(1)
Institute of Pathology, Medical University Graz, Graz, Austria
22.1 History
Mice, rats, and hamsters have been used as models for human lung cancer since several decades [1, 2]. In the past, mainly inhalation of tobacco smoke and other carcinogenic substances has been applied to these rodent species [3–6]. In most instances, lung carcinomas resembling the human counterpart could not be simulated with the exception of urethane-induced murine models. Moreover, rodents easily and spontaneously develop adenomas but not invasive carcinomas [7]. A cystic squamous lesion was induced in some experiments, which has been labeled as squamous cell carcinoma; however, this represents in essence bronchiectasis with squamous metaplasia and dysplasia, but never progressed into invasive squamous cell carcinoma [8, 9]. In recent time, genetically engineered lung carcinomas in mice became a tool to study carcinogenesis in adenocarcinomas and small-cell carcinomas. In this chapter, we will discuss progress as well as drawback by these different approaches in understanding human pulmonary carcinogenesis.
22.2 Tobacco Inhalation Experiments
In the 1950s and 1960s, many experimental tobacco inhalation studies were performed, some of them funded by the tobacco industry. In these experiments, mice and rats were exposed to tobacco smoke using standardized cigarettes. Inhalation was most often done by nose-only exposure: mice or rats were kept in an inhalation device with their head fixed and had to breathe through their nose tobacco smoke-enriched air for several hours. In other experiments, rats and mice were exposed to tobacco smoke through a tracheal tube inserted surgically into their trachea, thus circumventing breathing through their nose. In these experiments, pulmonary adenomas and adenocarcinomas developed, in rare instances also cystic bronchiectasis with squamous metaplasia and focal dysplasia. The results of these experiments were taken as a proof that tobacco smoking does not cause squamous cell carcinomas nor small-cell carcinomas – at that time regarded as the smoking-induced carcinomas.
22.3 Why Adenocarcinomas in Mice and Rats?
Rodents when exposed to noxious substances in the air will recirculate the air within their large upper respiratory tract especially their large sinuses and clean the air before inhaling it into their lower respiratory tract. When exposed to tobacco smoke via the trachea, the content, including particulates, will be inhaled into the lung periphery, acting on Clara cells and peripheral precursor/stem cells. The airway branching in rodents is dichotomous, which results in an almost ideal laminar flow of the air with tobacco smoke content, in contrast to the asymmetric branching of primate and human airways, where particulates will be trapped at bifurcations by turbulences. Therefore if humans inhale tobacco smoke with particulates (filterless cigarette), these substances will primarily act on the epithelium of large airways, where a program exists for squamous metaplasia and where also precursor cells for SCLC are located – one of the reasons for the occurrence of SCC and SCLC in patients smoking filterless cigarettes. So these inhalation experiments preceded the occurrence of adenocarcinomas in human in the era of the filter cigarette.
22.4 Xenograft Transplantation of Human Carcinomas/Cell Cultures into Nude Mice
New experimental strategies are focusing on xenograft transplantation [10–13]. Human pulmonary carcinomas are transplanted into nude mice, and their behavior and biology are studied. In addition also genetically modified cell lines are also used in xenograft experiments [14–17]. This experimental design has advantages as well as disadvantages: Advantages are the tumors studied are real human tumors, which are biologically identical to the tumor in patients. Their genetic makeup is identical. The disadvantage is that there is no tumor-stroma interaction. So the mouse stroma cells do not recognize the tumor cells, neither cooperate nor act against the tumor. The same is true for the mouse immune system. Therefore the modification of the tumor induced by the stroma and immune system is missing, which makes prediction of behavior impossible.
22.5 Differences in Chemically Induced Lung Tumors Compared to Humans
The use of different carcinogenic substances to induce lung carcinomas goes back to the early 1960s [22, 23]. By using chemical carcinogens, carcinomas could be induced in laboratory animals, especially mice [22, 24]; benzo-a-pyrene was one of the early substances by which pulmonary carcinomas could be induced in different animal models [25–29]. These chemically induced lung carcinomas have regained interest in recent years [30–33]. Some of these models when an intratracheal instillation technology was used developed squamous cell and undifferentiated pulmonary carcinomas. Other substances such as urethane when injected induce adenocarcinomas. The advantage of chemically induced carcinogenesis in laboratory animals is that the chemicals induce a similar spectrum of genetic aberrations as tobacco smoking-induced carcinomas in humans. However, the genetic spectrum of aberrations is not constant.
Another problem of inducing lung tumors by chemical substances is the formation of spontaneous adenomas in mice.
22.6 The Urethane Model
In the 1980s and 1990s, lung carcinomas were induced by injections of different chemicals such as urethane. It took some time until it was discovered that the selection of the mouse strain also is important, as in some strains this did not work. In A/J strain of mice, urethane (ethyl carbamate) induced lung hyperplasia, adenoma, and adenocarcinoma. These morphologic stages correlated with sequential molecular genetic changes where KRAS mutations arose early and p53 mutations late in tumorigenesis. KRAS mutations were predominantly seen at codon 61 and p53 mutations in exons 5–8 [34]. A comparison of genetic changes between the mouse model and human tumor samples showed 409 genes common in the mouse model and in human tissues. A number of changes were recapitulated such as changes in glycolytic enzymes and cell cycle proteins. However, genes coding for angiogenesis were differently expressed. Therefore the A/J mouse-urethane model reflects several but not all molecular details of human lung AC [6]. A further study using this model in combination with tobacco smoke exposure failed. The results of this study showed no significant difference in the number of nodules and hyperplasia between a urethane-only and three urethane and cigarette smoke-exposed groups of Balb/c mice in the lung [35]. Another study compared exposure of methyl-nitrosourea (MNU) or urethane with mutated KRAS-induced lung carcinomas. Although the MNU-induced tumors carried exactly the same initiating mutation in KRAS as seen in the KRAS model (G12D), MNU tumors had 192 somatic single-nucleotide variants, compared to six in tumors from the KRAS model. But the KRAS tumors exhibited a significantly higher level of aneuploidy and copy number alterations compared with the carcinogen-induced tumors, suggesting that carcinogen-induced and genetically engineered models lead to tumor development through different routes. In addition the urethane-induced tumors carry predominantly KRAS Q61R mutations, whereas those from KRAS animals carry predominantly KRAS Q61L mutations [36]. Another aspect of urethane-induced carcinomas is the fact that besides lung carcinomas also tumors in other organs are induced.
22.7 Genetically Engineered Mouse Models of Lung Cancer
In recent times, genetically engineered mouse models, which express oncogenes or have lost tumor suppressor genes for lung cancer formation, have been developed. The genetically induced lung tumors in these models closely resemble human tumors [37–41].
Descriptions of these types of tumors have been published by experimental and veterinary pathologists, which, however, are not experienced in human pathology. On the other hand, most pulmonary pathologists have no experience with these experimental models or with differences of the architecture of the rodent compared to human lung. One of the aims therefore is to answer the question to which extent human lung cancer can be histopathologically modeled in mice.
We have already discussed the spectrum of human pulmonary carcinomas. So here we have to discuss comparative morphology in diseased and healthy lungs from mammalians, but also across the animal kingdom. To evaluate genetically engineered lung tumors in laboratory animals and to interpret them require the understanding of normal lung anatomy and histology with respect to differences and similarities in the architecture as well as the distribution of epithelial and mesenchymal cells in the human lung. For example, the delivery of carcinogens by inhalation will result in a high concentration at the bronchioloalveolar junction and in alveoli due to the dichotomous branching of the rodent lung and the almost laminar airstream.
Lung tumors in mice can be genetically induced by expression of oncogenes in pulmonary cells. A popular model is LSL-KRASG12D mice that express the KRASG12D oncogene in pulmonary cells after Adeno-Cre or Lenti-Cre inhalation [37–39, 42–45]. Oncogenes can also be stochastically expressed after Cre-mediated activation, which allows investigation of gene cooperation [46]. These efforts have resulted in the generation of adenocarcinomas, but not squamous cell carcinomas. Moreover, five laboratories have described mouse models for small-cell lung cancer that are based on double deletion of TP53/RB [43] or triple deletion of TP53/RB/PTEN tumor suppressors [47].
22.7.1 The Pulmonary Adenocarcinoma Models
Lung tumors were induced in HRAS-driven Multi-Hit mice, which allow stochastic activation of MAPK, RAL, and PI3K effector pathways [46] and LSL-KRASG12D mice harboring additional genetic modifications (knockout of RANK, apelin, or ATG5) using inhalation of Cre-expressing viruses. In a subset of HRAS-driven Multi-Hit mice, TP53 or PTEN genes were deleted. In each of the above experiments between 7 and 70 lungs, tumors developed in mouse lungs. A morphologic sequence from hyperplasia to atypical proliferation to adenoma to adenocarcinoma in situ and invasive adenocarcinoma was found.
22.7.2 Histopathology of Adenocarcinomas
In all KRAS oncogene activated mice – regardless of additional genetic alterations – hyperplasia of type II pneumocytes could be found (Fig. 22.1), in several experiments also nodular aggregates of pneumocytes (nodular hyperplasia; Fig. 22.2). These proliferations, however, never progressed into high-grade malignancies. In these proliferations, the morphologic differentiation of type II pneumocytes could be seen, sometimes also a cuboidal transformation of pneumocytes, which is well known in human lung pathology as reactive, reparative, or preneoplastic. Nuclear to cytoplasmic ratio was only slightly increased, but not that amount one will see in neoplasia.



Fig. 22.1
Diffuse hyperplasia of pneumocytes type II in the mouse, induced by expressing mutant KRAS or HRAS. H&E, ×400

Fig. 22.2
Nodular hyperplasia of pneumocytes and slit-like spaces of alveoli in some areas. H&E, ×200
KRAS as well as HRAS oncogenes induced another type of proliferation at the bronchioloalveolar junction. This type of proliferation started as an expansion of epithelial cells at the basal layer of the terminal bronchiolar epithelium (basal or reserve/stem cells). The cells were polygonal or cuboidal and had larger than normal nuclei with still regularly distributed chromatin. Nucleoli were inconspicuous; the cells looked similar to regenerating epithelia (Figs. 22.3, 22.4, and, 22.5). In some cases, atypia was seen early on, the polymorphous nuclei were enlarged, the chromatin was coarse, and nucleoli were enlarged (Fig. 22.6). This proliferation later on developed into a micropapillary epithelial proliferation and further on acquired a mesenchymal stalk with newly formed capillary loops and primitive mesenchymal stroma cells, thus presenting as a papillary preneoplasia. This proliferation extended into the peripheral alveoli, completely filling the air spaces (Fig. 22.7). Since the alveoli in mice are smaller compared to human lung, but the mouse transformed epithelial cells are not as small, these lesions often appeared solid (the ratio of cell diameter of pneumocytes II and alveolar diameter is different across the different mammalian species). Only at high magnification, a slit-like alveolar lumen could be seen. Nodules grew larger (up to 1 cm) and developed central necrosis, most probably due to hypoxia (Fig. 22.8). However, no invasion occurred at this time, but the cytological atypia qualified these lesions as an in situ adenocarcinoma: nuclear to cytoplasmic ratio was >50 %, nuclear chromatin was irregular distributed, nucleoli were increased in size, and few mitotic figures were found.







Fig. 22.3
Preneoplastic proliferation of cells at the bronchioloalveolar junction. Left normal bronchiolar epithelium (single cell row, few reserve cells). Right expansion of preneoplastic cells at the basis of a bronchioles (at least double layers of cells). H&E, ×200

Fig. 22.4
Later stage with papillary proliferations into the bronchiolar lumen (left) and expansion into the adjacent alveoli (right). Note stroma formation and newly formed capillaries. H&E, bar 50 μm

Fig. 22.5
Preneoplastic papillary proliferation at the bronchioloalveolar junction zone (BAJ) shows still a differentiation toward bronchiolar epithelium demonstrated by positivity for Clara cell protein 10. Bar 20 μm

Fig. 22.6
Atypia early on in this preneoplastic proliferation at the bronchioloalveolar junction. H&E, bar 20 μm

Fig. 22.7
Extension of the papillary proliferation into the alveolar tissue, completely filling the lumina. H&E, bar 20 μm

Fig. 22.8
Adenocarcinoma in situ with central necrosis, in the case to the right additionally bleeding into the necrosis. H&E, ×200
Within these larger nodules of in situ adenocarcinomas, necrosis did occur. Almost concomitant with necrosis, neoangiogenesis started (Fig. 22.9). Primitive stroma cells were seen expressing CD31 and CD34, deposition of newly synthesized matrix proteins occurred, primitive capillaries were formed, and the stroma developed features of a desmoplastic stroma reaction comparable to that seen in human carcinomas (Fig. 22.9). Once desmoplastic stroma has developed, the tumor cells started to invade this stroma, developing into invasive adenocarcinomas (Fig. 22.9). Angioinvasion was found in some cases (Fig. 22.10), but it seems that an interaction of different genes is necessary: in the HRAS Multi-Hit model, angioinvasion was only seen in those adenocarcinomas, which harbored activation of all three RAS effectors (MAPK, RAL, and PI3K) or additional TP53 or PTEN inactivation [48].



Fig. 22.9
(a) Invasive adenocarcinoma, note the desmoplastic stroma in the center, which had replaced the necrosis. (b, c) Details of areas of invasion. There are single cells (b) as well as small complexes of adenocarcinoma invading the stroma. (d) Neoangiogenesis is seen in the necrotic center of an adenocarcinoma and specifically at the border of invasion (e). H&E, immunohistochemistry with CD31 antibodies, bars 200, 50, and 20 μm

Fig. 22.10
Invasive adenocarcinoma with angioinvasion, two different examples are shown. H&E, bars 50 and 20 μm
In some models, peripheral adenomas developed in addition to nodular hyperplasia. The difference between nodular hyperplasia and adenoma is preservation of the alveolar structure in nodular hyperplasia and the original capillary network confined to the alveolar septa, whereas in adenomas the alveolar architecture is lost, and new capillaries are formed. In all models studied, these adenomas never progressed into a malignant lesion.
22.7.3 Immunohistochemistry as an Aid to Identify the Precursor Cell Population
Clara cells expressed Clara cell protein 10 (CC10) in normal bronchi and bronchioles. During the papillary proliferations starting from the bronchioloalveolar junction, the expression of CC10 was still retained within the cuboidal cells but, however, was lost in most cells, when adenocarcinomas in situ were formed (Fig. 22.11). Only single cells still expressed this protein. A similar reaction was seen for surfactant apoproteins (SApo): type II pneumocytes expressed SApoA and SApoB, and this expression was retained in pneumocyte hyperplasia, diffuse as well as nodular. In peripheral adenomas, this expression was lost in almost all cells. Few proliferating cells in the papillary bronchioloalveolar junction expressed SApoB. In adenocarcinomas in situ, this expression was lost; however, in invasive adenocarcinomas, SApoB could be demonstrated in the more differentiated cells in the center of the adenocarcinoma, especially in the papillary component. SApoC was expressed in pneumocytes type II in hyperplasia, but single cells also expressed SApoC in the bronchioloalveolar junction papillary proliferations. Also in adenocarcinomas in situ and in invasive adenocarcinomas, single cells and small-cell clusters expressed SApoC.


Fig. 22.11
Loss of Clara cell protein 10 expression during the development of an in situ adenocarcinoma. Some cells still express CC10; others are negative. Immunohistochemistry for CC10 protein
Using double staining for CC10 and SApoC in selected cases, double staining for these markers occurred in very few or only single cells (Fig. 22.12). Cells coexpressing CC10 and SApoC are regarded as peripheral stem cells residing in the niches of bronchioloalveolar junctions [49]. There was no expansion of these cells indicating that the tumor proliferation most probably started from more differentiated cells and not peripheral stem cells. The proliferation starting from the bronchioloalveolar junction was constantly positive for pan-cytokeratin, ruling out the presence of newly invading hematopoietic stem cells as the source of tumor cells [48].


Fig. 22.12
Coexpression of CC10 and SApoC in cells of an in situ adenocarcinoma. Only a few cells coexpressed both markers (arrows), which characterize them as peripheral lung stem cells. Immunohistochemistry with antibodies for CC10 (brown) and SApoC (red), ×400
Within the necrotic centers, newly formed blood vessels could be seen using immunohistochemistry for CD31 and CD 34. When immunohistochemistry was applied for podoplanin, which is a marker of endothelia of small blood vessels and lymphatics, dilated lymphatics were seen at the periphery of the in situ adenocarcinomas, but not in the necrotic centers (Fig. 22.9d, e). Neoangiogenesis of lymphatics was only seen after new blood vessels already appeared in the hemorrhagic or necrotic centers of the carcinomas. Lymphangiogenesis accompanied the invasion of the carcinomas, but did not precede it.
22.7.4 Progression of Adenocarcinomas
Stromal invasion in all mouse models depended on tumor size, the occurrence of hypoxia in the center, necrosis, desmoplastic stroma reaction, and angiogenesis. Invasion occurred only in large nodules within their center. The morphologic changes preceding invasion were hemorrhage and infarct-like necrosis suggesting a prominent role of hypoxia. Necrosis and hemorrhage were followed by formation of desmoplastic stroma and primitive blood vessels. Carcinoma cells invaded predominantly into the newly formed modified stroma. Importantly, hypoxia seems to promote invasiveness of human carcinomas [50–53] as well indicating that the mouse models could be an ideal tool to study the association of hypoxia, invasion, and metastasis.
Epithelial to mesenchymal transition (EMT) is a mechanism, which facilitates invasion and oriented tumor cell movement within the stroma. It can be easily diagnosed by the spindle cell morphology of the tumor cells, which express cytokeratin, vimentin, and smooth muscle actin (cytokeratin expression can even be lost). In humans, the most instructive lung cancer type displaying features for EMT is sarcomatoid carcinoma (spindle cell and pleomorphic carcinoma). The molecular basis for EMT in lung carcinomas might be pleiotropic and cannot be attributed to single genes [20, 54–66]. In the investigated mouse models, EMT seemed to play a minor role and was only represented in a single tumor by a small focus of cells with less than 1 mm in diameter. Here tumor cells acquired typical spindle cell morphology, but still retained expression of cytokeratin (Fig. 22.13) [48].


Fig. 22.13
Epithelial to mesenchymal transition (EMT) in a small invasive adenocarcinoma. The spindle cells in the center showed longitudinal microfilaments illustrated by immunohistochemistry for vimentin (not shown). There are a few lymphocytes within the central stroma where tumor cells already have invaded (upper panel). In the lower panel, EMT is associated with invasion in this adenocarcinoma. It is difficult to sort desmoplastic stroma cells versus adenocarcinoma cells with spindle cell morphology. However, the large atypical nuclei in the latter help for the separation. H&E, Movat, bars 50 and 20 μm
Invasion into blood vessel required additional genetic aberrations such as TP53 or PTEN deletion in HRAS Multi-Hit mice. Interestingly none of the adenocarcinomas in various mice displayed metastasis, which might be due to tumor overload. Up to 70 foci in lungs and additional pneumocyte hyperplasia might have reduced the ability of oxygenation, because type II pneumocytes are large and increased the distance between alveolar and capillary lumina. Therefore, even in cases with angioinvasion, the animals might have died due to respiratory insufficiency before metastasis could occur. Moreover, as pointed out above, induction of metastasis might require additional genetic modifications [67, 68].
22.7.5 Specific Changes Induced by Genetic Modifications
22.7.5.1 Signet Ring Cell Formation
Signet ring cell formation was not observed in the bronchioloalveolar junction papillary hyperplasia. It should be noted that signet ring cell morphology in the mouse models did not exactly reflect signet ring cell carcinomas in humans: most human signet ring cell carcinomas show accumulation of mucins within vacuoles formed within the cell cytoplasm. In the mouse models, no mucinous material is present, although the morphologic features are almost identical to human tumors. In mice the content in the cytoplasmic vacuole is more likely composed of lipids, which are usually dissolved during tissue processing. However, there was a wide variation in numbers of signet ring cells in mouse models, which correlated with genetic manipulations. Only a few signet ring cells could be encountered in HRAS Multi-Hit mice and in KRAS models with deletion of RANK or ATG5, whereas substantial and sometimes even dominant signet ring cell differentiation was found in KRAS-induced adenocarcinomas with deletion of apelin (Fig. 22.14).


Fig. 22.14
Pseudo-signet ring cell formation in the KRAS-induced mouse model with knockout of apelin. H&E, ×200
22.7.5.2 Oxyphilic/Oncocytic Changes
In the KRAS model with deletion of ATG5, an oncocytic transformation was seen across the whole sequence of tumor progression, starting with pneumocyte hyperplasia, peripheral adenomas, in situ adenocarcinomas, and invasive adenocarcinomas. Oxyphilic/oncocytic transformation was absent in all other mouse models.
22.7.6 Do Mouse Adenocarcinomas Resemble Human Adenocarcinomas?
The morphology of mouse lung carcinomas, induced by exposure to carcinogens, has been described in previous reports [8, 69, 70]. These chemical models never simulated adenocarcinomas of humans although rare cases of mucinous tumors were reported. Even squamous cell carcinomas were predominantly characterized as cystic tumors surrounded by an atypical squamous epithelium without signs of invasiveness. Genetically induced mouse lung tumors [5, 71] resembled human adenocarcinomas more precisely, but the sequence of events in mouse models was most often described by basic researchers not familiar with human lung carcinoma morphology. Recently, a consensus conference established a nomenclature for proliferative lesions evolving in mouse models [72]. This classification is a first step to precisely define the different sequences of proliferation in mouse models, but some aspects are still missing:
- 1.
Human lung adenocarcinomas arise after decades of carcinogen exposure, whereas tumors in mouse models develop within weeks.
- 2.
Only a few genetic changes give rise to mouse adenocarcinomas, which is in contrast to human tumors.
- 3.
Differences in the anatomy and histology of mouse and human lungs have not been taken into account.
Human adenocarcinomas can be separated into the common peripheral types, presenting with a lepidic, acinar, micropapillary, cribriform, and/or solid pattern. Usually one pattern is predominant but rarely only one pattern is present. In addition, peripheral adenocarcinomas can be further separated into mucinous and non-mucinous types. Rarely, a mixed mucinous and non-mucinous morphology can be observed. In addition, some rare variants exist such as fetal-, intestinal-, or colloid-type adenocarcinomas [73, 74]. Moreover, rare central adenocarcinomas with a morphologic pattern resembling bronchial glands but also solid and acinar adenocarcinomas can occur.
Adenocarcinomas in different mouse models morphologically present as papillary (Fig. 22.15) and solid types, where solid forms (Fig. 22.16) have to be carefully evaluated, because they are often pseudo-solid due to the filling of the alveoli with tumor cells. This is a common morphology of in situ adenocarcinomas. Lepidic, micropapillary, cribriform non-mucinous, and various forms of mucinous adenocarcinomas are never observed. Lepidic adenocarcinomas probably could not develop in a mouse lung because of the small diameter of alveoli and the relatively large size of the tumor cells. Therefore, in situ adenocarcinomas often showed a pseudo-solid morphology, but slit-like spaces of alveolar remnants are identified on higher magnification. Other types of adenocarcinomas are not formed because additional stimuli (e.g., for goblet cell formation) are missing during early steps of carcinogenesis [75–79]. Thus, only a small percentage of human pulmonary adenocarcinomas are morphologically represented in the mouse models, and for the vast majority, no suitable model exists.



Fig. 22.15
Invasive adenocarcinoma with differentiation into papillary subtype. H&E, ×200

Fig. 22.16
Invasive adenocarcinoma with a solid highly atypical component in the right lower area and a better differentiated one on top. H&E, ×200
The histopathology of HRAS- and KRAS-induced tumors suggests that these mice represent a model for non-mucinous peripheral thyroid transcription factor-1 (TTF1)-positive human adenocarcinomas. Papillary and solid differentiation was most commonly found in KRAS models, whereas solid and acinar adenocarcinomas were predominantly seen in HRAS models. Additional genetic modifications might be needed for development of micropapillary or cribriform non-mucinous adenocarcinomas as observed in human patients.
22.7.7 Differences in Mouse and Human Lung Morphology as Explanation for Different Adenocarcinoma Appearance
The fate of inhaled material is quite different in humans and mice: particulate materials are predominantly cleared in the upper respiratory tract of mice. The inhaled air circulates within the large sinusoidal areas where particulates are deposited. Therefore, inhaled particulates being either toxic and/or carcinogenic will act primarily in the upper respiratory tract [80, 81]. In addition, the airways of the mouse lung branch in a dichotomous manner, i.e., each bronchus divides into two equally sized bronchial branches. This results in an almost laminar airflow, and particles with an aerodynamic diameter of <2 μm will be deposited in the peripheral lung. In contrast, there is not much clearance of particulates in the nose and nasal sinuses of humans, but due to an asymmetric bronchial branching (a bronchus divides into a main branch with two thirds of the diameter and a minor bronchus with one-third diameter), particulates are deposited in the larger bronchi due to turbulences at the bifurcations. Therefore, even particulates with a small aerodynamic diameter might already be filtered out at central bronchial bifurcations. In human bronchi, the number of branches outnumbers those of mice more than three times, and the cell composition and distribution are different: in human bronchi, the ratio of ciliated to goblet cells is 6–8:1, whereas the number of goblet cells in the mouse is significantly lower. Ciliated cells occur in human lungs down to the larger bronchioles, whereas in mouse lungs, bronchioles are almost devoid of ciliated cells. In contrast, Clara cells in mouse lungs do not only occur in a substantial number in bronchioles, but also larger bronchi, whereas in human lungs, these cells are mainly confined to the terminal bronchioles and alveolar ducts. Secretory cells are forming one compartment in human and mouse bronchioles, but have been renamed as tuft cells or pneumocytes type III in mice. Short microvilli and secretory granules in the cytoplasm characterize these cells [82, 83]. Their function is unknown, but they can be found in well-differentiated peripheral human lung adenocarcinomas. These morphologic differences might partially explain some of the differences between human and mouse adenocarcinomas.
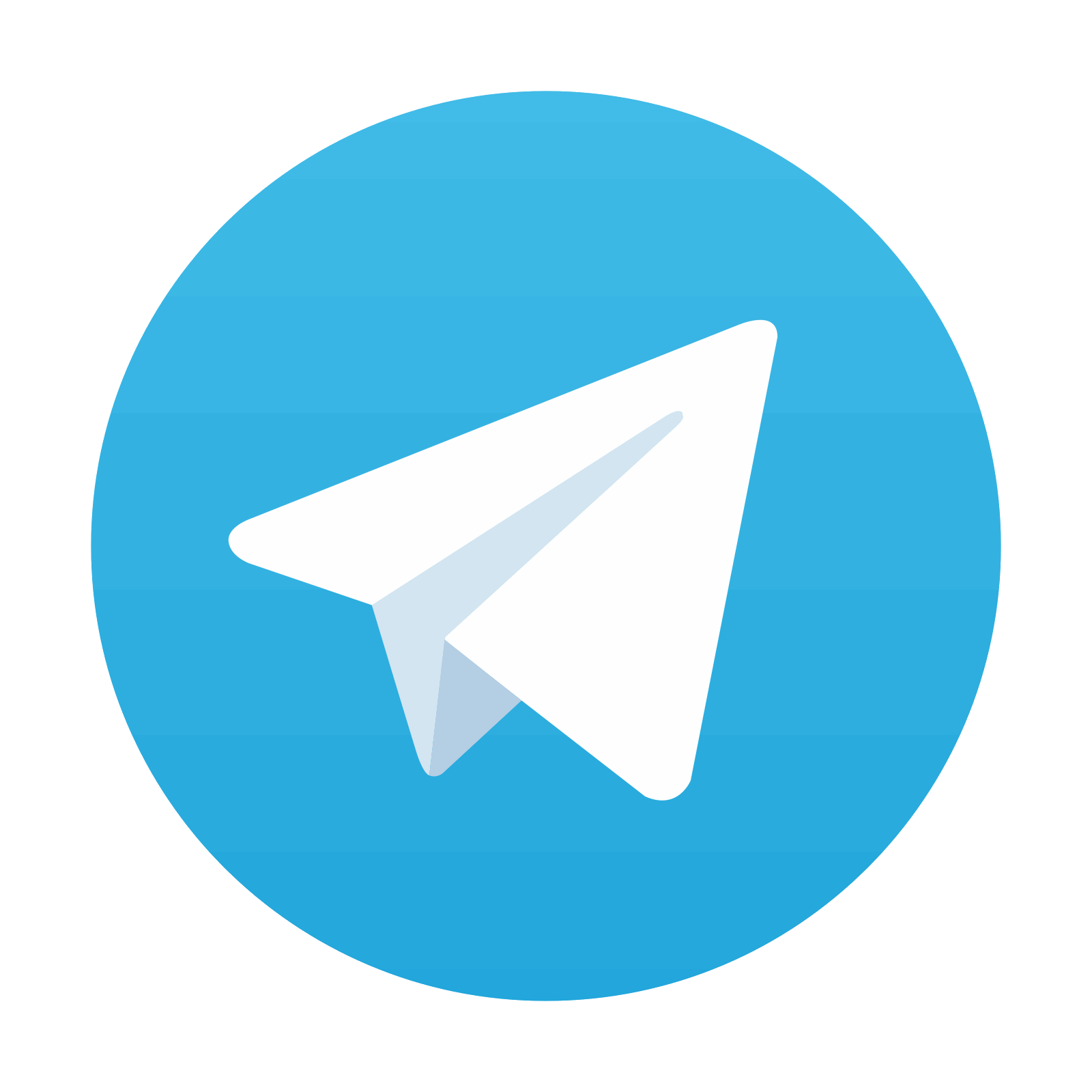
Stay updated, free articles. Join our Telegram channel

Full access? Get Clinical Tree
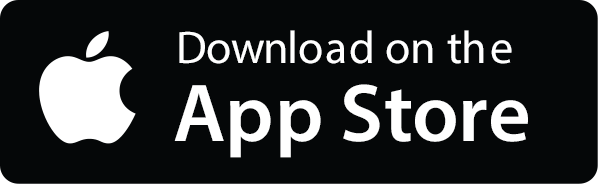
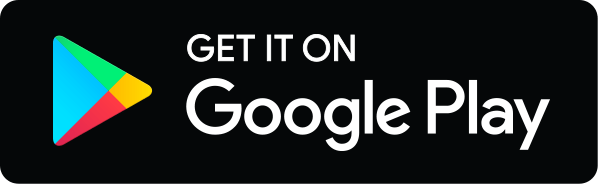