Fig. 33.1
Mechanisms to augment cardiac output (C.O.) in (a) healthy persons and (b) Patients with heart failure VO2 = C.O. (HR × SV) (Reprinted from Pina et al. [4], © 2003, with permission from Wolters Kluwer Health)
In heart failure patients, decreased exercise capacity has similarly been attributed to a decreased cardiac output response which leads to skeletal muscle underperfusion and intramuscular lactic acidosis [1, 2]. This is based on observations that patients with heart failure exhibit reduced cardiac output responses to exercise compared to normal subjects. Additionally, there is a pronounced increase in filling pressures with the development of marked pulmonary hypertension with pulmonary capillary wedge pressures reaching as high as 50–60 mm Hg [2].
Exercise hemodynamic and ventilatory gas measurements during progressive treadmill exercise in patients with heart failure were first described by Karl Weber in 1981 [5]. This report demonstrated the usefulness of this technique as a non-invasive method for characterizing cardiac reserve and functional status. Weber demonstrated a significant correlation between cardiac output response and oxygen consumption and was able to classify patients into groups of worsening severity on the basis of this non-invasive technique. He found that with worsening heart failure the cardiac output response is markedly diminished. Several other studies have shown a significant correlation between the peak VO2 and cardiac output [6–8]. It is this correlation between peak VO2 and cardiac output which underlies the prognostic value of VO2 in HF leading to the widespread use of cardiopulmonary exercise testing in the evaluation of these patients.
Peripheral Factors
The peak cardiac output response to exercise is not the sole determinant of exercise performance in HF patients. Patients with similar reductions in left ventricular function estimated using ejection fraction have a wide range of exercise capacity [3]. Furthermore, therapeutic interventions aimed at acutely increasing cardiac output such as positive inotropic drugs do not significantly increase exercise capacity [9, 10]. The discrepancy between enhanced cardiac output and fixed exercise capacity can be explained by abnormalities of skeletal muscle and of the peripheral vasculature. Alterations of skeletal muscle metabolism and mass plays an important role in limiting peak functional capacity in HF patients [11–14]. The changes that occur in the skeletal muscle are critical in the genesis of the primary symptoms of heart failure (dyspnea and fatigue) (Fig. 33.2). Additionally as heart failure becomes progressive the compensatory response to this disease process further negatively impacts skeletal muscle function creating a downward spiral (Fig. 33.3).



Fig. 33.2
The muscle hypothesis is that the changes in the skeletal muscle result in reduced exercise capacity. Stimulation of ergoreceptors i.e. muscle nerve afferents sensitive to the amount of skeletal muscle work leads to the sensation of dyspnea and fatigue

Fig. 33.3
Downward spiral of worsening central cardiac function and increased sympathetic stimulation
CHF patients exhibit generalized skeletal muscle atrophy [11]. Muscle atrophy results from decreased protein synthesis, increased protein degradation or both. CHF is a catabolic state with deficiencies in several anabolic hormones [15–17]. In male CHF patients, deficiencies in circulating total testosterone and insulin-like growth factor are common and these decreases correlate with CHF severity [18]. Growth hormone (GH) resistance and reduction in skeletal muscle IGF-1 concentration contribute to skeletal muscle atrophy in HF by directly reducing protein synthesis [19]. Exercise training has been shown to increase local expression of IGF-1 in normal subjects and HF patients [20]. Patients with HF develop insulin resistance that is related to clinical events and mortality [21].
Chronic low level systemic inflammation, characteristic of the CHF state, also effect changes in skeletal muscle [22] and with the progression of CHF. Inflammatory mediators released into the circulation further activate systemic inflammation and promote muscle atrophy [23, 24].
In addition to skeletal muscle changes, the peripheral circulation undergoes substantial transformations during the progression of HF with an alteration of regional vascular control. These changes occur both at the level of the vascular endothelium and the vascular smooth muscle. Changes in capillary density have been reported in skeletal muscle of patients with HF. Capillary density has been reported to be normal or decreased [25, 26] depending on normalization to muscle fibers number and size. Aerobic training increases capillary density. Nitric oxide (NO)-mediated control of vasomotor tone in CHF [27–29] is attenuated representing endothelial dysfunction. Of note, aerobic training normalizes endothelial function in patients with HF probably via a mechanism of increased shear stress [30].
Finally, the respiratory muscles are also affected by chronic hypoperfusion and neurohormonal activation which occurs in heart failure [31]. Dyspnea is a complex sensation but weak respiratory muscles coupled with stiff congested lungs as occurs in HF predisposes patients to shortness of breath. Measurement of diaphragmatic work per breath demonstrates dramatic increases in patients with heart failure at rest and during exercise [32]. A reduction in inspiratory and expiratory respiratory muscle strength in patients with HF from both systolic and diastolic dysfunction has been shown [33, 34]. The endurance of the respiratory muscles in HF patients is also diminished compared to normal subjects [35]. The effect of selective respiratory muscle training on exertional dyspnea and exercise capacity has been examined in heart failure subjects. Respiratory muscle endurance, respiratory muscle strength, submaximal and maximal exercise capacity can be significantly improved with selective respiratory muscle training [36, 37].
In summary, exercise capacity is markedly reduced in most patients with CHF due to both a diminished cardiac output with exercise and to secondary changes in skeletal muscle, the peripheral circulation and the lungs. As noted above, most of the individual factors that contribute to reduced exercise capacity in CHF are improved by exercise training.
Exercise Performance and Prognosis in Patients with CHF
As discussed previously, Peak VO2 is derived from the Fick Principle and is the product of peak cardiac output and maximal arterio-venous oxygen difference. As most sedentary individuals will achieve comparable maximal arterio-venous difference, peak VO 2 provides an indirect assessment of cardiac output reserve. Several peripheral factors may also impact peak VO2, such as skeletal muscle mass and endothelial function, as well as age, gender and conditioning status.
The use of peak VO2 to predict prognosis in patients with HF was first described by Szlachcic [38]. In a prospective study of 114 ambulatory patients with severe CHF referred for cardiac transplantation, a VO2 of less than 14 mL/kg per minute was used as a criterion for acceptance for cardiac transplantation. One-year survival was 94 % in patients with a VO2 above 14 mL/kg/min. Accepted transplant candidates with a VO2 below 14 mL/kg per minute had a 1-year survival of 70 %, whereas the patients with a significant co-morbidity and reduced VO2 had a 1-year survival of 47 %. This approach permitted the identification of candidates whose transplant could be safely deferred [39].
Analysis of peak VO2 normalized by a predicted maximum based on age, obesity, and gender has been performed to determine if better prognostication can be achieved using percent of predicted peak VO2. Some investigators have suggested the superiority of this approach, though others have shown no clear benefit [40, 41]. Likely, the additional value of adjusting for sex, age and body composition in any study cohort is a function of how these characteristics are distributed across the cohort; in studies focusing on middle age men of average weight, both approaches yield similar results [40] while cohorts with greater heterogeneity would likely be better served by reference to sex and age specific prediction equations with adjustment for weight extremes [41].
Since the initial report of the value of peak VO2 in guiding transplant candidate selection in 1991, there have been many advances in the treatment of heart failure in particular, the use of beta blockade. This therapy has impacted significantly long-term survival without improving peak VO2. Whether VO2 retained its predictive power of in the beta-blocker era has been the subject of several reports [42–44]. Consistent across the reports was the sustained utility of this parameter in predicting survival whether cohorts were dichotomized by threshold values of above and below 14 ml/kg/min, or above and below 10 ml/kg/min. The survival for patients on beta blocking agents improved but nevertheless survival separated according to peak VO2. With the improved survival, a lower cut point than 14 ml/kg/min for referral or listing for cardiac transplant has generally been accepted, with the AHA/ACC guidelines now selecting a peak VO2 below 10 ml/kg/min with achievement of anaerobic threshold as an absolute indication for transplant (in the absence of significant contraindications). A peak VO2 of 11–14 ml/kg/min or 55 % of predicted peak VO2 resulting in major limitation of the patient’s daily activities is considered a relative indication for transplant listing [45].
During cardiopulmonary exercise testing other variables are collected that also confer prognostic information. Ventilatory efficiency during exercise, most frequently measured by the VE/VCO2 ratio or slope, has been found by several investigators to be even more predictive of outcome than peak VO2 [46–50]. The abnormal VE/VCO2 response results from increased ventilation–perfusion mismatching and heightened chemosensitivity and ergoreflex responses. This heightened ventilatory response occurs from the onset of exercise and thus unlike peak VO2, the VE/VCO2 relation does not require a maximal effort. VE/VCO2 >34 has been the cut-point selected in many studies but similar to peak VO2, this parameter is a continuous variable with no absolute cut-point. Frequently in studies, both peak VO2 and VE/VCO2 are found to have independent prognostic power, thus, the combination of both VE/VCO2 and peak VO2 may provide the strongest way to determine risk. Thus both peak VO2 and VE/VCO2 slope provide independent and complementary data on prognosis and should be used together to assess risk [46–50]. VE/VCO2 correlates more strongly with pulmonary pressures measured during exercise than does peak VO2. Exercise oscillatory breathing is associated with a poor prognosis. There is no uniform definition of this type of breathing but it is a periodic cycling of hyper and hypopnea with appropriate changes in PET O2 and PET CO2. This breathing pattern is observed in about 12–30 % of HF patients during exercise and most patients with exercise oscillatory breathing will have central sleep apnea. Presence of periodic breathing can predict mortality by itself or when combined with the ventilatory slope. In one study of 156 patients with HF, this breathing pattern was strongly correlated with sudden death [51, 52].
Other parameters measured during cardiopulmonary exercise testing also shown to have prognostic power in chronic heart failure include: blood pressure response to exercise (i.e. blunted or failure to increase BP with exercise associated with poor prognosis), the heart rate response to exercise (i.e. chronotropic incompetence), the ventilatory threshold, circulatory power (Peak VO2 × systolic BP), oxygen kinetics, end tidal Pco2 and oxygen recovery post exercise [45–53].
As we discussed, the prognostic value of peak VO2 has been presumed to be as a non-invasive indicator of peak cardiac output response to exercise. Exercise studies in HF patients using both metabolic carts and hemodynamic measurements from Swan Ganz catheters have shown that some measurements such as a reduced left ventricular stroke work index (LVSWI) at peak exercise to be a better prognostic indicator than peak VO2 [54–57]. As exercise hemodynamic studies are difficult to perform, other investigators have tried to derive other non-invasive parameters which would better approximate this variable. Cohen-Solal [53] proposed ‘peak circulatory power’ which is the product of the peak VO2 and the last systolic arterial pressure measurement. The data for this calculation is available from any cardiopulmonary exercise test without the need for special equipment. The value of the ‘circulatory power’ was assessed in a study involving 175 heart failure patients. During a 25 ± 10 month follow-up, 16 % died and 18 % underwent cardiac transplantation. Multivariable analysis demonstrated that the peak ‘circulatory power’ (chi-square = 19.9, P < 0.001) was the only variable predictive of death or need for transplant. When this was analyzed in terms of quartiles of peak VO2 or circulatory power, it appeared that prognosis was worse as peak VO2 declined, but that circulatory power aids in selecting subgroups with particularly poor prognosis—those with both reduced peak VO2 and reduced blood pressure.
The technology of metabolic carts has improved and new systems now permit non-invasive measurement of cardiac output using inert gas re-breathing techniques [58, 59]. Inert gas rebreathing is a novel, non-invasive method to measure cardiac output during exercise and is reliable, safe and easily performed in patients with CHF [59]. The Innocor rebreathing system uses an oxygen enriched mixture of an inert soluble gas (0.5 % nitrous oxide [N2O]) and an inert insoluble gas (0.1 % sulfur hexafluoride, [SF6]) N2O concentration decreases during the rebreathing maneuver, with a rate proportional to pulmonary blood flow. With the ability to measure cardiac output during exercise, cardiac power can then be derived. Cardiac Power incorporates blood pressure into the exercise hemodynamic assessment. It takes into account both the flow and pressure generating ability of the heart. Tan [60] has argued that it could be viewed as a comprehensive indicator of cardiac function. This technology was applied in 171 consecutive CHF patients during symptom limited bicycle exercise [59]. An accurate measure of peak CO was obtained in 148 patients (85 % of patients). Peak cardiac power was derived from the product of the peak mean arterial blood pressure and CO divided by 451. Duration of follow-up averaged 1 year. Univariable and multivariable analyses were performed using cardiopulmonary exercise variables (i.e., peak VO2, peak CO, peak cardiac power, VE/VCO2 slope, and VO2 at anaerobic threshold). Event-free survival for the entire cohort was 83 % with 5 deaths, 4 LVAD implants and 16 urgent transplants. In this cohort, peak VO2 was 12.9 ± 4.5 ml/kg/min and peak cardiac power was 1.7 ± 0.9 watts. Univariable predictors of adverse outcome were peak VO2, peak CO, peak cardiac power, VE/VCO2 slope and VO2 at anaerobic threshold. By multivariable analysis, peak cardiac power and peak CO were predictive of outcome with peak cardiac power being the most powerful independent predictor of outcome (P = 0.01).
As the population ages and more patients survive debilitating myocardial infarctions and/or previously untreatable cardiac disease, there is an ever increasing population of patients with heart failure. When patients approach Stage D, their therapeutic options become increasingly limited. Cardiac transplant and left ventricular assist devices provide (LVAD) the salvage therapies of these patients. With the limited donor supply for transplant, inevitably there will be an increasing number of chronic device patients. The exercise response of patients supported by mechanical assist device will become increasingly important. Despite mechanical support, the exercise capacity of device patients is significantly reduced [61, 62]. The exercise physiology of these patients is unique and results from both the central and peripheral effects of heart failure in conjunction with the limitations of the mechanical device. The right heart is unsupported by the commonly used left ventricular continuous flow pumps. Right ventricular dysfunction is one of the limiting factors for peak exercise performance in these patients particularly since the exercise response is primarily preload mediated. Unlike the earlier pulsatile left ventricular assist devices which had an automatic fill mode which ejected faster with an increase in pump filling, the continuous flow LVADs work with a fixed pump speed (8600–10,000) set with the patient at rest. The speed is selected to optimize unloading of the ventricle and to prevent excessive emptying that results in “suction” (hypotension, arrhythmias). The pump speed of these devices does not change with exercise such that the exercise response is primarily pre-load dependent. Maximum cardiac output of these devices is approximately 10–12 L/min. Additional cardiac output response during exercise can be provided by the native heart working in parallel with the mechanical device. Several long term complications associated with the new continuous flow devices such as anemia and the development of aortic valve leaflet fusion with or without aortic insufficiency can also impact exercise performance [63, 64].
Nevertheless, rest and exercise hemodynamic measurements in LVAD patients are improved compared to unsupported heart failure patients with lower pulmonary pressures and higher cardiac output [61, 62, 64]. Peak VO2 following support with a continuous flow LVAD averages 12–17 ml/kg/min. The largest study of Heartmate II patients (n = 18) revealed an average percent peak VO2 of 49 ± 19 % or 15.6 ± 4.7 ml/kg/min 3 months post insertion.
The 6 min walk test is a popularly used assessment of functional capacity in patients with heart failure. It is considered a measure of submaximal exercise performance and thus the level of function needed to perform the activities of daily living. The 6 min walk test, i.e., the distance walked over a period of 6 min, is less subjective than the NYHA functional class, but still can be heavily influenced by the patient’s and/or tester’s motivation. The 6 min walk test has been shown to provide prognostic information by the Study of Left Ventricular Dysfunction (SOLVD) investigators who demonstrated in a sub-study of 898 HF patients in their registry that mortality risk was 3.7 times higher in those patients with a 6 min walk distance <350 meters compared to those who walked >450 m. Similarly, the risk of HF hospitalization was 1.4 times higher in those with reduced walk distance [65]. Given the limitations of the current LVADs, the 6 min walk test rather than peak VO2 has been increasingly used to assess the therapeutic response to mechanical support.
Benefits of Exercise for the Treatment of Chronic Heart Failure
Histologic and metabolic changes of skeletal muscle in HF as described earlier in this chapter may be partially due to muscle disuse [11, 66]. Exercise is a physiologic intervention with a broad variety of positive cardiovascular effects that include changes in lipid metabolism, insulin resistance, weight, arterial hypertension, inflammation and mood [67]. Effects of aerobic exercise on the myocardium have been well established. Regular dynamic exercise increases stroke volume, cardiac output and reduces beta-adrenergic stimulation. Exercise training increases myocardial mass, left ventricular dimensions and stroke volume in healthy subjects [68]. In chronic heart failure, exercise has been shown to improve exercise tolerance and symptoms which is attributed to peripheral adaptations such as improved endothelial function and skeletal muscle strengthening [13].
Many of the central and peripheral changes induced by aerobic training may have a marked therapeutic effect in patients with heart failure. Potential advantages of training in HF (Table 33.1) include central hemodynamic changes such as an increase in stroke volume, and a potential increase in contractility, alteration of autonomic tone with a decrease in sympathetic stimulation, an improvement in endothelial and vascular function with a decrease in peripheral vascular resistance, muscle enzymatic changes with an increased oxidative capacity and decrease in lactate production.
Table 33.1
Benefits of aerobic training
Morphologic | ↑ Myocardial mass |
↑ Left ventricular end diastolic volume | |
↑ Diameter of coronary arteries | |
↑ Myocardial & Skeletal muscle capillary to fiber ratio | |
Skeletal muscle | ↑ Capillary density |
↑ Mitochondrial volume and cristae | |
↑ Enzymes citric acid cycle and electron transport chain | |
±↑ Myoglobin | |
↑ Use of free fatty acids | |
↑ Potential to store glycogen | |
↑ Local A-VO2 difference | |
↑ Maximal flow rate through muscle | |
Hemodynamic | ↓ Resting HR |
↓ Double product at submaximal workloads | |
↑ Stroke volume | |
↑ Maximum cardiac output | |
↑ Peak VO2 | |
Metabolic | ↑ HDL |
↓ Triglycerides | |
↓ Fasting glucose | |
↓ Catecholamines | |
↑ Lipoprotein lipase | |
↓ Hepatic lipase (converts HDL2 HDL3) | |
↑ LCAT (esterifies chol with FFA, enhancing chol transport) | |
↑ βeta hydroxacyl Co dehydrogenase (↑ βeta oxidation FFA) |
Animal models have been used to investigate the effects of exercise training on the failing heart. Using an ischemic rat model of HF, Musch [69] studied the effects of endurance training. The training protocol consisted of 60 min sessions of treadmill exercise 5 days a week for 10–12 weeks. Following the training program in the rats with heart failure, VO2 was higher, succinate dehydrogenase activity increased and lactate levels were lower during submaximal exercise. No differences were observed in regional perfusion or in hemodynamic measurements. In this rat model of heart failure, all derived benefits of training were from peripheral mechanisms. Central cardiac function was not altered. Todaka [70] using a pacing-induced model of HF in dogs demonstrated both central and peripheral effects of exercise training. One group received daily treadmill exercise (4.4 km/h, 2 h/day) while the other dogs remained sedentary. At 4 weeks, in-vivo hemodynamic measurements revealed relative preservation of maximum rate of pressure rise and left ventricular end-diastolic pressure in the exercised compared with the sedentary dogs. Subsequent in-vitro analysis of cardiac function revealed similarly depressed systolic functions in both groups. However, whereas the diastolic myocardial stiffness constant was elevated in the sedentary group, it was normal in the exercise training group (32 ± 3 in sedentary dogs, 21 ± 3 in exercised dogs, 20 ± 4 in otherwise normal dogs). Thus, daily exercise training preserved in-vivo hemodynamics and in-vitro measures of diastolic stiffness. The authors concluded that changes in heart function may contribute to the overall beneficial hemodynamic effects of exercise training in this canine model of CHF by a significant effect on diastolic properties.
In patients with CHF, a wide range of hemodynamic changes observed in response to exercise training may also impact on reverse remodeling [13, 14, 67]. One study reported an increase in peak cardiac output [13]. The same workload is achieved at a lower heart rate and rate-pressure product [13], indicating a more efficient utilization of myocardial work and oxygen consumption. Several studies have attempted to characterize the physiologic mechanisms for the clinical improvement in patients with HF. Sullivan studied 12 patients with HF with a mean VO2 of 16.3 ml/kg/min and an ejection fraction 21 % [71]. Aerobic training was performed 3–5 h a week for 6 months. With exercise training, peak VO2 rose 23 % from 16.8 to 20.6 ml/kg/min. Peak cardiac output, peak A-VO2 difference and peak leg blood flow also significantly increased but ejection fraction was unchanged. Training decreased leg lactate production. Leg blood flow during submaximal exercise did not increase suggesting that the major benefit derived from training was via increased oxygen extraction by the skeletal muscles and the largest proportion of the increase in VO2 was derived from peripheral adaptation with a possible small central contribution.
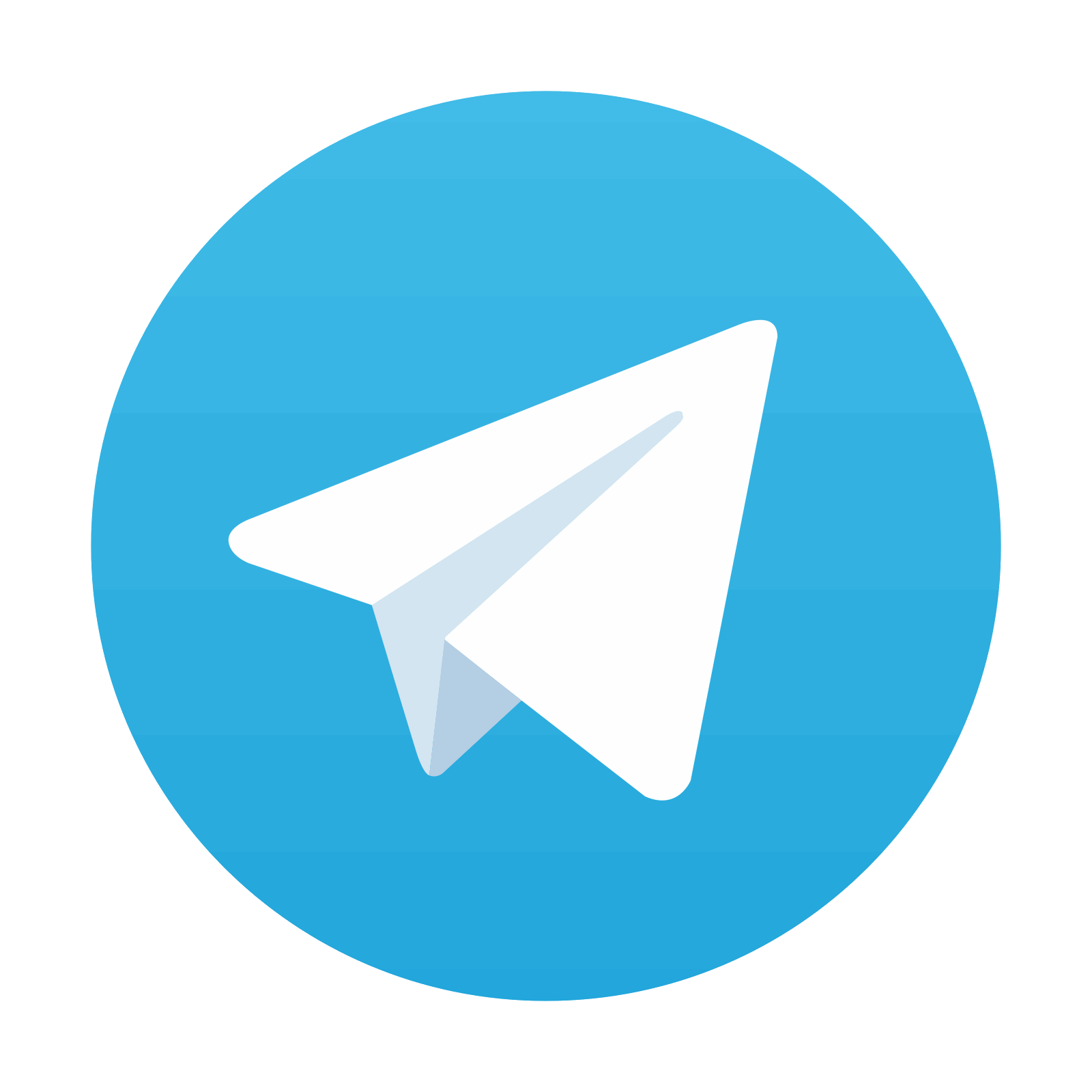
Stay updated, free articles. Join our Telegram channel

Full access? Get Clinical Tree
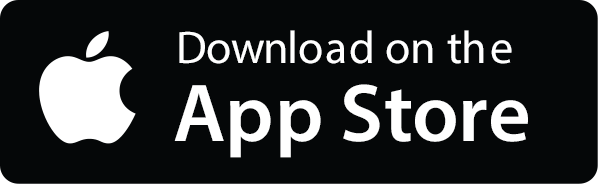
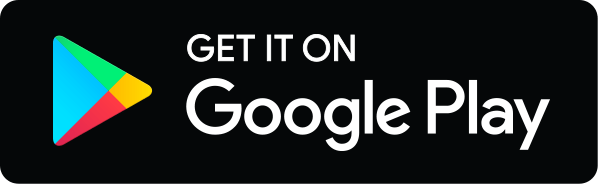