheters are typically inserted through purse-string guarded incisions in the right atrial appendage, lateral atrial wall, or directly in the SVC and IVC.
Three basic approaches for central venous cannulation are used: bicaval, single atrial, or cavoatrial (“two-stage”) (Fig. 13-3). Bicaval cannulation and caval tourniquets are necessary to prevent bleeding into the field, and air entry into the system when the right heart is entered during CPB. Because of coronary sinus return, caval tourniquets should not be tightened without decompressing the right atrium if the heart is not still ejecting. Bicaval cannulation without caval snares is sometimes preferred to facilitate venous return during exposure of the left atrium and mitral valve.
Single venous cannulation is adequate for most aortic valve and coronary artery surgery; however, usually a cavoatrial cannula (“two-stage”) is employed (Fig. 13-3B). Introduced via the right atrial appendage, the narrowed distal end is guided into the IVC, leaving the wider proximal portion with multiple side holes to rest within the mid-right atrium. This tends to provide better venous drainage than a single cannula; however, proper positioning is critical.2 Care must be taken with both a single and two-stage cannula as elevation of the heart may kink the superior cavoatrial junction, decreasing venous return, and potentially, and more importantly, impeding venous outflow from the cerebral circulation.
Venous cannulation can also be accomplished via the femoral or iliac veins through an open or percutaneous technique. This technique is frequently employed in emergency situations, where central venous cannulation may be difficult (as in complex redo sternotomies3) or for cardiopulmonary support when a thoracotomy is the approach of choice, as in descending aortic procedures, or redo mitral valve operations. It is also valuable in the support of critically ill or unstable patients prior to the induction of anesthesia, and for applications of CPB that do not require a chest incision. Adequate venous drainage requires the use of larger cannulas (up to 28 French), with the drainage ports either within the intrahepatic IVC or in the right atrium. Transesophageal echocardiography (TEE) can be particularly helpful in assuring proper placement of these cannulas. Specially designed commercially manufactured long, ultrathin, wire-reinforced catheters are available for this purpose. With recent advances in minimally invasive thoracic surgery, longer two stage cannulas which can be inserted percutaneously via the femoral veins and guided superiorly into the SVC and RA have been developed and are now readily available.
A persistent left SVC (PLSVC) is present in 0.3 to 0.5% of the general population and usually drains into the coronary sinus; however, in about 10% of cases it drains into the left atrium.4 Although more common in association with other congenital defects, it can be seen as an isolated anomaly, and should be suspected when the (left) innominate vein is small or absent, or when a large coronary sinus (or the PLSVC itself) is seen on baseline TEE.5
A PLSVC may complicate the delivery of retrograde cardioplegia or entry into the right heart.6 If an adequate-sized innominate vein is present (30% of patients), the PLSVC can simply be occluded during CPB, assuming the ostium of the coronary sinus is present, and the coronary venous drainage is not dependent on the PLSVC.7 If the right SVC is absent (approximately 20% of patients with PLSVC), the left cava cannot be occluded and should be drained. With a normal right SVC (RSVC), but an innominate vein that is absent (40% of patients) or small (about 33%), occlusion of the PLSVC may cause venous hypertension and possible cerebral injury. Although division of the innominate vein during redo-sternotomy or complex surgery such as transplantation has been shown to be safe, occlusion of the PLSVC during CPB relies on drainage of the cerebral venous return by the contralateral system, and so special attention must be paid to assure adequate RSVC cannula size, and prevent any kinking of the RSVC. In circumstances in which retrograde cardioplegia is required (severe aortic insufficiency) in the presence of a PLSVC, the retrograde cardioplegia cannula can be directly inserted into the coronary sinus and secured with a pursestring around the orifice of the coronary sinus, and with temporary snaring of the PLSVC, successful retrograde cardioplegia can be delivered.
Negative pressure can be applied to the venous line to provide assisted venous drainage (AVD) using either a roller or a centrifugal pump system,8 or by applying a regulated vacuum to a closed hard-shell venous reservoir (vacuum-assisted venous drainage, VAVD).9 This may permit use of smaller diameter catheters10 and may be helpful when long, peripheral catheters are used. However augmented negative pressure in the venous line increases the risk of aspirating gross or microscopic air and causing cerebral injury,11,12 hemolysis, or aspiration of air into the blood phase of hollow fiber oxygenators. Conversely, positive pressure in the venous reservoir can cause air to enter the venous lines and the right heart.13 These potential complications require special safety monitors and devices and adherence to detailed protocols when using AVD techniques.13,14
Atrial arrhythmias, bleeding from atrial or vena caval tears, air embolization, venous injury, or obstruction owing to catheter malposition, reversing arterial and venous lines, and unexpected decannulation can all occur during the conduct of cannulation for CPB. Encircling the vena cavae for snaring may lacerate branches or nearby vessels (eg, right pulmonary artery), or injure the vena cava itself. All of these injuries are more likely in the presence of previous surgery, and need to be recognized and corrected early to assure the proper conduct of CPB and minimize additional complications. During cannulation for minimally invasive procedures where placement is more “blind” than with direct open cannulation techniques, the use of ultrasound and TEE may help guide placement of the various cannulas and potentially prevent very serious complications.
Either before or after CPB, cannulas still in place may compromise venous return to the right atrium. The venous cannulas in the SVC or the superior caval tape may displace or compromise central venous or pulmonary arterial monitoring catheters. Conversely, monitoring catheters may compromise the function of caval tapes, allowing air to enter the venous lines between the cannulas and the catheters or sheaths.
During the conduct of the operation itself, any intracardiac catheter may be trapped by sutures, which may impede removal before or after the wound is closed. Any connection between the atmosphere and cannula intake ports may entrain air to produce an air lock or gaseous microembolism. AVD increases the risk of air entrainment.15 Finally, improperly placed pursestring sutures may obstruct a cava when tied, particularly in the SVC.16
Low venous pressure, hypovolemia, drug- or anesthetic-induced venous dilatation, inadequate differential height between the heart and the reservoir, inadequate cannula size, cannula obstruction or kinking, “air-lock,” and excessive flow resistance in the drainage system are all possible causes of impaired or inadequate venous return. These can usually be prevented or quickly corrected through close attention to detail, keeping the venous lines visible within the field when possible, and perhaps most importantly, frequent and detailed communication between surgeon and perfusionist. In addition to contributing to inadequate antegrade flow from the pump, partial obstruction of the venous line may lead to right ventricular distention and impair contractility off CPB.
The tip of the arterial cannula is usually the narrowest part of the perfusion system and may produce high pressure differentials, jets, turbulence, and cavitation at the required flows for CPB, particularly if the arterial catheters are small. Most arterial catheters are rated by a performance index, which relates external diameter, flow, and pressure differential.17 High-velocity jets may damage the aortic wall, dislodge atheroemboli, produce dissections, disturb flow to nearby vessels, and cause cavitation and hemolysis. Pressure differences that exceed 100 mm Hg cause excessive hemolysis and protein denaturation.18 Weinstein19 attributed a predominance of left-sided stroke after cardiac surgery to the “sand-blasting” effect of end-hole aortic cannulas directing debris into the left carotid artery. Available aortic catheters with only side ports20 are designed to minimize jet effects and better distribute arch vessel perfusion and pressure21 and may be associated with fewer strokes.19
Recently a dual-stream aortic perfusion catheter has been developed that features an inflatable horizontal streaming baffle that is designed to protect the arch vessels from atherosclerotic and other emboli and permits selective cerebral hypothermia.22 Another novel aortic cannula features a side port that deploys a 120-μm mesh filter to remove particulate emboli beyond the ascending aorta.23 Although this catheter may increase the pressure gradient by 50%,24 it has been shown to remove an average of eight emboli in 99% of 243 patients studied, and reduce the incidence of cerebral injuries below an expected rate.25
Anatomical sites available for arterial inflow include the proximal aorta, innominate artery and distal arch, femoral, external iliac, axillary, and subclavian arteries. Cannulation can be direct by arterial puncture within a pursestring, through a side graft anastomosed to an arterial vessel, or percutaneous, although usually only in emergency situations. The choice is influenced by the planned operation26 and distribution of atherosclerotic disease.27
Dislodgement of atheromatous debris from the aortic wall from manipulation,28 cross-clamping, or the sand-blasting effect of the cannula jet is a major cause of perioperative stroke29 as well as a risk factor for aortic dissection30 and postoperative renal dysfunction.31 Simple palpation has been shown to be sensitive and accurate for detecting severe atherosclerosis than epiaortic ultrasonic scanning.28,32 Although some have advocated for its use, even TEE views of the middle and distal ascending aorta are often inadequate.32,33 Epiaortic scanning is now the preferred method of screening in all patients with a history of transient ischemic attack, stroke, severe peripheral vascular disease, palpable calcification in the ascending aorta, calcified aortic knob on chest radiograph, age older than 50 to 60 years, or TEE findings of moderate aortic atherosclerosis.28 A calcified aorta (“porcelain aorta”), which occurs in 1.2 to 4.3% of patients,34 is another indication for relocation of the aortic cannula.35 Alternative sites include the distal aortic arch34 along with the innominate, axillary-subclavian, or femoral arteries.
The distal ascending aorta is the most common cannulation site because of easy access, and few complications. The cannula is usually placed through a small stab wound within one or two concentric pursestring sutures that are then snared to secure the cannula and provide hemostasis. Risk of dissection may be reduced by avoiding cannulation into the hypertensive aorta, and many surgeons choose to transiently reduce the systemic pressure below 100 mm Hg. The observation of pulsatile back bleeding into the cannula confirms that the tip is within the lumen of the aorta, and then the cannula should be positioned to direct flow to the mid-transverse aorta. The use of a long catheter with the tip placed beyond the left subclavian artery has also been reported.36 Proper cannula placement is critical21 and is confirmed by noting pulsatile pressure in the aortic line monitor and equivalent pressure in the radial artery. The cannula must be properly secured in place to prevent inadvertant dislodgement during the conduct of the operation.
Complications include difficult insertion; bleeding; tearing of the aortic wall; intramural or malposition of the cannula tip (in or against the aortic wall, toward the valve, or in an arch vessel)37; atheromatous emboli; failure to remove all air from the arterial line after connection; injury to the aortic back wall; high line pressure, indicating obstruction to flow; inadequate or excessive cerebral perfusion38; inadvertent decannulation; and aortic dissection.39 It is essential to monitor aortic line and radial artery pressures and carefully observe the aorta for possible cannula-related complications particularly during the initiation of CPB as well as during the placement of aortic clamps. Asymmetric cooling of the face or neck may suggest a problem with cerebral perfusion. Late bleeding and infected or noninfected false aneurysms are delayed complications of aortic cannulation.
Aortic dissection occurs in 0.01 to 0.09% of aortic cannulations30,40 and is more common in patients with aortic root disease. Early signs of aortic dissection include discoloration beneath the adventia near the cannulation site, an increase in arterial line pressure, or a sharp reduction in return to the venous reservoir. TEE may be helpful in confirming the diagnosis,41 but prompt action is necessary to limit the dissection and maintain perfusion. The cannula must be promptly transferred to a peripheral artery or uninvolved distal aorta. Blood pressure should be controlled pharmacologically and perfusion cooling to temperatures less than 20°C initiated. During hypothermic circulatory arrest, the aorta is opened at the original site of cannulation and repaired by direct suture, patch, or circumferential graft.40 When recognized early, survival rates range from 66 to 85%, but when undiscovered until late during of after the operation, survival is approximately 50%.
These vessels are usually the first alternative to aortic cannulation, but may be the primary choice for rapid initiation of CPB for severe bleeding, cardiac arrest, acute intraoperative dissection, or severe shock. It is also a common first choice for limited access cardiac surgery, as well as in selected reoperative patients.3 Femoral or iliac cannulation limits cannula size but the retrograde distribution of blood flow is similar to antegrade flow.42 Percutaneous cannulation kits are available for emergency femoral access, and many surgeons also use these long wire reinforced peripheral arterial cannulas with an open Seldinger technique, inserting the cannula through a pursestring in the femoral or iliac vessel by direct cutdown. This may reduce some of the complications of open insertion of large short cannluas, and simplifies cannula removal and arterial repair. Femoral cannulation may be associated with many complications,3 including tears, dissection, late stenosis or thrombosis, bleeding, lymphatic collection or drainage, groin infection, and cerebral and coronary atheroembolism. In patients with prior aortic dissections, retrograde femoral perfusion may create a malperfusion situation; thus, some surgeons recommend alternative cannulation sites for these patients.43 Ischemic complications of the distal leg may occur during prolonged (3- to 6-hour) retrograde perfusions,44,45 unless perfusion is provided to the distal vessel. This may be provided by a small Y catheter in the distal vessel45 or a side graft sutured to the artery.46
Retrograde arterial dissection is the most serious complication of femoral or iliac arterial cannulation and may extend to the aortic root or cause retroperitoneal hemorrhage with an incidence of around 1% or less,47 and is associated with a mortality of about 50%. This complication is more common in patients greater than 40 years, and in those with significantly diseased arteries. The diagnosis is similar to an aortic cannula dissection and may be confirmed by TEE of the descending thoracic aorta.41 Antegrade perfusion in the true lumen must be immediately resumed by either the heart itself or cannulation in the distal aorta or axillary-subclavian artery. It is not always necessary to repair the dissected ascending aorta unless it progresses proximally to involve the aortic root.47
The axillary-subclavian artery has been increasingly used for cannulation.48,49 Advantages include freedom from atherosclerosis, antegrade flow into the arch vessels, and protection of the arm and hand by collateral flow. Because of these advantages and the dangers of retrograde perfusion in patients with aortic dissection, some surgeons prefer this cannulation site over femoral access for these patients.49 Brachial plexus injury and axillary artery thrombosis are reported complications.48 The axillary artery is approached through a subclavicular incision, whereas the intrathoracic subclavian artery may be cannulated through a thoracotomy.50
Occasionally the innominate artery may be cannulated through a pursestring suture without obstructing flow to the right carotid artery by using a 7- or 8-French cannula.26 The ascending aorta can also be cannulated by passing a cannula through the aortic valve from the left ventricular apex.51 Coselli and Crawford52 also describe retrograde perfusion through a graft sewn to the abdominal aorta.
The venous reservoir serves as volume reservoir during CPB and particularly with the body exsanguination of deep hypothermic circulatory arrest. It is placed immediately before the arterial pump when a membrane oxygenator is used (see Fig. 13-1). This reservoir serves as a high-capacitance (ie, low-pressure) receiving chamber for venous return, facilitates gravity drainage, is a venous bubble trap, provides access for drugs, fluids, or blood, and increases the storage capacity for the perfusion system. As much as 1 to 3 L of blood may be translocated from patient to circuit when full CPB is initiated. The venous reservoir also provides several seconds of reaction time if venous return is suddenly decreased or interrupted.
Reservoirs may be rigid (hard) plastic canisters (“open” types) or soft, collapsible plastic bags (“closed” types). The rigid canisters facilitate volume measurements and management of venous air, often have larger capacity, are easier to prime, permit suction for VAVD, and may be less expensive. Some hard-shell venous reservoirs incorporate macrofilters and microfilters and can serve as cardiotomy reservoirs to receive vented blood.
Disadvantages include the use of silicon antifoam compounds, which may produce microemboli,53 and increased activation of blood elements.54 Soft bag reservoirs eliminate the blood-gas interface and by collapsing reduce the risk of pumping massive air emboli if venous return is suddenly interrupted.
Interest in miniaturization of the circuit has peaked over recent years in an effort to reduce priming volumes and the need for transfusion with its associated consequences as discussed later in this chapter. A variety of these devices are now available. However as with all “advances”, there are associated disadvantages, primarily the loss of a safety margin of circuit blood volume for continued perfusion should there be a sudden interruption in venous return.
Membrane oxygenators imitate the natural lung by interspersing a thin membrane of microporous polypropylene or polymethylpentene (0.3- to 0.8-μm pores), or silicone rubber between the gas and blood phases. Compared with previously used bubble oxygenators, membrane oxygenators are safer, produce less particulate and gaseous microemboli,55 are less reactive to blood elements, and allow superior control of blood gases.56 With microporous membranes, plasma-filled pores prevent gas entering blood, but facilitate transfer of both oxygen and CO2. Because oxygen is poorly diffusible in plasma, blood must be spread as a thin film (approximately 100 μm) over a large area with high differential gas pressures between compartments to achieve oxygenation. Areas of turbulence and secondary flow enhance diffusion of oxygen within blood and thereby improve oxyhemoglobin saturation.57 Carbon dioxide is highly diffusible in plasma and easily exits the blood compartment despite small differential pressures across the membrane.
The most popular design uses sheaves of hollow fibers (120-200 μm) connected to inlet and outlet manifolds within a hard-shell jacket (Fig. 13-4). The most efficient configuration creates turbulence by passing blood between fibers and oxygen within fibers. Arterial PCO2 is controlled by gas flow rate, and PO2 is controlled by the fraction of inspired oxygen (FIO2) produced by an air-oxygen blender. Modern membrane oxygenators add up to 470 mL of O2 and remove up to 350 mL CO2 per minute at 1 to 7 L of flow with priming volumes of 220 to 560 mL and resistances of 12 to 15 mm Hg per liter blood flow. Most units combine a venous reservoir, heat exchanger, and hollow fiber membrane oxygenator into one compact unit.
FIGURE 13-4
Diagram of a hollow fiber membrane oxygenator and heat exchanger unit. Blood enters the heat exchanger first and flows over water-cooled or water-warmed coils and then enters the oxygenator to pass between woven strands of hollow fibers. Oxygen enters one end of the bundles of hollow fibers and exits at the opposite end. The hollow fiber bundles are potted at each end to separate the blood and gas compartments. Oxygen and carbon dioxide diffuse in opposite directions across the aggregate large surface of the hollow fibers.

Oxygen and CO2 diffuse across thin silicone membranes, which are made into envelopes and wound around a spool to produce a spiral coil oxygenator. Gas passes through the envelope and blood passes between the coil windings. Because of protein leakage that frequently occurs with hollow fiber membranes after 8 to 12 hours of use, these spiral coil silicone membranes have been preferred for the prolonged perfusions (days) used in long-term respiratory and cardiac support of extracorporeal membrane oxygenation or “ECMO” systems. More recently, however, the development of polymethylpentene oxygenators have combined the benefit of efficient small surface area hollow fiber oxygenators without the detrimental plasma leakage seen with polypropylene, and have allowed these membranes to be used with both CPB and ECMO.
Other membranes feature a very thin (0.05 μm), solid membrane on the blood side of a highly porous support matrix. This membrane reduces the risk of gas emboli and plasma leakage during prolonged CPB, but may impair transfer of volatile anesthetics.58
Flow regulators, flow meters, gas blender, oxygen analyzer, gas filter, and moisture trap are parts of the oxygenator gas supply system used to control the ventilating gases within membrane oxygenators. Often an anesthetic vaporizer is added, but care must be taken to prevent volatile anesthetic liquids from destroying plastic components of the perfusion circuit.
Bubble oxygenators are obsolete in the United States, but may still be used elsewhere for short-term CPB because of low cost and efficiency. Because each bubble presents a new foreign surface to which blood elements react, bubble oxygenators cause progressive injury to blood elements and entrain more gaseous microemboli.59 In bubble oxygenators, venous blood drains directly into a chamber into which oxygen is infused through a diffusion plate (sparger). The sparger produces thousands of small (approximately 36 μm) oxygen bubbles within blood. Gas exchange occurs across a thin film at the blood-gas interface around each bubble. Carbon dioxide diffuses into the bubble and oxygen diffuses outward into blood. Small bubbles improve oxygen exchange by effectively increasing the surface area of the gas-blood interface,60 but are difficult to remove. Large bubbles facilitate CO2 removal. Bubbles and blood are separated by settling, filtration, and defoaming surfactants in a reservoir. Bubble oxygenators add 350 to 400 mL oxygen to blood and remove 300 to 330 mL CO2 per minute at flow rates from 1 to 7 L/min.56 Priming volumes are less than 500 mL. Commercial bubble oxygenators incorporate a reservoir and heat exchanger within the same unit and are placed upstream to the arterial pump.
Oxygenator malfunction requiring change during CPB occurs in 0.02 to 0.26% of cases,61-63 but the incidence varies between membrane oxygenator designs.64 Development of abnormal resistant areas in the blood path is the most common cause,63 but other problems include leaks, loss of gas supply, rupture of connections, failure of the blender, and deteriorating gas exchange. Blood gases need to be monitored to ensure adequate CO2 removal and oxygenation. Heparin coating may reduce development of abnormally high resistance areas.62
Heat exchangers control body temperature by heating or cooling blood passing through the perfusion circuit. Hypothermia is frequently used during cardiac surgery to reduce oxygen demand or facilitate operative exposure with brief periods of circulatory arrest. Because gases are more soluble in cold than in warm blood, rapid rewarming of cold blood within the circuit or the body may cause formation of bubble emboli.65 Most membrane oxygenator units incorporate a heat exchanger upstream to the oxygenator to minimize this potential problem. Blood should not be heated above 40°C to prevent denaturation of plasma proteins, and the temperature gradient between the body and the perfusion circuit remains within 10°C to prevent bubble emboli. The heat exchanger may be supplied by hot and cold tap water, but separate heater/cooler units with convenient temperature-regulating controls are preferred. Leakage of water into the blood path can cause hemolysis and malfunction of heater/cooler units may occur.61
Separate heat exchangers are needed for cardioplegia. The simplest system uses bags of precooled cardioplegia solution; however, commonly cardioplegia fluid is circulated through a dedicated heat exchanger or tubing coils placed in an ice or warm water bath.
Most heart-lung machines use two types of pumps, although roller pumps can be used exclusively (Table 13-1). Centrifugal pumps are usually used for the primary perfusion circuit for safety reasons and for a possible reduction in injury to blood elements, although this remains controversial and unproven.66
Roller pump | Centrifugal pump | |
---|---|---|
Description | Nearly occlusive | Nonocclusive |
Afterload independent | Afterload sensitive | |
Advantages | Low prime volume | Portable, position insensitive |
Low cost | Safe positive and negative pressure | |
No potential for backflow | Adapts to venous return | |
Shallow sine-wave pulse | Superior for right or left heart bypass | |
Preferred for long-term bypass | ||
Protects against massive air embolism | ||
Disadvantages | Excessive positive and negative pressure | Large priming volume |
Spallation | Requires flowmeter | |
Tubing rupture | Potential passive backward flow | |
Potential for massive air embolism | Higher cost | |
Necessary occlusion adjustments | ||
Requires close supervision |
Centrifugal pumps (Fig. 13-5) consist of a vaned impeller or nested, smooth plastic cones, which when rotated rapidly, propel blood by centrifugal force.67 An arterial flowmeter is required to determine forward blood flow, which varies with the speed of rotation and the afterload of the arterial line. Unless a check valve is used,68 the arterial line must be clamped to prevent backward flow when the pump is off. Centrifugal blood pumps generate up to 900 mm Hg of forward pressure, but only 400 to 500 mm Hg of negative pressure and, therefore, less cavitation and fewer gaseous microemboli. They can pump small amounts of air, but become “deprimed” if more than 30 to 50 mL of air enters the blood chamber. Centrifugal pumps are probably superior for temporary extracorporeal cardiac assist devices and left heart bypass, and for generating pump-augmented venous return.
FIGURE 13-5
Diagrams of blood pumps. (A) Roller pump with two rollers, 180° apart. The compression of the rollers against the raceway is adjustable and is set to be barely nonocclusive. Blood is propelled in the direction of rotation. (B) The impeller pump uses vanes mounted on a rotating central shaft. (C) The centrifugal pump uses three rapidly rotated, concentric cones to propel blood forward by centrifugal force.

Roller pumps consist of a length of 1/4- to 5/8-inch (internal diameter) polyvinyl, silicone, or latex tubing, which is compressed by two rollers 180° apart, inside a circular raceway. Forward flow is generated by roller compression and flow rate depends upon the diameter of the tubing, rate of rotation (RPM), the length of the compression raceway, and completeness of compression or “occlusion.” Compression is adjusted before use to be barely nonocclusive against a standing column of fluid that produces 45 to 75 mm Hg back pressure.69 Hemolysis and tubing wear are minimal at this degree of compression.69 Flow rate is determined from calibration curves for each pump for different tubing sizes and rates of rotation. Roller pumps are inexpensive, reliable, safe, insensitive to after-load, and have small priming volumes, but can produce high negative pressures and microparticles shed from compressed tubing (spallation).70 Roller pumps are vulnerable to careless operation that results in propelling air; inaccurate flow calibration; backflow when not in use if rollers are not sufficiently occlusive; excessive pressure with rupture of connections if arterial inflow is obstructed; tears in tubing; and changing roller compression settings during operation. In general roller pumps rather than centrifugal pumps are used for sucker systems and for delivering cardioplegic solutions.
Centrifugal pumps produce pulseless blood flow and standard roller pumps produce a sine wave pulse around 5 mm Hg. The arterial cannula dampens the pulse of pulsatile pumps, and it is difficult to generate pulse pressures above 20 mm Hg within the body during full CPB.71 To date no one has conclusively demonstrated the need for pulsatile perfusion during short- or long-term CPB or circulatory assistance.72
Complications that may occur during operation of either type of pump include loss of electricity; loss of the ability to control pump speed, which produces “runaway pump” or “pump creep” when turned off; loss of the flow meter or RPM indicator; rupture of tubing in the roller pump raceway; and reversal of flow by improper tubing in the raceway. A means to manually provide pumping in case of electrical failure should always be available.
During clinical cardiac surgery with CPB the wound and the perfusion circuit generate gaseous and biologic and nonbiologic particulate microemboli (<500 μm diameter).23,73,74 Microemboli produce much of the morbidity associated with cardiac operations using CPB (see section “Organ Damage” later in this chapter). Gaseous emboli contain oxygen or nitrogen and may enter the perfusate from multiple sources and pass through other components of the system.12,15 Potential sources of gas entry include stopcocks, sampling and injection sites,74 priming solutions, priming procedures, intravenous fluids, vents, the cardiotomy reservoir, tears or breaks in the perfusion circuit, loose pursestring sutures (especially during augmented venous return),12 rapid warming of cold blood,65 cavitation, oxygenators, venous reservoirs with low perfusate levels,15 and the heart and great vessels. Bubble oxygenators produce many gaseous emboli; membrane oxygenators produce very few.55,56 Aside from technical errors (open stopcocks, empty venous reservoir, air in the heart) the cardiotomy reservoir is the largest source of gaseous emboli in membrane oxygenator perfusion systems.
Blood produces a large number of particulate emboli related to thrombus formation (clots), fibrin, platelet and platelet-leukocyte aggregation, hemolyzed red cells, cellular debris, and generation of chylomicrons, fat particles, and denatured proteins.75 Stored donor blood is also an important source of blood-generated particles.76 Other biologic emboli include atherosclerotic debris and cholesterol crystals and calcium particles dislodged by cannulation, manipulation for exposure, or the surgery itself. Both biologic and nonbiologic particulate emboli are aspirated from the wound. Bits of muscle, bone, and fat are mixed with suture material, talc, glue, and dust and aspirated into the cardiotomy reservoir.76,77 Materials used in manufacturing, spallated material, and dust may also enter the perfusate from the perfusion circuit76 if it is not first rinsed by recirculating saline through a prebypass microfilter, which is discarded.
In vivo microemboli larger than 100 μm are detected by transcranial Doppler ultrasound,78 fluorescein angiography,55 TEE, and retinal inspection. In the circuit, microemboli are monitored by arterial line ultrasound79 or monitoring screen filtration pressure. Microfilter weights and examination, histology of autopsy tissues, and electron particle size counters of blood samples76 verify microemboli beyond the circuit.
Table 13-2 outlines sources of microemboli. Major methods include using a membrane oxygenator and cardiotomy reservoir filter; minimizing and washing blood aspirated from the field80; and preventing air entry into the circuit and using left ventricular vents when the heart is opened.81,82
Gas | Foreign | Blood |
---|---|---|
Bubble oxygenators | Atherosclerotic debris | Fibrin |
Air entry into the circuit | Fat, fat droplets | Free fat |
Residual air in the heart | Fibrin clot | Aggregated chylomicrons |
Loose purse-string sutures | Cholesterol crystals | Denatured proteins |
Cardiotomy reservoir | Calcium particles | Platelet aggregates |
Rapid rewarming | Muscle fragments | Platelet-leukocyte aggregates |
Cavitation | Tubing debris, dust | Hemolyzed red cells |
Bone wax, talc | Transfused blood | |
Silicone antifoam | ||
Glue, Surgicel | ||
Cotton sponge fiber |
The brain receives 14% of the cardiac output and is the most sensitive organ for microembolic injury.83 Strategies to selectively reduce microembolism to the brain include reducing PaCO2 to cause cerebral vasoconstriction84; hypothermia85; placing aortic cannulas downstream to the cerebral vessel36,74; and using special aortic cannulas with22,23,30 or without19 special baffles or screens designed to prevent cannula-produced cerebral atherosclerotic emboli.
Two types of blood microfilters are available for use within the perfusion circuit: depth and screen.86,87 Depth filters consist of packed fibers or porous foam, have no defined pore size, present a large, tortuous, wetted surface, and remove microemboli by impaction and absorption. Screen filters are usually made of woven polyester or nylon thread, have a defined pore size, and filter by interception. Screen filters vary in pore size and configuration and block most air emboli; however, as pore size decreases, resistance increases. As compared with no filter, studies indicate that all commercial filters effectively remove gaseous and particulate emboli.88,89 Most investigations find that the Dacron wool depth filter is most effective, particularly in removing microscopic and macroscopic air. Pressure differences across filters vary between 24 and 36 mm Hg at 5 L/min flow. Filters cause slight hemolysis and tend to trap some platelets; nylon filters may also activate complement.86
The need for microfilters in the cardiotomy suction reservoir is universally accepted,77 and most commercial units contain an integrated micropore filter. The need for a filter in the cardioplegia delivery system, however, remains debatable,90 and although almost always used, the requirement of an arterial line filter is unsettled.87 In vitro studies demonstrate that an arterial filter reduces circulating microemboli89 and clinical studies are confirmatory.89 However, these filters do not remove all microemboli generated by the extracorporeal circuit.12,74,77 When bubble oxygenators are used, studies show equivocal or modest reductions in microemboli55,91 and neurologic outcome markers.91 In contrast, membrane oxygenators produce far fewer microemboli and when used without an arterial filter, the numbers of microemboli are similar to those found with bubble oxygenators plus arterial line filters.87
Although the efficacy of arterial line microfilters remains unsettled, their use is almost universal92; and although they are effective bubble traps, they do increase cost, occasionally obstruct during use, are difficult to de-air during priming, and require a small bypass line and valved purge line to remove any air.
Other sources of biologic microemboli may be more important. Cerebral microemboli are most numerous during aortic cannulation,93,94 application and release of aortic clamps,94 and at the beginning of cardiac ejection after open heart procedures.95 Furthermore, as compared with perfusion microemboli, surgically induced emboli are more likely to cause postoperative neurologic deficits.96
Leukocyte-depleting filters are discussed later in this chapter and have been recently reviewed.97 These filters reduce circulating leukocyte counts in most studies,98 but fail to produce convincing evidence of clinical benefit.99
The various components of the heart-lung machine are connected by polyvinyl tubing and fluted polycarbonate connectors. Medical grade polyvinyl chloride (PVC) tubing is universally used because it is flexible, compatible with blood, inert, nontoxic, smooth, nonwettable, tough, transparent, resistant to kinking and collapse, and can be heat sterilized. To reduce priming volume, tubing connections should be short. To reduce turbulence, cavitation, and stagnant areas, the flow path should be smooth and uniform without areas of constriction or expansion. Wide tubing improves rheology, but also increases priming volume. In practice 1/2- to 5/8-inch (internal diameter) tubing is used for most adults, but until a compact, integrated, complete heart-lung machine can be designed and produced as a unit, the flow path will produce some turbulence. Loose tubing connections can be sources of air intake or blood leakage and so all connections must be secure. For convenience and safety, most tubing and connectors are prepackaged and disposable.
Heparin can be bound to blood surfaces of all components of the extracorporeal circuit by ionic or covalent bonds. The Duraflo II heparin coating ionically attaches heparin to a quaternary ammonium carrier (alkylbenzyl dimethyl-ammonium chloride), which binds to plastic surfaces (Edwards Lifesciences, Irvine, CA). Covalent attachment is produced by first depositing a polyethylenimine polymer spacer onto the plastic surface, to which heparin fragments bind (Carmeda Bioactive Surface, Medtronic, Inc., Minneapolis, MN). Ionic-bound heparin slowly leaches, but this is irrelevant in clinical cardiac surgery. The use of heparin-coated circuits during CPB has spawned an enormous literature100-102 and remains controversial largely because studies are contaminated by patient selection, reduced doses of systemic heparin, and washing or discarding field-aspirated blood.102 To date, there is no irrefutable and credible evidence that heparin-coated perfusion circuits reduce the need for systemic heparin or reduce bleeding or thrombotic problems associated with CPB. Although the majority of studies indicate that heparin coatings reduce concentrations of C3a and C5b-9,103 the inflammatory response to CPB is not reduced and the evidence for clinical benefit is not convincing.104
Other more recently developed and available surface modifications and coatings101 include a phosphorylcholine coating,105 surface-modifying additives,106 and a trillium biopassive surface107 as well as other proprietary substances. While many in vitro and even in vivo studies imply multiple benefits from these coatings by reductions in various inflammatory mediators and in the stimulation of the coagulation cascade, there have been no blinded randomized trials demonstrating any measurable benefit in patient outcomes. Clearly additional investigation in this area is desirable.
Blood aspirated from the surgical wound may be directed to the cardiotomy reservoir for defoaming, filtration, and storage before it is added directly to the perfusate. A sponge impregnated with a surfactant removes bubbles by reducing surface tension at the blood interface and macro, micro, or combined filters remove particulate emboli. Negative pressure is generated by either a roller pump or vacuum applied to the rigid outer shell of the reservoir. The degree of negative pressure and blood level must be monitored to avoid excessive suction or introducing air into the perfusate.
The cardiotomy suction and reservoir are major sources of hemolysis, particulate and gaseous microemboli, fat globules, cellular aggregates, platelet injury and loss, thrombin generation, and fibrinolysis.73,77,108 Air aspirated with wound blood contributes to blood activation and destruction and is difficult to remove because of the high proportion of nitrogen, which is poorly soluble in blood. High suction volumes and admixture of air are particularly destructive of platelets and red cells.108 Commercial reservoirs are designed to minimize air entrainment and excessive injury to blood elements. Air and microemboli removal are also facilitated by allowing aspirated blood to settle within the reservoir before it is added to the perfusate.
An alternative method for recovering field-aspirated blood is to dilute the blood with saline and then remove the saline to return only packed red cells to the perfusate. Two types of centrifugal cell washers automate the process. Intermittent centrifugation (eg, Haemonetics Cell Saver, Meomonetics Corp., Braintree, MA) removes air, thrombin, and many biologic and nonbiologic microemboli from the aspirate at the cost of discarding plasma. Continuous centrifugation (eg, Fresenius/Terumo CATS, Elkton, MD) in addition removes fat and activated leukocytes.109 A third alternative is to discard all field-aspirated blood, although most surgeons would find this practice unacceptable if it increased allogeneic blood transfusion. Increasingly, field-aspirated blood is recognized as a major contributor to the thrombotic, bleeding, and inflammatory complications of CPB.
If the heart is unable to contract, distention of either ventricle is detrimental to subsequent contractility.110 Right ventricular distention during cardiac arrest or ventricular fibrillation is rarely a problem, but left ventricular distention can be insidious in that blood can enter the flaccid, thick-walled chamber from multiple sources during this period. During CPB, blood escaping atrial or venous cannulas and from the coronary sinus and thebesian veins may pass through the unopened right heart into the pulmonary circulation. This blood plus bronchial arterial and venous blood, blood regurgitating through the aortic valve, and blood from undiagnosed abnormal sources (patent foramen ovale, patent ductus, etc.) may distend the left ventricle unless a vent catheter is used (Fig. 13-6). During CPB bronchial blood and noncoronary collateral flow average approximately 140 ± 182 and 48 ± 74 mL/min, respectively.111
FIGURE 13-6
Diagram shows locations used to vent (decompress the heart). (A) Aortic root vent, which can also be used to administer cardioplegic solution after the ascending aorta is clamped. (B) A catheter placed in the right superior pulmonary vein/left atrial junction can be passed through the mitral valve into the left ventricle. (C) Direct venting of the left ventricle at the apex. (D) Venting the main pulmonary artery, which decompresses the left atrium because pulmonary veins lack valves.

There are several methods for venting the left heart during cardiac arrest. Although it was used commonly in the past, few surgeons in the modern era vent the left ventricular apex directly because of inconvenience and myocardial injury. Most often a multihole, soft-tip catheter (8-10 French) is inserted into the junction of the right superior pulmonary vein and left atrium (see Fig. 13-6) or left atrial appendage and may or may not be passed into the left ventricle. Others prefer to place a small suction catheter into the pulmonary artery.112 The ventricle can also be vented by passing a catheter retrograde across the aortic valve when working on the mitral valve. Vent catheters are drained to the cardiotomy reservoir by a roller pump, vacuum source, or gravity drainage,113 but must be carefully monitored for malfunction. If connected to a roller pump, the system should be carefully tested before use to ensure proper operation. Although inspection and palpation may detect ventricular distention, TEE monitoring or direct measurements of left atrial or pulmonary arterial pressures are more reliable. The heart is no longer vented for most myocardial revascularization operations, but the ventricle must be protected from distention.114 If the heart cannot remain decompressed during distal anastomoses, a vent should be inserted. Often the cardioplegia line inserted into the aortic root is used for venting when not used for cardioplegia.115
The most common and serious complication of left heart venting is residual air when the heart is filled and begins to contract. De-airing maneuvers and TEE are important methods for ensuring removal of all residual air. In addition, many surgeons aspirate the ascending aorta via a small metal or plastic cannula to detect and remove any escaping air as the heart begins to eject.116 Bleeding, atrial perforation, mitral valve injury, and direct injury to the myocardium are other complications associated with left ventricular vents.
Cardioplegic solutions contain 8 to 20 mEq/L potassium, magnesium, and often other components that are infused into the aortic root proximal to the aortic cross clamp, or retrograde into the coronary sinus to arrest the heart in diastole. The carrier may be crystalloid or blood and is infused at temperatures around 4 or 37°C, depending upon surgeon’s preference. Normothermic cardioplegia must be delivered almost continuously to keep the heart arrested while cold cardioplegia may be infused intermittently. Cardioplegic solutions are delivered through a separate perfusion system that includes a reservoir, heat exchanger, roller pump, bubble trap, and perhaps microfilter (see Fig. 13-2). Temperature and infusion pressure are monitored. The system may be completely independent of the main perfusion circuit or it may branch from the arterial line. The system also may be configured to vent the aortic root when not delivering cardioplegia.
Antegrade cardioplegia is delivered through a small cannula in the aortic root or via cannulas directly into the coronary ostia when the aortic valve is exposed. Retrograde cardioplegia is delivered through a cuffed catheter inserted into the coronary sinus.117 Proper placement of the retrograde catheter is critical, but not difficult, and is verified by palpation, TEE, color of the aspirated blood, or pressure waveform of a catheter pressure sensor.118 Complications of retrograde cardioplegia include rupture or perforation of the sinus, hematoma, and rupture of the catheter cuff.119
Hemoconcentrators, like oxygenators, contain one of several available semipermeable membranes (typically hollow fibers) that transfer water, electrolytes (eg, potassium), and molecules up to 20 kDa out of the blood compartment.120 Hemoconcentrators may be connected to either venous or arterial lines or a reservoir in the main perfusion circuit, but require high pressure in the blood compartment to effect fluid removal. Thus a roller pump is needed unless connected to the arterial line. Suction may or may not be applied to the air side of the membrane to facilitate filtration. Up to 180 mL/min of fluid can be removed at flows of 500 mL/min.121 Hemoconcentrators conserve platelets and most plasma proteins as compared with centrifugal cell washers, and may allow greater control of potassium concentrations than diuretics.122 Aside from cost, disadvantages are few and adverse effects are rare.121
Table 13-3 lists monitors and safety devices that are commonly used during CPB. Pressure in the arterial line between pump and arterial line filter is monitored continuously to instantly detect any increased resistance to arterial inflow into the patient. This pressure should be higher than radial arterial pressure because of resistance of the filter (if used) and cannula. The arterial pressure monitor may be connected to an audible alarm or the pump switch to alert the perfusionist to dangerous increases in the arterial line pressure.
Device or procedure |
---|
Low venous blood level alarm with pump cut-off |
High arterial line pressure alarm with pump cut-off |
Macrobubble detector with pump cut-off |
Arterial line filter |
Prebypass recirculation/filtration |
Oxygen supply filter |
In-line venous oxygen saturation |
In-line arterial oxygen saturation |
Oxygenator gas supply oxygen analyzer |
One-way valved intracardiac vent lines |
Batteries in heart-lung machine |
Alternate dedicated power supply |
Electrical generator |
Back-up arterial pump head |
Back-up heater-cooler |
Back-up oxygen supply |
Emergency lighting |
Prebypass activated clotting time |
Activated clotting time during cardiopulmonary bypass |
Prebypass check list |
Written protocols |
Log of perfusion incidents |
Log of device failures |
An arterial line flowmeter is essential for centrifugal pumps and may be desirable to confirm flow calculations with roller pumps as well.
In-line devices are available to continuously measure blood gases, hemoglobin/hematocrit, and some electrolytes.123 Placed within the venous line, these devices permit continuous assessment of oxygen supply and demand.124 In the arterial line the devices offer better control of blood gases.125 The need for these devices is unproved and because reliability is still uncertain, use may distract operative personnel and spawn unnecessary laboratory measurements.126 The use of automated analyzers by the perfusion team in the operating room is an alternative if frequent measurement of blood gases, hematocrit, and electrolytes is desirable.123
The flow and concentration of oxygen entering the oxygenator should be monitored.127 Some teams also monitor exit gases to indirectly estimate metabolic activity and depth of anesthesia.127 Some manufacturers recommend monitoring the pressure gradient across membrane oxygenators, which may be an early indication of oxygenator failure, although it is a rare event.62-64
Temperatures of the water entering heat exchangers must be monitored and carefully controlled to prevent blood protein denaturation and gaseous microemboli.65 During operations using deep hypothermia, changes in venous line temperatures reflect rates of temperature change in the patient, and monitoring arterial inflow temperature helps prevent brain hyperthermia during rewarming.
A low-level sensor with alarms on the venous reservoir and a bubble detector on the arterial line are additional safety devices sometimes used. A one-way valve is recommended in the purge line between an arterial filter/bubble trap and cardiotomy reservoir to prevent air embolism. Ultrasound transducers imbedded in the arterial perfusion tubing distal to the filter are now available to monitor low-level air entry into the circulation. Valves in the venous and vent lines protect against retrograde air entry into the circulation or in the arterial line to prevent inadvertent exsanguination.68
Automatic data collection systems are available for preoperative calculations and to process and store data during CPB.128 Computer systems for operating CPB are in development.129
Although the surgeon is directly responsible for the outcome of the operation, he or she needs a close working relationship with both the anesthesiologist and the perfusionist. These three principals must communicate freely, often, and candidly. Their overlapping and independent responsibilities relevant to CPB are best defined by written policies that include protocols for various types of operations and emergencies and by periodic multidisciplinary conferences. This teamwork is not unlike the communication advocated for the cockpit crew of commercial and military aircraft.
The surgeon determines the planned operation, target perfusion temperatures, methods of cardioplegia, cannulations, and anticipated special procedures. During operation the surgeon communicates the procedural steps involved in connecting and disconnecting the patient to CPB and interacts with the other principals to coordinate perfusion management with surgical exposure and working conditions. The perfusionist is responsible for setting up and priming the heart-lung machine, performing safety checks, operating the heart-lung machine, monitoring the conduct of bypass, monitoring anticoagulation, adding prescribed drugs, and maintaining a written perfusion record.
The anesthesiologist monitors the operative field, anesthetic state and ventilation of the patient, the patient’s physiology, and conduct of perfusion. A vigilant anesthesiologist is the safety officer and often “troubleshooter” of these complex procedures and along with the surgeon is in the best position to anticipate, detect, and correct deviations from desired conditions. In addition the anesthesiologist provides TEE observations before, during, and immediately after bypass.
The perfusionist is responsible for setting up and preparing the heart-lung machine and all associated components necessary for the proposed operation. Most perfusionists use commercial, sterile, preprepared customized tubing packs that are connected to the various components that constitute the heart-lung machine. This dry assembly takes about 10 to 15 minutes, and a dry system can be kept in standby for up to 7 days. Once the system is primed with fluid, which takes about 15 minutes, it should be used within 8 hours to prevent malfunction of the oxygenator. After assembly, the perfusionist conducts a safety inspection and completes a written prebypass checklist.
Traditional adult ECP circuits require 1.5 to 2.0 L of balanced electrolyte solution such as lactated Ringer’s solution, Normosol-A, or Plasma-Lyte. Before connections are made to the patient, the prime is recirculated through a micropore filter to remove any residual particulate matter or air. In the average-sized adult, the priming volume represents approximately 30 to 35% of the patient’s blood volume and reduces the hematocrit to about two-thirds of the preoperative value. In smaller patients or in the presence of peroperative anemia, banked blood may be added to the prime volume to raise the hematocrit to a predetermined minimum (eg, 25% or more), to achieve an acceptable resultant hemotocrit once CPB has been initiated. There is no consensus regarding the optimal hematocrit during CPB; most perfusates have hematocrits between 20% and 25% when used with moderate hypothermia (25-32°C). Dilution reduces perfusate viscosity, which is not a problem during clinical CPB, but also reduces oxygen-carrying capacity; mixed venous oxygen saturations less than 60% usually prompt either transfusion or increased pump flow.124 Sometimes 12.5 to 50 g of mannitol is added to stimulate diuresis and possibly minimize postoperative renal dysfunction.
Efforts to avoid the use of autologous blood include reducing the priming requirement of the machine by using smaller diameter and shorter tubing lengths and operating the machine with minimal perfusate in the venous and cardiotomy reservoirs. This latter practice increases the risk of air embolism, the risk of which can be reduced by using collapsible reservoirs and reservoir level sensors that stop the pump. In recent years, smaller, more compact circuits have been designed to reduce the prime volume and subsequent hemodilution, reducing transfusion requirements and platelet consumption.130 Many of these circuits have totally removed the venous reservoir and used a variety of coated surfaces in an attempt to reduce hemodilution, avoid points of stasis and minimize activation of inflammation and coagulation cascades. A typical such mini-circuit is pictured in Fig. 13-7.
Autologous blood prime is another technique to minimize hemodilution, which displaces and then removes crystalloid prime by draining blood volume from the patient into the circuit just before beginning CPB.131 This method reduces perfusate volume, but phenylephrine may be required to maintain stable hemodynamics.131 The method reduces transfusions and does not affect clinical outcome.
The use of colloids (albumin, gelatins, dextrans, and hetastarches) in the priming volume is controversial.132 Although their use clearly minimizes the decrease in colloid osmotic pressure133 and may reduce the amount of fluid entering the extracellular space, any impact on clinical outcome remains unproved. Prospective clinical studies have failed to document significant clinical benefits with albumin,133 which is expensive and may have adverse effects.134 Hetastarch may contribute to postoperative bleeding.135 McKnight et al. found no influence of prime composition on postoperative nitrogen balance.136 Because of possible adverse effects, including neurologic deficits, the addition of glucose and/or lactate to the prime is generally avoided.137,138
Porcine heparin (300-400 units/kg IV) is administered before arterial or venous cannulas are inserted, and CPB is not started until anticoagulation is confirmed by either an activated clotting time (ACT) or the Hepcon test. Although widely used, bovine heparin is more antigenic in inducing antiplatelet IgG antibodies than is porcine heparin.139 Although the distribution of intravenously administered heparin has been shown to be extremely rapid,140 in general, the anticoagulation effect is measured about 3 minutes after heparin administration. However, groups differ in the minimum ACT that is considered safe for CPB. The generally accepted minimum for ACT before initiation of CPB is greater than or equal to 400 seconds; however, many groups recommend 480 seconds141 because heparin only partially inhibits thrombin formation during CPB. More recently, in an attempt to reduce surgical bleeding, some centers have advocated accepting lower ACTs in the 300 range. Although early unpublished results may suggest that this can be done safely, it is still not generally accepted. Outside the United States, where aprotinin is still available, it is important to measure ACT with kaolin as opposed to celite, because celite may artifactually and erroneously increases the ACT. Failure to achieve a satisfactory ACT may result from either inadequate heparin dosage or low concentrations of antithrombin. If a total of 500 units/kg of heparin fails to adequately prolong ACT, antithrombin III should be administered to the patient either as fresh-frozen plasma or as recombinant antithrombin III when available142 to increase antithrombin concentrations to overcome “heparin resistance.” Antithrombin III is a necessary cofactor that binds circulating thrombin; heparin accelerates this reaction a thousandfold. See Thrombosis and Bleeding for management of patients with suspected or proved heparin-induced antiplatelet IgG antibodies and alternative anticoagulants to heparin.
During CPB, ACT or the Hepcon test is measured every 30 minutes. If ACT goes below the target level, more heparin is given. As a general rule, one-third of the initial total heparin bolus required for adequate anticoagulation is given every hour even when the ACT is within the normal range. The Hepcon test titrates the heparin concentration and is more reproducible than ACT, but ACT provides satisfactory monitoring of anticoagulation. Although excessively high concentrations of heparin (ACT > 1000 seconds) may cause remote bleeding away from operative sites, low concentrations increase circulating thrombin concentrations and risk clotting within the ECP circuit.
After the patient has successfully weaned from CPB and remains stable, 1 mg of protamine is given for each 100 units of heparin given in the initial bolus dose, but not to exceed 3 mg/kg. The heparin-protamine complex activates complement and may causes acute hypotension, which may be attenuated by the administration of calcium (2 mg/1 mg protamine). Once the administration of protamine has begun, it is generally recommended that the use of cardiotomy suction into the reservoir is discontinued because of the risk of generating clot within the circuit and losing the potential for emergency support should the patient become unstable. Rarely, protamine may cause an anaphylactic reaction in patients with antibodies to protamine insulin.143 This severe reaction may require urgently placing the patient back on CPB, although it may also be treating with resuscitation using epinephrine and immediate discontinuation of the protamine infusion. Neutralization of heparin is usually confirmed by an ACT or Hepcon test and more protamine (50 mg) is given if either test remains prolonged or bleeding is a problem. Heparin rebound is a term used to describe a delayed heparin effect because of release of tissue heparin after protamine is cleared from the circulation, particularly from heparin deposited in adipose tissues, and seen more often in obese patients. Although protamine is a mild anticoagulant at higher doses, one or two supplemental 25- to 50-mg doses can be given empirically if heparin rebound is suspected, or if the ACT remains elevated. It is also noted that the ACT can be elevated in the presence of significant thrombocytopenia, despite the full reversal of heparin. As a rule, the heart-lung machine should be available for unexpected decompensation and the need for urgent return to CPB until the wound is closed, the drapes are removed, and at many centers until the patient leaves the operating room.
Once the appropriate cannulation has occurred and adequate anticoagulation has been confirmed, CPB is initiated at the surgeon’s request with concurrence of the anesthesiologist and perfusionist. As the venous return enters the machine, the perfusionist progressively increases arterial flow while monitoring the patient’s blood pressure and volume levels in all reservoirs. Six observations are critical:
The venous drainage is adequate for the desired flow
The pressure in the arterial line is acceptable
The arterial blood is adequately oxygenated
The systemic arterial pressure is acceptable
The systemic venous pressure is acceptable
The heart is adequately decompressed
Once full stable CPB is established for at least 2 minutes, lung ventilation is discontinued, perfusion cooling may begin, and the aorta may be clamped for arresting the heart. Just as is seen with initiation of dialysis, it is not uncommon to see some vasodilation and early hypotension as the patient is first exposed to the artificial surfaces, particularly the oxygenator. This can usually be managed by the perfusionist with increased flows until the vasodilation resolves, although occasionally vasopressors such as neosynephrine may be transiently required.
Antegrade blood or crystalloid cardioplegia is administered directly into the aortic root at 60 to 100 mm Hg pressure proximal to the aortic cross-clamp by a dedicated cardioplegia roller pump (see Fig. 13-2). Blood entering the right atrium from the coronary sinus is captured by the right atrial or unsnared caval catheters. If the caval snares are tightened, the right atrium should be vented to prevent right ventricular distention. Many surgeons choose to monitor myocardial temperature and administer cardioplegia to cool the myocardium to a specific temperature range. Others deliver a specific amount of cardioplegia, or monitor the electrical activity to determine the delivered volume. With appropriate delivery of antegrade cardioplegia, the heart should usually arrest within 30 to 60 seconds, and failure to do so may indicate problems with delivery of the solution or unrecognized aortic regurgitation. Some surgeons monitor myocardial temperature or pH via direct needle sensors.144
The usual flow of retrograde cardioplegia is 200 to 400 mL/min at coronary sinus pressures between 30 and 50 mm Hg.145 Higher pressures may injure the coronary venous system119; low pressures usually indicate inadequate delivery owing to malposition of the catheter or leakage around the catheter cuff, but may also indicate a tear in the coronary sinus. Induction of electrical arrest is slower (2-4 minutes) than with antegrade delivery, and retrograde cardioplegia may provide incomplete protection of the right ventricle.117
The following offers rational guidelines for management of CPB, which uses manipulation of temperature, hematocrit, pressure, and flow rate to adequately support cellular metabolism during nonphysiologic conditions.
Under normal circumstances, basal cardiac output is determined by oxygen consumption, which is approximately 250 mL/min. It is impractical to measure oxygen consumption while on CPB, so a generally accepted flow rate at 35 to 37°C with a hematocrit of 25% is approximately 2.4 L/min/m2 in deeply anesthetized and muscle-relaxed patients. Hemodilution reduces blood oxygen content from approximately 20 to 10 to 12 mL/dL; consequently, flow rate must increase over resting normal cardiac output or oxygen demand must decrease. The resistance of venous catheters, turbulence, and loss of physiologic controls of the vasculature may also effect venous return and limit maximum pump flow.
Hypothermia reduces oxygen consumption by a factor of 0.5 for every 10°C decrease in temperature. However, at both normothermia and hypothermia maximal oxygen consumption falls with decreasing flow as described in the following equation:
This relationship at various temperatures is depicted in Figure 13-8. For this reason Kirklin and Barratt-Boyes146 recommend that flows be reduced only to levels that permit at least 85% of maximal oxygen consumption. At 30°C this flow rate in adults is approximately 1.8 L/min/m2; at 25°C, 1.6 L/min/m2; and at 18°C, 1.0 L/min/m2.
As long as mean arterial pressure remains above 50 to 60 mm Hg (ie, above the autoregulatory range), cerebral blood flow is preserved even if systemic flow is less than normal. However, there is a hierarchal reduction of flow to other organs as total systemic flow is progressively reduced. First skeletal muscle flow falls, then abdominal viscera and bowel, and finally renal blood flow.
Theoretical benefits of pulsatile blood flow include increased transmission of energy to the microcirculation, which reduces critical capillary closing pressure, augments lymph flow, and improves tissue perfusion and cellular metabolism. Theoretically, pulsatile flow reduces vasocontrictive reflexes and neuroendocrine responses and may increase oxygen consumption, reduce acidosis, and improve organ perfusion. However, despite extensive investigation no one has convincingly demonstrated a benefit of pulsatile blood flow over nonpulsatile blood flow for short- or long-term CPB.71,147 Two studies reported the association of pulsatile flow with lower rates of mortality, myocardial infarction, and low cardiac output syndrome,148 but others failed to detect clinical benefits.149
Pulsatile CPB can reproduce the normal pulse pressure within the body, but is expensive, complicated, and requires a large-diameter aortic cannula. Higher nozzle velocities increase trauma to blood elements,150 and pulsations may damage micromembrane oxygenators.151 Thus for clinical CPB, nonpulsatile blood flow is an acceptable, nonphysiologic compromise with few disadvantages.
Systemic arterial blood pressure is a function of flow rate, blood viscosity (hematocrit), and vascular tone. Perfusion of the brain is normally protected by autoregulation, but autoregulation appears to be lost somewhere between 55 and 60 mm Hg during CPB at moderate hypothermia and a hematocrit of 24%.84,152 Cerebral blood flow may still be adequate at lower arterial pressures,153 but the only prospective randomized study found a lower combined major morbidity/mortality rate when mean arterial pressure was maintained near 70 mm Hg (average 69 ± 7) rather than below 60 (average 52).154 In older patients, who may have vascular disease155 and/or hypertension, mean arterial blood pressure is generally maintained between 70 and 80 mm Hg at 37°C. Higher pressures are undesirable because collateral blood flow to the heart and lungs increases blood in the operative field.
Hypotension during CPB may be the result of low pump flow, aortic dissection, measurement error, or vasodilatation. Phenylephrine is most often used to elevate blood pressure, but arginine vasopressin (0.05-0.1 unit/min) has more recently been introduced. If anesthesia is adequate, hypertension can be treated with nitroprusside, an arterial dilator, or nitroglycerin, which predominantly dilates veins and pulmonary vessels.
The ideal hematocrit during CPB remains controversial. Low hematocrits reduce blood viscosity and hemolysis, reduce oxygen-carrying capacity, and reduce the need for autologous blood transfusion. In general, viscosity remains stable when percent hematocrit and blood temperature (in degrees Celsius) are equal (ie, viscosity is constant at hematocrit 37%, temperature 37°C, or at hematocrit 20%, temperature 20°C). Hypothermia reduces oxygen consumption and permits perfusion at 26 to 28°C with hematocrits between 18% and 22%, but at higher temperatures limits on pump flow may not satisfy oxygen demand.156,157 Hill158 found that hematocrit during CPB did not affect either hospital mortality or neurologic outcome, but DeFoe observed159 increasing hospital mortality with hematocrits below 23% during CPB; thus the issue remains unresolved.160 However, higher hematocrits (25-30%) during CPB appear justified157 in view of the increasing safety of autologous blood transfusion, improved neurologic outcomes with higher hematocrits in infant cardiac surgery,161 and more frequent operations near normothermia in older sicker patients.
The ideal temperature for uncomplicated adult cardiac surgery is also an unsettled question.157 Until recently, nearly all operations reduced body temperature to 25 to 30°C during CPB to protect the brain, support hypothermic cardioplegia, permit perfusion at lower flows and hematocrits, and increase the safe duration of circulatory arrest in case of emergency. Hypothermia, however, interferes with enzyme and organ function, aggravates bleeding, increases systemic vascular resistance, delays cardiac recovery, lengthens duration of bypass, increases the risk of cerebral hyperthermia, and is associated with higher levels of depression and anxiety postoperatively.162 Because the embolic risk of cerebral injury often is greater than perfusion risk, perfusion at higher temperatures (33-35°C), or “tepid” CPB, is recommended, in part because detrimental high blood temperatures are avoided during rewarming.163 Increasingly, efforts are made to avoid cerebral hyperthermia during and after operation, and one study suggests improved neuropsychometric outcomes if patients are rewarmed to only 34°C.164
There are two strategies for managing pH/PCO2 during hypothermia: pH stat and alpha stat. During deep hypothermia and circulatory arrest (see the following) there is increasing evidence that pH-stat management may produce better neurologic outcomes during pediatric cardiac surgery.161 Alpha stat may be better in adults.165 pH stat maintains temperature-corrected pH 7.40 at all temperatures and requires the addition of CO2 as the patient is cooled. Alpha stat allows the pH to increase during cooling so that blood becomes alkalotic. Cerebral blood flow is higher, and pressure is passive and uncoupled from cerebral oxygen demand with pH stat. With alpha stat, cerebral blood flow is lower, autoregulated, and coupled to cerebral oxygen demand.166
PaO2 should probably be kept above 150 mm Hg to assure complete arterial saturation. Whether or not high levels (ie, >200 mm Hg) are detrimental has not yet been determined.
Although Hill158 found no relationship between blood glucose concentrations during CPB and adverse neurologic outcome, others have been concerned that hyperglycemia (>180 mg/dL) aggravates neurologic injury138 and other morbidity/mortality,167 and recently many studies have documented the importance of tight glucose control in the prevention of infection, neurologic injury, renal and cardiac complications, as well as a reduction in ICU length of stay and overall mortality.168
Systemic arterial pressure is typically monitored by radial, brachial, or femoral arterial catheter. Central venous pressure is routinely monitored by a jugular venous catheter. Routine use of a Swan-Ganz pulmonary arterial catheter is controversial and not necessary for uncomplicated operations in low-risk patients.169 During CPB the pulmonary artery catheter should be withdrawn into the main pulmonary artery to prevent lung perforation and suture ensnarement.
A comprehensive TEE examination170 is an important monitor during most applications of CPB171 to assess catheter and vent insertion and location117,172,173; severity of regional atherosclerosis33; myocardial injury, infarction, dilatation, contractility, thrombi, and residual air; undiagnosed anatomic abnormalities170; valve function after repair or replacement; diagnosis of dissection41,174; and adequacy of de-airing at the end of CPB.175
Bladder or rectal temperature is usually used to estimate temperature of the main body mass, but does not reflect brain temperature.176 Esophageal and pulmonary artery temperatures may be affected by local cooling associated with cardioplegia. The jugular venous bulb temperature is considered the best surrogate for brain temperature, but is more cumbersome to obtain.177 Nasopharyngeal or tympanic membrane temperatures are more commonly used, but tend to underestimate jugular venous bulb temperature during rewarming by 3 to 5°C.178 During rewarming, arterial line temperature correlates best with jugular venous bulb temperature.179
Neurophysiologic monitoring during CPB is becoming more commonly used in both adult and pediatric perfusion, particularly in preparation for deep hypothermic circulatory arrest. However, its full efficacy and impact on outcomes has not yet been fully established as a necessary component of CPB, and remains under investigation. Techniques being investigated include jugular venous bulb temperature and saturation, transcranial Doppler ultrasound, near-infrared transcranial reflectance spectroscopy (NIRS), and the raw or processed electroencephalogram (EEG).180
During CPB oxygen consumption (VO2) equals pump flow rate multiplied by the difference in arterial (CaO2) and venous oxygen content (CVO2). For a given temperature, maintaining VO2 at 85% predicted maximum during CPB assures adequate oxygen delivery (see Fig. 13-8).146 Oxygen delivery (DO2) equals pump flow multiplied by CaO2 and should be greater than 250 mL/min/m2 during normothermic perfusion.156 Mixed venous oxygen saturation (SVO2) assesses the relationship between DO2 and VO2; values less than 60% indicate inadequate oxygen delivery. Because of differences in regional vascular tone, higher SVO2 does not assure adequate oxygen delivery to all vascular beds.181 Metabolic acidosis (base deficit) or elevated lactic acid levels may indicate inadequate perfusion, even in the face of “normal” SVO2 measurements.
Urine output is usually monitored but varies with renal perfusion, temperature, composition of the pump prime, diuretics, absence of pulsatility, and hemoconcentration. Urine production is reassuring during CPB and oliguria requires investigation.
These Doppler and laser measurements gauge splanchnic perfusion but are rarely used clinically.
Before stopping CPB the patient is rewarmed to 34 to 36°C, the heart is defibrillated if necessary, and the lungs are reexpanded and ventilated. The cardiac rhythm is monitored, and hematocrit, blood gases, acid-base status, and plasma electrolytes are reviewed. If the heart has been opened, TEE is recommended for detection and removal of trapped air before the heart is allowed to eject. Caval catheters are adjusted to ensure unobstructed venous return to the heart. If the need for inotropic drugs is anticipated, these are started at low flow rates. Vent catheters are removed, although sometimes an aortic root vent is placed on gentle suction to remove undiscovered air.
Once preparations are completed, the surgeon, anesthesiologist, and perfusionist begin to wean the patient off CPB. The perfusionist gradually occludes the venous line to allow filling of the right heart and ejection through the lungs to the left side, while simultaneously reducing pump input as cardiac rate and rhythm, arterial pressure and pulse, and central venous pressure are monitored and adjusted. Initially blood volume within the pump is kept constant, but as pump flow approaches zero, volume is added or removed from the patient to produce arterial and venous pressures within the physiologic ranges. During weaning, cardiac filling and contractility is often monitored by TEE, and intracardiac repairs and regional myocardial contractility are assessed. Pulse oximetry saturation near 100%, end-tidal CO2 greater than 25 mm Hg, and mixed venous oxygen saturation higher than 65% confirm satisfactory ventilation and circulation. When cardiac performance is satisfactory and stable, all catheters and cannulas are removed, protamine is given to reverse the heparin, and blood return from the surgical field is discontinued.
Once the patient is hemodynamically stable, as determined by surgeon and anesthesiologist, and after starting wound closure, the perfusate may be returned to the patient in several ways. The entire perfusate may be washed and returned as packed cells, excess fluid may be removed by a hemoconcentrator, or the perfusate, which still contains heparin, can be gradually pumped into the patient for hemoconcentration by the kidneys. Occasionally some of the perfusate must be bagged and given later. The heart-lung machine should not be completely disassembled until the chest is closed and the patient is ready to leave the operating room.
Reoperations, surgery of the descending thoracic aorta, and minimally invasive procedures may be facilitated by surgical incisions other than midline sternotomy. These alternative incisions often require alternative methods for connecting the patient to the heart-lung machine. Some alternative applications of CPB are presented in the following.
Anterolateral incisions through the fourth or fifth interspaces provide easy access to the cavae and right atrium, adequate access to the ascending aorta, as well as exposure to the left atrium and mitral valve, but no direct access to the left ventricle. Adequate exposure of the ascending aorta is available for cross-clamping, aortotomy, and administration of cardioplegia by retracting the right atrial appendage. De-airing the left ventricle (eg, after mitral valve repair) is more difficult. External pads facilitate defibrillation.
Lateral or posterolateral incisions in the left chest are used for a variety of operations. Venous return may be captured by cannulating the pulmonary artery via a stab wound in the right ventricle, or by retrograde cannulation of the left pulmonary artery or cannulation of the left iliac or femoral vein. With iliac or femoral cannulation, venous return may be augmented by threading the cannula into the right atrium using TEE guidance.182 The descending thoracic aorta or left subclavian, iliac, or femoral arteries are accessible for arterial cannulation.
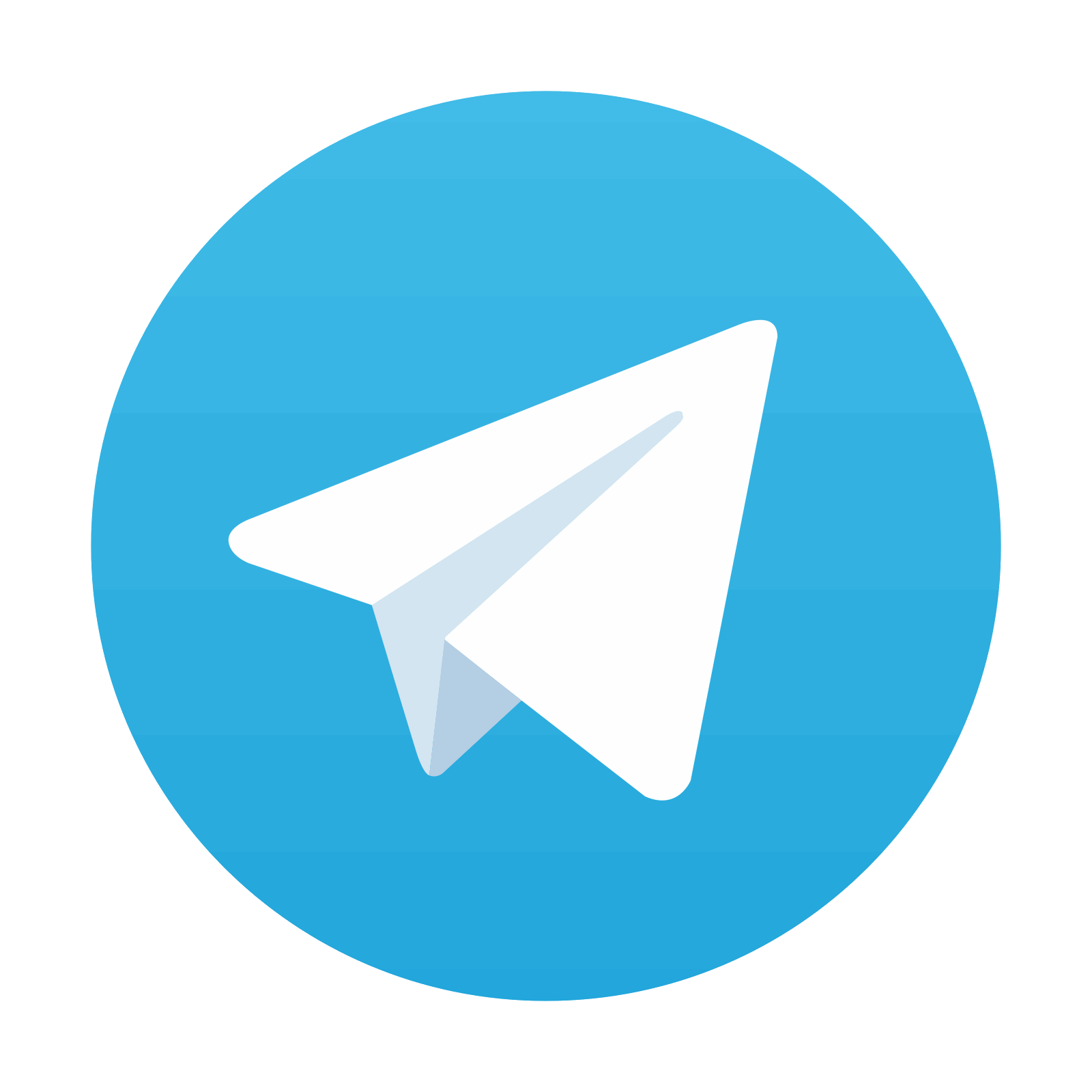
Stay updated, free articles. Join our Telegram channel

Full access? Get Clinical Tree
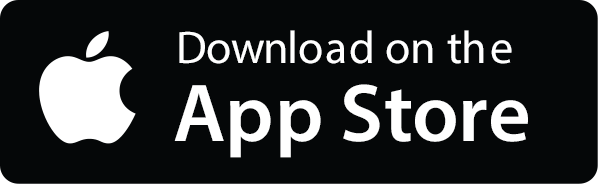
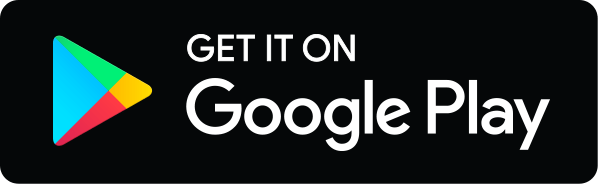