Background
The contribution of left ventricular (LV) twist and recoil in augmenting stroke volume during exercise is poorly understood, and few data are available describing the impact of endurance exercise on LV twist and recoil in middle-aged individuals. Therefore, the effects of chronic endurance training on these LV indices at rest and during submaximal exercise were examined in healthy, middle-aged untrained (MU) men and age-matched healthy, middle-aged endurance-trained (MT) men and in healthy, young untrained (YU) men as a healthy model.
Methods
Two-dimensional echocardiography with speckle-tracking imaging was used to quantify LV twist and recoil in 11 YU (mean age, 24.0 ± 4.1 years), 9 MU (mean age, 54.8 ± 4.3 years), and 12 MT (mean age, 53.8 ± 4.1 years) subjects at supine rest and during submaximal supine cycle ergometry.
Results
LV twist increased from rest to exercise in YU subjects (8.8 ± 3.8° to 12.8 ± 6.6°, P = .026) but not in MU subjects. LV twist also increased from supine rest to exercise in MT subjects (10.0 ± 3.1° to 13.1 ± 4.1°, P = .046). Time to peak untwisting velocity was significantly delayed in MU subjects during rest and exercise compared with both YU ( P = .005 and P = .02, respectively) and MT ( P = .018 and P = .045, respectively) subjects. No differences were detected in any of the timing indices of LV twist between YU and MT subjects.
Conclusions
This response pattern may help explain the mechanism underlying the training-induced augmentation in stroke volume during exercise, particularly the importance of the timing of key LV events that contribute to enhanced diastolic performance seen after endurance training.
The heart’s ability to increase stroke volume (SV) during exercise has been attributed to the synergistic effects of several mechanisms, which include enhancing myocardial contractility, increasing SV secondary to increasing preload (the Frank-Starling mechanism), reducing afterload, and increasing the rate of both ventricular emptying and ventricular filling. Although these mechanisms are related to left ventricular (LV) longitudinal and radial compression and expansion, other mechanisms attributed to motion in the circumferential plane have been identified as contributors to augmented SV. In particular, LV twist has emerged as a quantifiable measure to evaluate cardiac function in the circumferential plane, and further study is warranted to demonstrate the importance of LV twist as a contributing mechanism to increased SV during exercise.
The net difference between apical and basal LV rotation (measured in degrees) and rotation rate (measured in radians per second) during systole and diastole gives rise to LV twist and recoil, respectively, and LV twist and untwisting velocity, respectively. There appears to be a distinct sequence of events that dictates LV relaxation and filling, which begins with achieving peak untwisting velocity, followed by generation of the peak intraventricular pressure gradient, which is thought to create the diastolic suction that facilitates LV filling, and finally ends with establishing the peak early diastolic blood flow velocity across the mitral valve (peak E).
Although resting twist appears to increase with advancing age, peak untwisting velocity is both reduced and delayed, which may limit LV filling during exercise. However, there are few studies describing the effects of aging on twist and recoil during exercise in healthy individuals, and therefore further investigation is necessary to consolidate our current understanding of cardiovascular aging.
Although there are studies that have examined resting twist in young, highly trained endurance athletes, to the best of our knowledge, no studies to date have investigated LV twist at rest or during exercise in middle-aged, highly trained endurance athletes. Interestingly, those who have studied LV twist at rest in young, highly trained athletes failed to detect any significant differences in resting twist between the athletes and their sedentary control groups. Therefore, we sought to investigate the effects of endurance training on LV twist and recoil at rest and during submaximal exercise in middle-aged individuals, using healthy, young untrained (YU) individuals as our control group. We hypothesized that a history of chronic endurance training would result in preservation of LV twist and recoil both at rest and during exercise in middle-aged men, similar to LV twist and recoil demonstrated in the controls.
Methods
General Experimental Design
Participants initially underwent graded exercise testing to determine maximal aerobic power (maximal oxygen consumption [V o 2 max]). On a separate visit, two-dimensional (2D) echocardiography was used to assess LV function, with a primary emphasis on LV rotation indices in subjects lying supine at rest in the left lateral decubitus position and while cycling on a supine bicycle ergometer at a steady-state heart rate of 105 to 110 beats/min. Because the echocardiographic images were obtained during exercise, every precaution was taken to minimize artifacts associated with movement and increased through-plane motion that deteriorates the quality of the echocardiographic images. Consequently, a lower exercise intensity was used to maximize image quality.
Study Population
Thirty-two male volunteers were recruited from the general community, representing three groups: YU men (aged 18–35 years) who reported <2 hours of vigorous aerobic exercise per week for the past 3 years ( n = 11); middle-aged untrained (MU) men (aged 50–65 years) who reported the same aerobic exercise as above ( n = 9), and middle-aged endurance-trained (MT) men (aged 50–65 years) with histories of ≥6 hours of vigorous aerobic exercise per week for the past 3 years and competitive participation in endurance events within the past 3 years ( n = 12). The MT group was composed of seven cyclists, three triathletes, and two long-distance speed skaters. Vigorous aerobic exercise was defined as a planned session of aerobic activity that was performed for the purpose of training, in which ≥50% of the exercise session was carried out at ≥6.0 metabolic equivalents or at a V o 2 max ≥ 60%. This definition incorporates the American Heart Association’s 2003 physical activity and health guidelines definition of vigorous aerobic exercise. In addition to the classification requirements for each group stated above, objective criteria based on V o 2 max results as stipulated by the American College of Sports Medicine were used to classify endurance-trained versus untrained subjects. Endurance-trained and untrained subjects fell above the 85th percentile and below the 50th percentile of their age-predicted V o 2 max, respectively. On the basis of history and resting electrocardiographic findings, all subjects were considered to be healthy, with a low likelihood of having coronary heart disease, and were free of any medications. All subjects were normotensive, were nonsmokers, and did not have diabetes. The Physical Activity Readiness Questionnaire was successfully completed by all subjects. The study was approved by the hospital and university research ethics boards, and informed consent was obtained from all participants before commencing the study.
Maximal Exercise Testing
Graded exercise testing for determination of V o 2 max was performed by all subjects using an upright cycle ergometer (Ergoline 850; Ergoline GmbH, Bitz, Germany). After an initial workload of 25 W, the work rate was increased by 25 W/min until exhaustion. Breath-by-breath expired gases were collected using a metabolic cart (Moxus Modular System; Applied Electrochemistry Inc., Pittsburgh, PA) and recorded as 20-sec averages, with heart rate monitored throughout the exercise test (Polar810i) and recorded electronically (HRTrak II Heart Rate Tracker; Equilibrated Bio Systems Inc., New York, NY). V o 2 max was confirmed by the following criteria: (1) a plateau in oxygen consumption despite an increase in exercise intensity, (2) achievement of age-predicted maximal heart rate values, and (3) attainment of a respiratory exchange ratio ≥ 1.15.
Echocardiography
Resting and exercise 2D echocardiography was performed without caffeine consumption during the previous 12 hours and upon consumption of 500 mL of water within 1 hour of testing. All images were obtained using an M4S probe on a commercial system (GE Vivid 7 & Imaging System version BT03-5; GE Healthcare, Milwaukee, WI) in accordance with the American Society of Echocardiography. Short-axis views of the left ventricle were taken at the basal and apical levels to evaluate twist indices. Landmarking of the left ventricle in the short-axis view was performed as follows: the base of the left ventricle was defined as the level of the mitral valve with mitral leaflets on view, and the apex of the left ventricle was defined as a circular cavity with no papillary muscle visible and recorded as distally as possible. Short-axis views were taken at a frame rate of 60 to 90 Hz. Parasternal long-axis views were obtained at rest in the left lateral decubitus position to determine LV wall mass index, wall thicknesses, and internal dimensions using M-mode echocardiography. Pulsed-wave Doppler recordings of the LV inflow and outflow tracts were acquired for the assessment of diastolic function and timing of systole, respectively.
LV Twist Analysis
All ultrasound data were analyzed offline by a single trained observer using commercial software on a proprietary workstation (EchoPAC version 7; GE Healthcare). At least three consecutive cardiac cycles were analyzed and averaged whenever possible. Using both apical four-chamber and two-chamber views, LV volumes and LV ejection fraction were measured using the modified biplane Simpson’s method. Twist indices were determined by assigning counterclockwise rotation at the apex of the left ventricle to be positive, in conformity with convention. LV twist was defined as the net difference between apical and basal rotation angles, measured in degrees, while twist and untwisting velocities were calculated as the net difference between apical and basal rotation rates in radians per second. For each rest and submaximal exercise stage, the optimal images obtained from basal and apical short-axis slices that were within ±2 beats/min were selected and analyzed using speckle-tracking imaging (STI). Upon tracing the endocardial border from a frame at end-systole and specifying an appropriate region of interest, STI was applied on a frame-by-frame basis. The sum of absolute differences algorithm using the EchoPAC software generated the time domain profiles for LV rotation and LV rotation rate for each of the six equidistant segments defined in both basal and apical short-axis planes. The LV rotation and rotation rate profiles for each of the six equidistant segments were averaged to obtain final values for LV twist, twist velocity, and untwisting velocity. All twist indices were calculated from basal and apical data exported to spreadsheet software (Excel; Microsoft Corporation, Redmond, WA).
Interobserver Reliability
To ensure that measuring LV twist during submaximal exercise was feasible, the intraclass correlation coefficient was calculated for 10 randomly selected participants to determine interobserver differences. The intraclass correlation coefficient was determined to be 0.84, which indicates nearly perfect agreement between the observers (values between 0.8 and 1.0 indicate nearly perfect agreement).
Statistical Analysis
A repeated-measures analysis of variance with interaction was used to determine significance of main effect. The one within-group factor was for condition (rest vs exercise). Independent t tests using Bonferroni’s post hoc analysis were used to assess differences in main effect between young and old groups. For all statistics, P values < .05 were considered statistically significant. All values in tables, figures, and the text are reported as mean ± SD.
Results
Subjects
Subject characteristics are presented in Table 1 . Both the MU and MT subjects were matched for age ( P > .05) and were significantly older than YU subjects by design ( P < .001). The MU subjects were significantly heavier than YU ( P < .001) and MT ( P = .04) subjects and had greater body mass indexes compared with YU subjects ( P < .05). All subjects achieved at least two of the three requirements stipulated above in the determination of V o 2 max. The YU subjects achieved greater V o 2 max values than MU subjects ( P = .02), but both groups fell between the 40th and 50th percentiles for their age-predicted V o 2 max values as stipulated by the American College of Sports Medicine sixth edition guidelines. MT subjects achieved greater V o 2 max values than both YU ( P = .026) and MU ( P < .001) subjects and fell above the 90th percentile for their age-predicted V o 2 max values. Although the majority of LV internal dimensions and wall thicknesses appeared to be greater in MU and MT subjects compared with YU subjects, none of those measures was significantly different among the three groups. LV mass index and relative wall thickness, however, were significantly greater in MT subjects compared with both MU and YU subjects ( P ≤ .005 for all comparisons).
Variable | YU subjects ( n = 11) | MU subjects ( n = 9) | MT subjects ( n = 12) |
---|---|---|---|
Age (y) | 24.0 ± 4.1 | 54.8 ± 4.3 ∗ | 53.8 ± 4.1 ∗ |
Height (m) | 1.76 ± 0.06 | 1.82 ± 0.03 | 1.77 ± 0.07 |
Weight (kg) | 69.6 ± 5.9 | 81.7 ± 5.0 ∗ | 75.1 ± 4.8 † |
Body mass index (kg/m 2 ) | 22.5 ± 1.4 | 24.8 ± 2.1 ∗ | 23.9 ± 2.1 |
V o 2 max (mL/min/kg) | 41.4 ± 6.5 | 33.8 ± 5.0 ∗ | 47.9 ± 4.3 ∗ † |
IVSd (cm) | 1.0 ± 0.2 | 0.9 ± 0.2 | 0.9 ± 0.3 |
LVIDd (cm) | 4.2 ± 0.5 | 4.5 ± 1.0 | 4.5 ± 0.8 |
LVPWd (cm) | 0.9 ± 0.4 | 1.0 ± 0.6 | 1.0 ± 0.4 |
LVIDs (cm) | 2.8 ± 0.3 | 3.1 ± 0.7 | 3.0 ± 0.7 |
LVMI (g/m 2 ) | 70.2 ± 11 | 58.4 ± 8 ∗ | 90.3 ± 15 ∗ † |
RWT | 0.34 ± 0.02 | 0.36 ± 0.05 ∗ | 0.38 ± 0.01 ∗ † |
∗ Different from YU subjects ( P < .05).
Hemodynamic Response to Exercise
Hemodynamic responses to supine, submaximal leg exercise are shown in Table 2 . MT subjects had significantly lower resting heart rates compared with YU subjects and exhibited greater increases in heart rate with exercise compared with YU subjects. There were no significant differences in systolic and diastolic blood pressures, LV ejection fractions, end-diastolic volumes, or end-systolic volumes at rest or during submaximal exercise between the groups.
Variable | Condition | YU subjects | MU subjects | MT subjects |
---|---|---|---|---|
Heart rate (beats/min) | Rest | 66 ± 10.0 | 60 ± 3.8 | 57 ± 7.3 † |
Exercise | 104 ± 2.2 ∗ | 105 ± 1.9 ∗ | 104 ± 2.3 ∗ | |
Systolic blood pressure (mm Hg) | Rest | 107 ± 6 | 114 ± 10 | 112 ± 10 |
Exercise | 141 ± 5 ∗ | 144 ± 9 ∗ | 144 ± 3 ∗ | |
Diastolic blood pressure (mm Hg) | Rest | 65 ± 6 | 70 ± 10 | 69 ± 10 |
Exercise | 87 ± 5 ∗ | 88 ± 7 ∗ | 84 ± 8 ∗ | |
LV ejection fraction (%) | Rest | 61.7 ± 2.7 | 61.2 ± 2.4 | 61.6 ± 4.0 |
Exercise | 68.1 ± 1.8 ∗ | 67.4 ± 3.0 ∗ | 68.8 ± 4.8 ∗ | |
LV end-diastolic volume (mL) | Rest | 97 ± 20 | 108 ± 22 | 97 ± 29 |
Exercise | 99 ± 25 | 102 ± 30 | 101 ± 23 | |
LV end-systolic volume (mL) | Rest | 37 ± 9 | 42 ± 10 | 38 ± 14 |
Exercise | 32 ± 9 ∗ | 31 ± 9 ∗ | 31 ± 9 ∗ |
∗ Different from rest ( P < .05).
LV Diastolic Response to Exercise
Indices of diastolic function at rest and during submaximal exercise are presented in Table 3 . Peak E was significantly greater at rest in YU subjects compared with both MU and MT subjects ( P < .02 for both). However, there were no differences in peak E with exercise between the groups. Peak A was significantly higher in MU subjects at rest and during exercise compared with not only YU but also MT subjects ( P < .01 for all conditions). Transmitral valve E/A ratios in YU subjects were significantly increased compared with MU and MT subjects at rest ( P ≤ .003 for both). Under exercise conditions, both YU and MT subjects had significantly higher E/A ratios than MU subjects ( P < .001 and P = .02, respectively). The time to peak E from the onset of the QRS signal on electrocardiography decreased with exercise for all groups ( P < .001 for all conditions). During exercise, MT subjects had significantly shorter times to peak E from the onset of QRS signal than YU subjects ( P = .002) but not compared with MU subjects. The time to peak E from mitral valve opening (MVO) also decreased with exercise across all groups ( P ≤ .001 for all conditions). During exercise, MU subjects had significantly delayed time to peak E from MVO compared with YU and MT subjects ( P = .015 for both). There were no significant differences in time to peak E from MVO between YU and MT subjects under resting or exercise conditions. Bivariate correlation analysis revealed a significant correlation between time to peak E from MVO during supine exercise and V o 2 max ( r = −0.50, P = .007).
Variable | Condition | YU subjects | MU subjects | MT subjects |
---|---|---|---|---|
Peak E-wave velocity (m/sec) | Rest | 0.91 ± 0.10 | 0.73 ± 0.22 † | 0.73 ± 0.16 † |
Exercise | 1.15 ± 0.27 ∗ | 1.14 ± 0.27 ∗ | 1.14 ± 0.18 ∗ | |
Peak A-wave velocity (m/sec) | Rest | 0.52 ± 0.11 | 0.81 ± 0.13 † | 0.62 ± 0.14 ‡ |
Exercise | 0.60 ± 0.21 | 1.08 ± 0.19 ∗ † | 0.75 ± 0.24 ‡ | |
Mitral valve E/A ratio | Rest | 1.8 ± 0.4 | 0.9 ± 0.2 † | 1.2 ± 0.5 † |
Exercise | 2.0 ± 0.4 | 1.1 ± 0.3 † | 1.7 ± 0.6 ‡ | |
Mitral valve deceleration time (msec) | Rest | 188 ± 41 | 164 ± 46 | 190 ± 43 |
Exercise | 146 ± 38 ∗ | 144 ± 28 ∗ | 127 ± 55 ∗ | |
Time to peak E from onset of QRS signal on electrocardiography (msec) | Rest | 514 ± 26 | 534 ± 11 | 527 ± 24 |
Exercise | 411 ± 11 ∗ | 402 ± 11 ∗ | 387 ± 21 ∗ † | |
Time to peak E from MVO (msec) | Rest | 93 ± 7 | 97 ± 14 | 92 ± 10 |
Exercise | 72 ± 6 ∗ | 82 ± 8 ∗ † | 72 ± 8 ∗ ‡ |
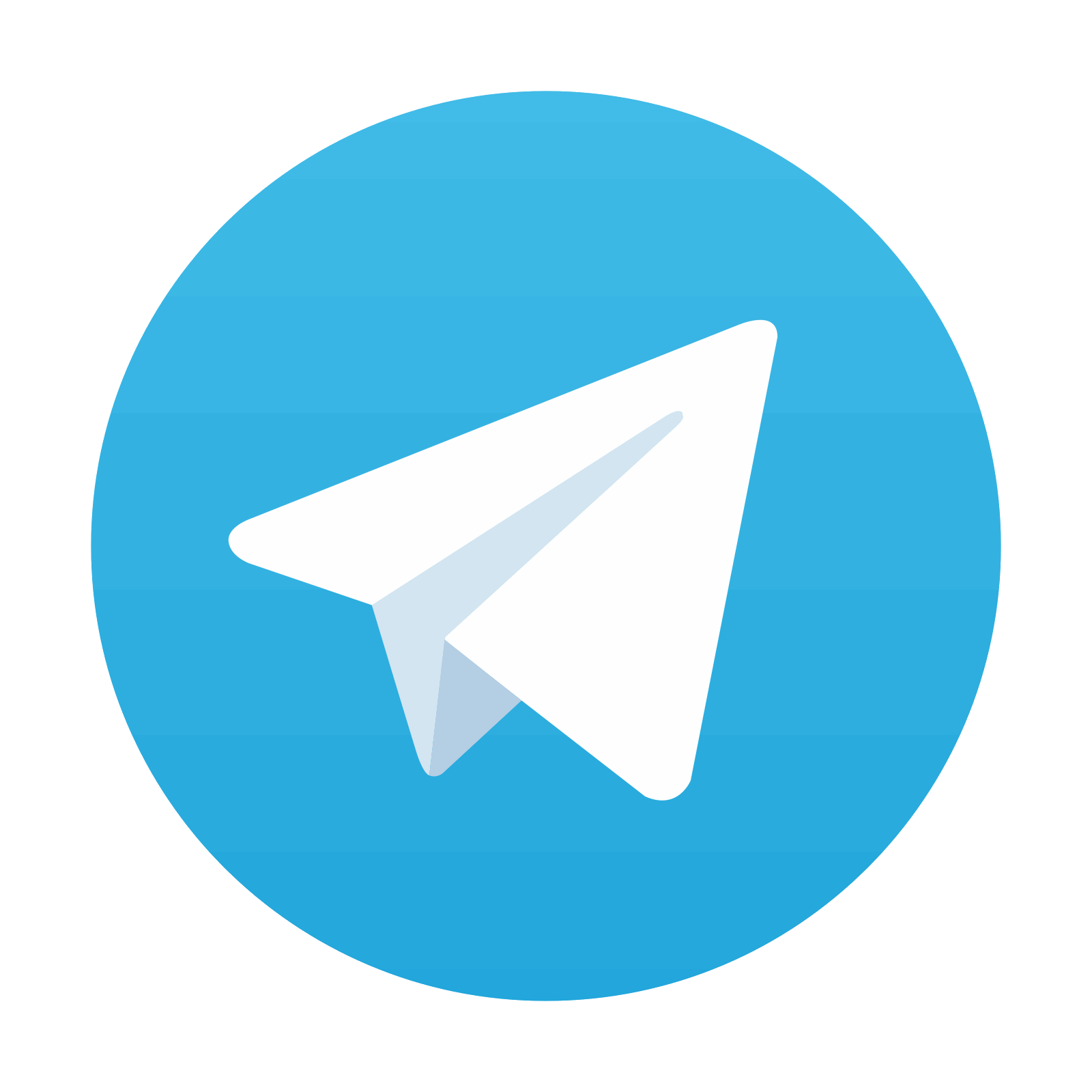
Stay updated, free articles. Join our Telegram channel

Full access? Get Clinical Tree
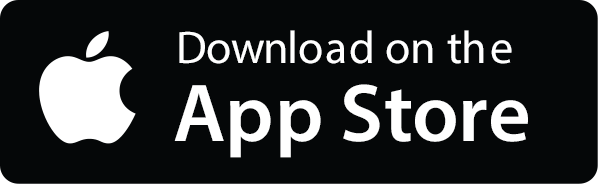
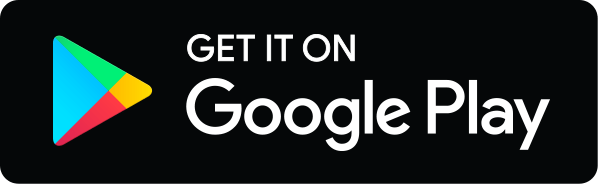
