Outcome
Strength of evidence
Improvement of exercise performance
A
Dyspnea relief
A
Improves health-related quality of life
A
Reduces number of hospitalizations and days in hospital
A
Reduces anxiety and depression
A
Upper limbs strength/endurance training improves arm function
B
Benefits persist beyond training period
B
Improves survival
B
Improves recovery after hospitalization for exacerbation
B
Enhance the effect of long-acting bronchodilators
B
Respiratory muscle training can be beneficial, especially when combined with general exercise training
C
9.1.3 Why Should the Treatment of the COPD Be more than Mono-pharmacotherapy and Include a Multidisciplinary Approach?
The basic pathogenesis of COPD is small airway disease and emphysema, which creates limited airflow. This limitation in airflow, in turn, causes ventilatory impairment by the dynamic hyperinflation on exertion and hypoxemia [5]. This results in the characteristic symptoms of COPD, such as shortness of breath and physical limitation on exertion. Due to these conditions, it becomes necessary to reduce dynamic hyperinflation to treatment and to increase inspiratory capacity (IC) via the use of a bronchodilator. The bronchodilator relieves shortness of breath by relaxing the bronchial smooth muscle which in turn expands the bronchial airways to eliminate the airflow restriction and hyperinflation. Therefore, since the mechanism of action of a bronchodilator directly acts to counter the pathophysiology of the COPD, it is an appropriate treatment regimen.
Since the limitation in bronchial airflow greatly contributes to shortness of breath, COPD patients are often considered unable to exercise. However, the symptoms of COPD patients are not simply limited to ventilatory impairment since many COPD patients also experience muscle fatigue [6]. Killian et al. examined the factors that limited exercise in 97 patients with COPD and 320 healthy subjects. They found that only 26 % of COPD patients and 22 % of healthy subjects reported dyspnea as a limiting factor, while 43 % and 36 %, respectively, reported lower limb fatigue as a limiting factor [7]. Hamilton et al. conducted incremental exercise testing in COPD, CHF, and healthy subjects and determined the reasons for discontinuation of exercise in each group. In all of the groups, the combination of dyspnea and lower limb fatigue was a more common reason than dyspnea alone as the patients’ reported reason for the discontinuation of exercise [8].
In exercise, the skeletal muscle contributes to acidosis via the production of lactic acid which leads to an increase in the respiratory center’s ventilation drive. This abnormal hyperventilation is regarded as an important factor in perpetuating pathologic dyspnea in COPD. COPD patients also attempt to increase their ventilatory drive during exertion; however, due to their dynamic hyperinflation, this results in shortness of breath secondary to inadequate ventilation. To exacerbate this situation, most patients with COPD naturally avoid moving and exertion due to their dyspnea; thus, this results in vicious circle that accelerates their deconditioning (Fig. 9.1) [9].
There are two methods to prevent the dynamic hyperinflation of patients with COPD. The first is a method that reduces airway resistance to quicken the expiratory phase. The second is a method that reduces the respiratory rate, thus increasing the total time for expiration. The current mainstream therapeutic drug is the bronchodilator that reduces hyperinflation primarily by the mechanism of action we previously described. In addition, the inhalation of oxygen also contributes to the reduction of dynamic hyperinflation through the oxygen-sensing chemoreceptors and signaling of the carotid body. In addition, the inhalation of oxygen is thought to reduce dynamic hyperinflation through a mechanism that reducing respiratory rate involves signaling from the carotid body. Furthermore, exercise training will acclimate the skeletal muscle to exercise, which will decrease its production of lactic acid. This decrease in lactic acid production improves hyperinflation because the ventilatory drive is now inhibited, which in turn reduces the respiratory rate [10]. In addition, the muscle itself displays changes induced by exercise, which we will discuss later.
Because dyspnea in COPD, as mentioned above, is a phenomenon that involves multiple factors, a multidisciplinary approach to treatment is beneficial because it targets the multiple mechanisms of COPD and not just any single mechanism. Therefore, most patients require both pharmacotherapy and non-pharmacologic therapies (e.g., exercise therapy, oxygen therapy, nutritional therapy, and patient education). Management of the patients with COPD according to the guidelines of Japan [11] is based on these concepts (Fig. 9.2).
9.1.4 When to Start Rehabilitation?
As for many COPD patients, initial treatment is usually pharmacotherapy, but the question remained as to when it should be initiated and at what point exercise rehabilitation should be added to the regimen. GOLD 2010 initially addressed this question by recommending that rehabilitation should target patients with stage II or more (% FEV1 < 80). However GOLD 2011 has outlined a new set of patient management recommendations which modifies the target of exercise rehabilitation to those who are equal to or greater than Group B. Group B encompasses patients with strong subjective symptoms (CAT > 10 or modified MRC scale ≥ 2) and patients with a risk of progression (exacerbation frequency is more than twice a year or % FEV1 < 50). GOLD 2011 maintains the enforcement of physical activity for patients in Group A, who have mild symptoms and a low risk of future exacerbation [5]. On the other hand, the Japanese COPD guidelines do not contain a similar outline of specific criteria [5]. Patients with symptoms and disabilities of all stages are targeted. However, the guideline that does exist involves insurance; insurance does not cover services for pulmonary rehabilitation unless patients have greater than COPD stage II or modified MRC scale ≥ 2.
Compared to severe COPD, there is little data available to determine the effect of exercise rehabilitation in mild-to-moderate COPD. In the INTERCOM study, which was a randomized controlled trial (RCT) conducted over a 2-year period in the Netherlands, rehabilitation made accessible by offering it within the patients’ local communities proved to be beneficial even for patients with mild-to-moderate COPD (mean % FEV1 ≥ 60 %). These patients displayed a significant improvement in quality of life, exercise tolerance, and shortness of breath when compared to the non-rehabilitation group [12].
In addition, the already decreased intramuscular oxidation capacity decreases further in mild COPD at a stage where muscle consumption of oxygen is not clear. In addition, it is also noted that exercise duration is shorter than that of a healthy subject [13]. The results of these studies also suggest that muscle training by pulmonary rehabilitation is not just necessary for the critically ill patients, but it is also beneficial when introduced at an early stage of COPD. At the very least, pulmonary rehabilitation is an intervention that is considered a standard of care for individuals who have COPD and remain symptomatic despite optimal bronchodilator therapy.
9.1.5 What Kind of Contribution Does Exercise Training Make?
Variations in exercise training can produce a difference in clinical effects. The different aspects of an exercise training regimen that can differ include the setting (inpatient vs. outpatient vs. home based), duration (short term vs. long term), and intensity (high vs. low intensity), which can all influence the observed clinical response. The high-intensity load refers to around 60–80 % of exercises that require maximum oxygen uptake, and the low-intensity load usually points to 40–60 % of exercises that require maximum oxygen uptake. The international guidance for pulmonary rehabilitation recommends a program that consists of 6–12 weeks, 2–3 sessions/week of 30–60 min duration at moderate-to-high exercise intensities [4].
Exercise of the high strength load and intensity has been said to be effective in the guidelines for a long time. However, for the elderly patients who have severe dyspnea, it is actually often difficult to perform exercise of the high-intensity training. Furthermore, there is the problem of the difficulty with the continuation of these high-intensity exercises. Also, the problem of costs and the access affects the ability for continuity in the exercise plan. It has recently been reported that even low-intensity training is beneficial. Baumann et al. conducted an RCT of a 26 week outpatient rehabilitation for 100 patients with moderate to severe cases of COPD. Associated with low-intensity exercise was a dyspnea severity score of 4–6 points on the Borg Scale. These results were compared to another exercise intervention group and a control group without a rehabilitation intervention. The intervention group had a significant improvement in 6MD (+59 m), HRQOL (SGRQ −5 points) [14]. Since it took a long time to see results, continuity of the program was ensured. Low-intensity load exercise is believed to be particularly suitable for the elderly and critically ill patients.
Endurance training has two modes: continuous exercise and interval exercise. High-intensity training has more effect on exercise capacity than low-intensity training, but in patients with severe COPD, it can be difficult to sustain high intensity by the continuous exercise. Although dynamic lung hyperinflation increases progressively during continuous exercise, interval exercise reported to induce less dynamic hyperinflation than continuous exercise in patients with COPD [15]. Beauchamp et al. reported that interval and continuous training modalities did not differ in their effect on measures of exercise capacity or HRQOL. They concluded that interval training may be considered as an alternative to continuous training in patients with varying degrees of COPD severity [16].
9.1.6 What Are the Problems Encountered While Undergoing Rehabilitation?
There are several problems associated with pulmonary rehabilitation. First, there is a low implementation rate of rehabilitation. According to the questionnaire survey for the certification facility of the Japanese Respiratory Society, only 57.3 % of facilities have implemented pulmonary rehabilitation. A number of hospitals that have implemented pulmonary rehabilitation differ regionally. When institutions that had not implemented rehabilitation were questioned as to why, 80 % reasoned lack of manpower, while 30 % reasoned lack of profit [17]. According to the questionnaire results of patients with the Japanese White Paper on Home Respiratory Care, 49 % were rehabilitated at the first investigation in 2005, but only 53 % of those maintained rehabilitation when reexamined in 2010.
In regional community facilities, there is also a new attempt to provide a maintenance program in cooperation with the exercise instructor; however, this is not done at the large medical institutions [18]. The teaching methods of COPD patients included an exercise instructor and exercise training of COPD patients in the community facilities of the region rather than a hospital (twice a week, 1 h or more once) for 6 months. After 6 months and 12 months, 6-min walk distance (6MWD) improved (pre-intervention, 385 m; after 6 months of intervention, 441 m; 12 months, 454 m; P < 0.001), and HRQOL was also significantly improved. The study attracted attention despite being carried out in a small regional program because there was a general exercise instructor, who was not a healthcare worker, working with them. Another problem with the rehabilitation is maintenance of the program over a long period of time has not been established. According to the results of the meta-analysis of maintenance of rehabilitation, the intervention group with 9–15 months of maintenance was significantly better than the usual care group after 6 months of building exercise tolerance. However, there was no significant difference when the program continued for 12 months. In addition, between the usual care groups, there was no significant difference in HRQOL after 6 or 12 months. Furthermore continuation rate of these maintenance programs was only 60 % [19]. Determining how to provide programs that enhance continuity has become the problem.
9.2 Does Exercise Affect the Muscle of COPD Patients?
9.2.1 Muscle Structure in the COPD and Metabolic Change
The disordered muscle function in patients with COPD affects muscular strength and decreases the endurance of the muscle. The muscular strength is decided by size and the thickness of the muscle, but the muscular endurance is decided by the amount of glycogen stored. Factors that influence the function of the muscle include intramuscular capillary density, oxygen delivery, and oxidative capacity of the myofibers. Table 9.2 presents the structural and functional abnormalities of the muscle found in COPD. According to a European study, in about 1/3 of patients only possess about 25 % of the average strength of healthy adults [20]. In addition, recent meta-analysis concludes that there is also a decrease of the quadriceps femoris muscle when compared to a healthy control [21].
Table 9.2
Structural and functional abnormalities of muscles in COPD
(1) Muscle fiber distribution change |
↓ Type I fibers (slow twitch, oxidative) |
↑ Type IIx fibers (fast twitch, glycolytic) |
(2) Reduced muscle fiber size |
(3) Reduction in the capillary numbers and contacts |
(4) Decreased oxidative capacity (reduced mitochondrial content) |
(5) Early lactic acidosis increases ventilator demands at lower exercise intensity |
Biopsies of a COPD patient’s femoral muscle (vastus lateralis) show that when compared to healthy controls, there is a decrease in type I muscle fibers (slow-twitch fibers) and an increase in type IIx fibers (fast-twitch fibers). Because oxidative metabolism is dominant in the type I fiber, these are characteristics of fibers for endurance and are thus the less-fatigable muscle fibers. On the other hand, type IIx muscle fibers predominantly utilize glycolytic metabolism, which contributes to agility, but are easily fatigued muscle fibers. These fibers are often mixed, and the proportion at which they are mixed varies according to the site of the muscle. The muscle fibers of patients with severe COPD are thin, thus contributing to a decrease in muscle mass [22].
Jobin et al. compared muscle biopsies from COPD and healthy subjects and found that capillary number per unit area in COPD was significantly less than healthy subjects (92.6 ± 16.1 and 213.3 ± 33.5, P < 0.001), and the ratio of capillaries per muscle fiber was reduced (0.83 ± 0.05 in COPD and 1.56 ± 0.10 in healthy subjects, p < 0.001) [23]. Oxygenation thereby decreases because contact with the muscle fiber decreases when the size of an intramuscular capillary decreases [24].
The aerobic metabolism in the skeletal muscle increases on exercise, but this metabolism usually depends on enough oxygen being supplied and adequate ATP production by the intramuscular mitochondria. As for patients with COPD, they have a higher rate of anaerobic metabolism when compared to healthy controls, as noted by the hyperventilation at an early stage of exercise associated with the increase in blood lactic acid concentrations, thus contributing to a decrease in muscular endurance. This is associated with a decreased mitochondrial content in the skeletal muscle [25].
It is thought that the change of these muscles in a COPD patient is caused by disuse, systemic inflammation, oxidative stress, hypoxemia, undernutrition, and a side effect of steroid treatment. Recently Natanek et al. reported that a reduction of phospho-AMPK is involved in the reduction of oxidative capacity and endurance of COPD patients [26].
9.2.2 Change of the Muscle by the Exercise
Exercise training is found to not only improve muscle composition, but it also improves exercise capacity and the quality of life. Vogiatzis et al. studied patients with COPD (GOLD stages II, III, and IV) who underwent comprehensive pulmonary rehabilitation three times a week of high-intensity exercise training for 10 weeks and then examined the changes in muscle composition by vastus lateralis muscle percutaneous biopsy. As a result, type I fiber or type IIa increased after rehabilitation, and type IIb decreased (Fig. 9.3). Moreover, the capillary ratios for the muscular fiber increased. Furthermore, they concluded that because the changes of these muscles were found to be independent on the severity of COPD, then they could expect that severity of COPD would also have no effect on exercise training [27].


Fig. 9.3
Fiber-type distribution of the vastus lateralis muscle before (open bars) and after (solid bars) rehabilitation in patients with COPD in GOLD stages II, III, and IV. (a) Type I. (b) Type II. (c) Type IIa. (d) Type IIb. Data are represented as means ± SEM. Asterisks denote significant differences between before and after training (P < 0.01) (Reproduced by permission from Reference [27])
Puente-Maestu et al. reported that exercise training sessions in pulmonary rehabilitation programs produced a significant decrease in the number of mitochondrial DNA in skeletal muscle of patients with COPD, as well as overexpression of peroxisome proliferator-activated receptor-γ coactivator-1α (PGC-1α) mRNA, most likely associated with the enhanced oxidative stress [28].
9.3 Physical Activity and Exercise
9.3.1 The Importance of Physical Activity
Exercise capacity and physical activity are different concepts. Physical activity has been traditionally defined as “any bodily movement produced by skeletal muscles that results in energy expenditure,” and this definition has been adapted to physical activity in daily life as “the totality of voluntary movement produced by skeletal muscles during every day functioning” [29]. On the other hand, the exercise capacity is described by how well and how much. The exercise capacity is commonly evaluated by 6-min walk test (6MWT) or a shuttle walk test.
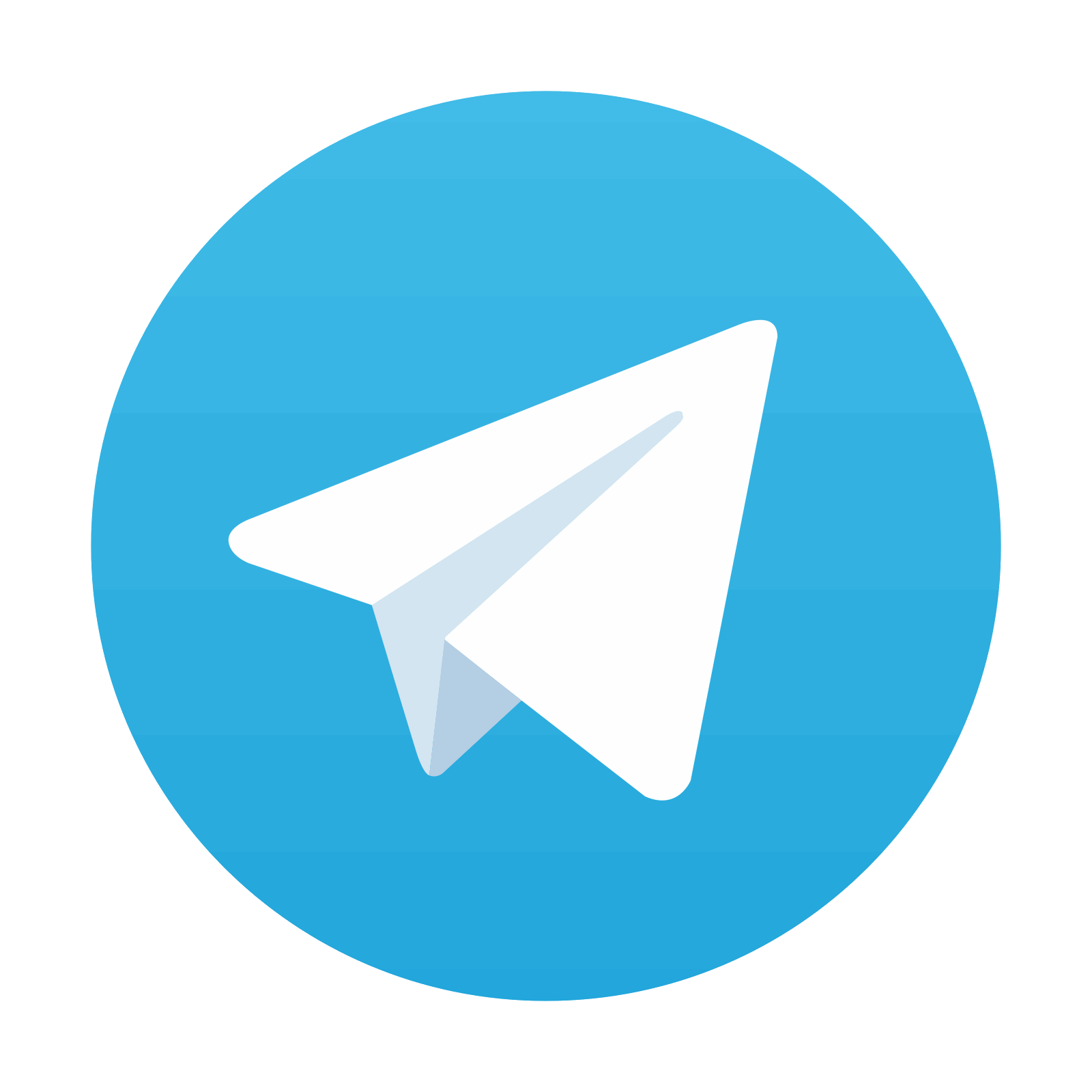
Stay updated, free articles. Join our Telegram channel

Full access? Get Clinical Tree
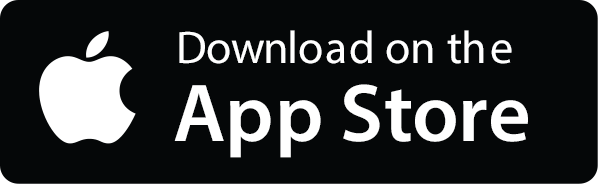
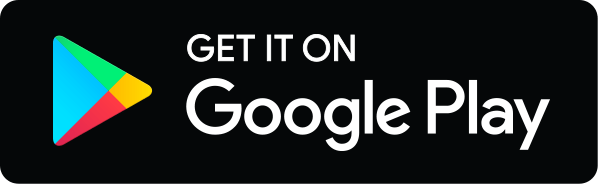