Exercise and Physical Activity
Barry A. Franklin
Exercise training appears to play an important role in the medical management and rehabilitation of patients with coronary heart disease (CHD). The salutary effects of chronic exercise training, increased lifestyle physical activity, or both are well documented. Numerous studies also suggest that cardiorespiratory fitness, expressed as metabolic equivalents (METs; 1 MET = 3.5 mL O2/kg/minute), is one of the strongest prognostic markers in persons with and without CHD.1 Accordingly, sedentary patients should be counseled to engage in regular walking at moderate exercise intensities so they can move out of the least fit, least active, “high-risk” cohort (bottom 20%).2
There are, however, limitations to the benefits that exercise training offers relative to the prevention of initial and recurrent cardiovascular events. Contrary to the speculation of a few overzealous enthusiasts, regular exercise training, regardless of the intensity, duration, or frequency, does not confer “immunity” to CHD.3 Furthermore, exertion-related cardiovascular events have been reported in the medical literature4 and the lay press,5 suggesting that strenuous physical activity may actually trigger cardiac arrest or acute myocardial infarction (AMI) in selected individuals.
This chapter reviews the physiologic basis and rationale for exercise therapy in patients with CHD, with specific reference to the liberalization of physical activity in the treatment of patients with AMI, cardiorespiratory adaptations to exercise training, fitness and mortality, cardioprotective effects of exercise, exercise prescription, exercise-related cardiovascular events, and strategies to increase patient compliance to exercise regimens.
EARLY CONVALESCENCE AFTER ACUTE MYOCARDIAL INFARCTION
Although in his classic cardiology text, Friedberg6 still recommended 2 to 3 weeks in bed and an additional period of 3 to 4 weeks in hospital for patients with AMI, prolonged bed rest is no longer recommended in the care of patients with acute cardiovascular events because of an abbreviated hospital stay. Moreover, extended bed rest has been shown to result in physiologic deconditioning and significant decreases in peak or maximal oxygen consumption (VO2 max), stroke volume, and cardiac output.7 Other adverse sequelae include increased muscle fatigue associated with reduced muscle blood flow, red cell volume, capillary growth, and oxidative enzymes; loss of muscle mass and bone density; constipation; urinary retention; thrombophlebitis; pulmonary embolism; hypostatic pneumonia; orthostatic intolerance; and depression.8
A seminal report by Levine and Lown,9 involving 81 patients with AMI who were subjected to “armchair treatment,” further prompted liberalization of activity restriction soon after an acute coronary event. Most patients were out of bed and sitting in a chair for up to 2 hours on their first day of hospitalization. Their time in the chair was progressively increased during hospital convalescence and most were encouraged to feed themselves and use the bedside commode or bathroom. Nearly all were discharged after 4 weeks. There were no complications attributed to the intervention, and the mortality rate of patients treated with chair rest, was lower than that of a control group who had received conventional therapy (e.g., bed rest). These findings have now been supported by numerous controlled clinical trials, providing convincing evidence that early mobilization and hospital discharge of patients with uncomplicated myocardial infarction is a safe practice that is associated with numerous physiologic, psychological, and economic benefits. Today, many uncomplicated postmyocardial infarction patients are being discharged from the hospital within 3 to 4 days.
Numerous studies now suggest that the lack of orthostatic stress is more important than physical inactivity in producing many of the deleterious effects of bed rest; consequently, interventions to simulate gravitational stress are widely used in preventing or attenuating these consequences. Convertino et al.10 studied changes in VO2 max before and after 14 days of bed rest using daily treatments with a reverse gradient garment that simulated the effects of standing. Aerobic capacity decreased only 6% in subjects who received venous pooling treatments, compared with a 15% decrease in nontreated (control) subjects (Table 36.1). These data have important practical implications for inpatient cardiac rehabilitation and early home convalescence.11 The loss of exposure to gravitational stress during the hospital stay contributes to the decrease in VO2 max after AMI, independent of related clinical variables (e.g., ejection fraction). Thus, it appears that the deterioration in VO2 max with bed rest may be lessened simply by regular exposure to orthostatic stress, such as intermittent sitting or standing. Structured, formalized in-hospital exercise programs after AMI appear to offer little additional physiologic or behavioral benefits over routine medical care.12
TABLE 36.1 Mean Changes in Maximal Oxygen Uptake (VO2 max) Before and After Bed Rest | |||||||||||||||||||||||
---|---|---|---|---|---|---|---|---|---|---|---|---|---|---|---|---|---|---|---|---|---|---|---|
|
CARDIORESPIRATORY ADAPTATIONS TO EXERCISE TRAINING
Regular endurance exercise training increases physical work (functional) capacity and provides relief of angina pectoris in many patients with CHD. These are particularly beneficial outcomes because many cardiac patients have a reduced functional or aerobic capacity (50% to 70% age, sex predicted), whereas others are limited by anginal chest pain at relatively low levels of effort. The postconditioning improvement in aerobic capacity appears to be mediated by increased central and/or peripheral oxygen transport and utilization, whereas relief of angina pectoris may result from a decreased rate-pressure product at rest and during any given level of submaximal exercise, increased oxygen delivery, or both.
Studies of physical conditioning in patients with CHD have generally demonstrated a 10% to 40% increase in preconditioning values of VO2 max; the wide variation in improvement is attributed to numerous factors affecting exercise trainability, including the patient’s age, clinical status, initial fitness, time from the acute cardiac event, the exercise prescription, and compliance to the conditioning program. Because a given submaximal task requires a relatively constant rate of oxygen uptake, expressed as METs, the cardiac patient finds that after an exercise training program, he/she is working at a lower percentage of his/her aerobic capacity, with a greater functional reserve (Figure 36.1).13
CARDIORESPIRATORY FITNESS AS A PREDICTOR OF MORTALITY
To examine the relationship between aerobic fitness and mortality in patients with documented cardiovascular disease (CVD), Vanhees et al.14 studied 527 men who were referred to an outpatient cardiac rehabilitation program. Peak oxygen uptake on a cycle ergometer was directly measured 12.9 ± 2.7 weeks after AMI (n = 312) or coronary artery bypass surgery (n = 215). During the average follow-up duration of 6.1 years, 33 and 20 patients died of cardiovascular and noncardiovascular causes, respectively. Those with the highest cardiovascular and all-cause mortality averaged ≤4.4 METs. In contrast, there were no deaths among patients who averaged ≥9.2 METs.
In a cohort of men (n = 6,213) reported by Myers et al.,15 3,679 with an abnormal exercise-test result and/or known CVD were referred for treadmill exercise testing. The average followup was 6.2 ± 3.7 years. Those with an exercise capacity of ≤4.9 METs had the highest relative risk of death, whereas those with a fitness level ≥10.7 METs had the lowest relative risk of death (4.1 and 1.0, respectively). For the total group, every 1-MET increase in exercise capacity conferred a 12% improvement in survival. Similarly, long-term findings from the National Exercise and Heart Disease Project among postmyocardial infarction patients demonstrated that every 1-MET increase in exercise capacity after a training period was associated with a reduction in mortality from any cause that ranged from 8% to 14% over the course of 19 years follow-up.16
Kavanagh et al.17,18 evaluated the predictive value of cardiopulmonary exercise testing in 12,169 men (55.0 ± 9.6 years) and 2,380 women (59.7 ± 9.5 years) with known CHD who were referred for exercise-based cardiac rehabilitation. The men and women were followed for an average of 7.9 and 6.1 years, respectively. Directly measured peak oxygen uptake on a cycle ergometer at program entry proved to be a powerful predictor of cardiovascular and all-cause mortality. The cutoff point, above which there was a marked benefit in prognosis, was 13 mL/kg/minute (3.7 METs) in women and 15 mL/kg/minute (4.3 METs) in men. For each 1 mL/kg/minute increase in exercise capacity, there was a 10% reduction of cardiac mortality in women versus 9% in men.
More recently, Dutcher et al.,19 using the well-described primary angioplasty in AMI (PAMI-2) database, reported that exercise capacity more accurately predicts 2- and 5-year mortality than does left ventricular ejection fraction in patients with ST-elevation myocardial infarction who were emergently treated with percutaneous coronary intervention. Those who had an exercise capacity ≥4 METs had better long-term survival; in contrast, those with an exercise capacity <4 METs were at a substantially increased risk of mortality, which was worsened in the presence of left ventricular dysfunction (ejection fraction <40%). The investigators concluded that exercise capacity was a better predictor of mortality than was left ventricular ejection fraction in this escalating patient subset. Accordingly, these data have important implications for the medical management and triaging of postmyocardial infarction patients who may benefit the most from an exercise-based cardiac rehabilitation program.
CARDIOPROTECTIVE EFFECTS OF EXERCISE
Two meta-analyses20,21 have now shown that regular exercise participation can decrease the overall risk of cardiovascular events by up to 50%, presumably from multiple mechanisms, including antiatherosclerotic, anti-ischemic, antiarrhythmic, antithrombotic, and psychological effects (Figure 36.2). Regular aerobic exercise can result in moderate losses in body weight, moderate-to-large losses in body fat, and small-to-moderate increases in lean body weight. Endurance exercise can promote decreases in blood pressure (particularly in hypertensives), total blood cholesterol, serum triglycerides, and low-density lipoprotein
cholesterol, and increases in the “antiatherogenic” high-density lipoprotein cholesterol subfraction. Moreover, exercise has favorable effects on glucose and insulin homeostasis, inflammatory markers (e.g., C-reactive protein), coagulability, fibrinolysis, and coronary endothelial function.22
cholesterol, and increases in the “antiatherogenic” high-density lipoprotein cholesterol subfraction. Moreover, exercise has favorable effects on glucose and insulin homeostasis, inflammatory markers (e.g., C-reactive protein), coagulability, fibrinolysis, and coronary endothelial function.22
Because >40% of the risk reduction associated with exercise cannot be explained by changes in conventional risk factors, Green et al.23 proposed a cardioprotective “vascular conditioning” effect, including enhanced nitric oxide vasodilator function, improved vascular reactivity, altered vascular structure, or combinations thereof. Decreased vulnerability to arrhythmias and increased resistance to ventricular fibrillation have also been postulated to reflect exercise-related adaptations in autonomic control. As a consequence of endurance training, sympathetic drive at rest is reduced and vagal tone is increased. Moreover, ischemic preconditioning before coronary occlusion, at least in animal models, can reduce subsequent infarct size and/or the potential for malignant ventricular arrhythmias.24,25
EXERCISE PRESCRIPTION
COMPONENTS
Structured exercise training sessions should include a preliminary aerobic warm-up (approximately 10 minutes), a continuous or accumulated conditioning phase (≥30 minutes or multiple 10 to 15 minutes exercise bouts), and a cool-down (5 to 10 minutes) followed by stretching activities. The warm-up facilitates the transition from rest to the conditioning phase, reducing the potential for ventricular ectopy and ischemic electrocardiographic (ECG) responses, which can occur with sudden strenuous exertion.26 A walking cool-down enhances venous return during recovery, decreasing the possibility of hypotension and related sequelae, and ameliorates the potential, deleterious effects of the postexercise rise in plasma catecholamines.27
MODALITIES/INTENSITIES
The most effective exercises for the conditioning phase include walking, jogging, stationary or outdoor cycling, swimming, rowing, stair climbing, and combined arm-leg ergometry. To improve cardiorespiratory fitness in the coronary patient, the threshold intensity for training should approximate 45% of the oxygen uptake reserve, which corresponds approximately to 69% of the highest heart rate achieved during peak or symptom-limited exercise testing.28 For ≥90% of the men and women participating in early outpatient cardiac rehabilitation programs, this intensity can be achieved by walking on a level surface at speeds of 2.9 to 3.3 miles per hour.29 Over time, the exercise intensity should be increased to 50% and up to 75% of the oxygen uptake reserve (or maximal heart rate reserve) to further increase aerobic fitness.
THE MET CONCEPT
The metabolic costs of many household, recreational/training (Table 36.2
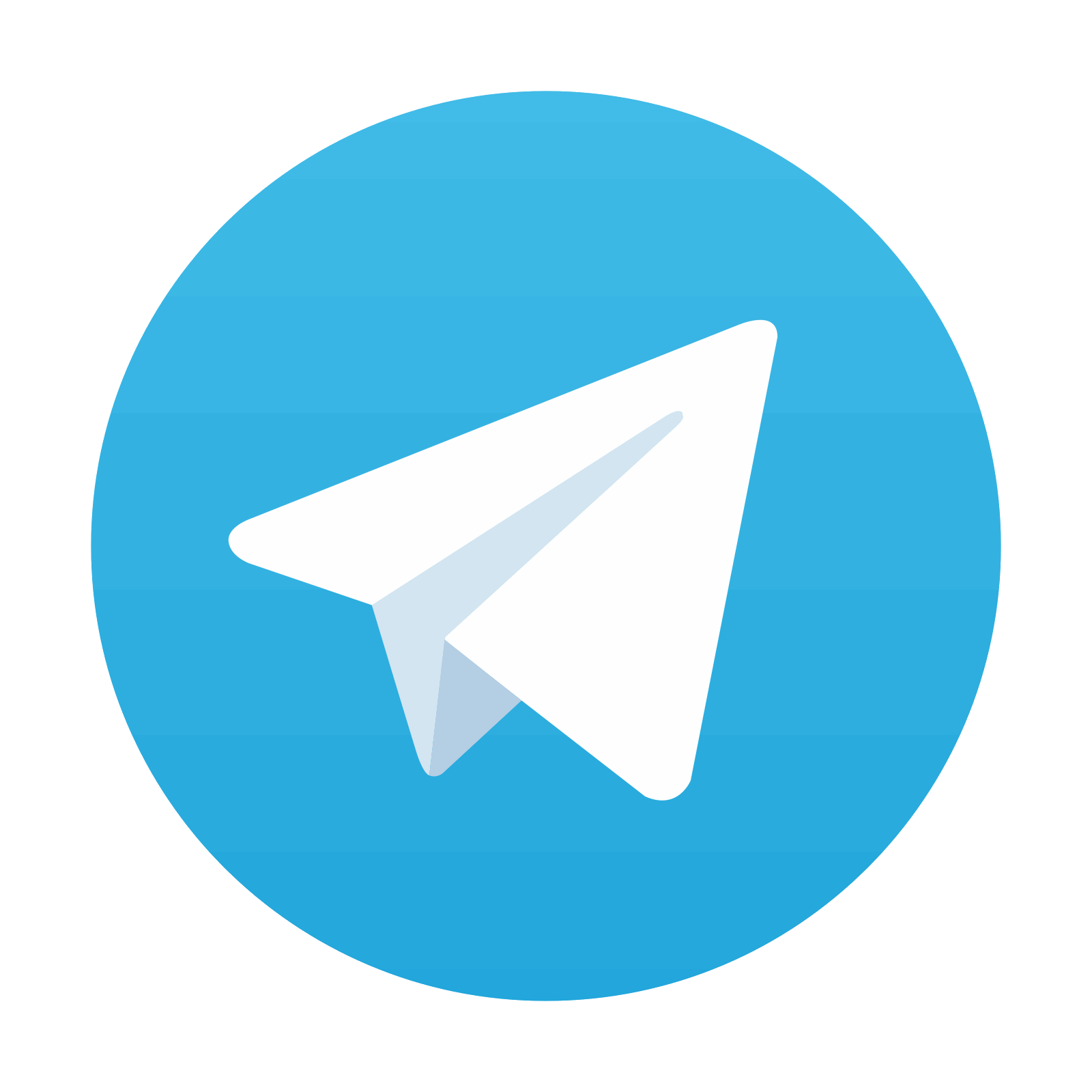
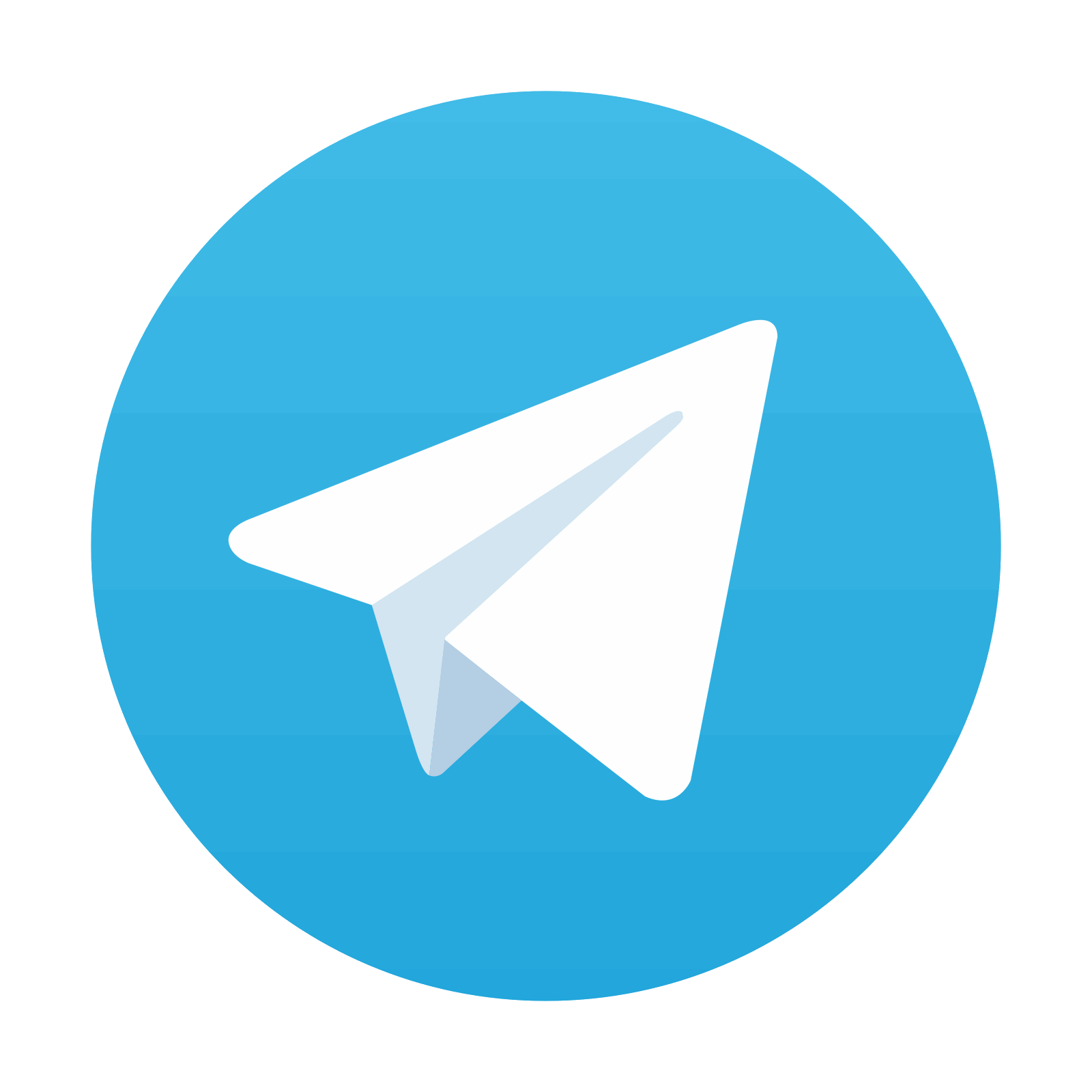
Stay updated, free articles. Join our Telegram channel

Full access? Get Clinical Tree
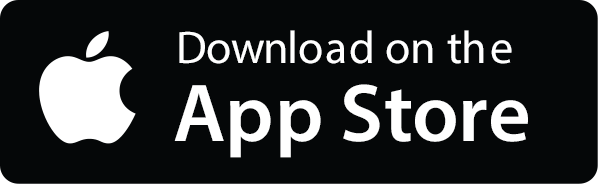
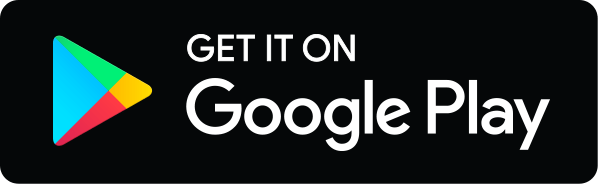
