Lung transplantation is a life-saving therapy for patients suffering from end-stage lung diseases. The number of patients waiting for lung transplantation, however, greatly exceeds the number of donor lungs available. Moreover, only about 15% of lungs from multiorgan donors are deemed usable for transplantation.1 Most potential lungs are considered unsuitable as the result of injury that occurs with brain death and ICU-related complications (i.e., barotrauma or lung edema associated with fluid resuscitation). Since primary graft dysfunction leads to severe early and long-term consequences for lung transplant recipients, transplant teams tend to be very conservative in their selection of donor lungs. As a result, fewer organs are selected and the wait list mortality may climb as high as 30% to 40%.2,3 While the current cornerstone of clinical lung preservation is to limit the metabolic rate of the donor lung by hypothermia, this strategy best serves lungs meeting ideal acceptance criteria. The current donor organ shortage has prompted most donor programs to use increasing numbers of extended criteria organs, where lung function is not as assured as with an ideal lung. The ex vivo phase of organ preservation, prior transplantation into the recipient, provides a window of opportunity for further evaluation and even resuscitation of these compromised lungs. To take advantage of this opportunity, however, it is necessary to preserve the donor organs under normothermic or near-normothermic conditions. One strategy, termed ex vivo lung perfusion (EVLP), attempts to simulate in vivo conditions through ventilation and perfusion of the donor lung graft. One important advantage of normothermic perfusion is that it maintains the active metabolic functions of the lung, providing an opportunity for continued assessment of the organ during the ex vivo phase of organ preservation, active treatments, and restoration of normal function. This chapter focuses on the resurgence of EVLP, important technical aspects, clinical outcomes, and future perspectives.
Originally proposed in 1938 by Carrel for organs in general and then in 1970 by Jirsch et al. for the evaluation and preservation of lungs in cases of distant procurement, attempts at EVLP in those eras failed because of an inability to maintain the air/fluid barrier integrity within the lung, which led to the development of edema and increased pulmonary vascular resistance in the donor lung.4,5 For most of the past century, EVLP systems were used in small animal studies of lung physiology. More recently, normothermic perfusion became a research focus as a preservation alternative in experimental models of lung, liver, and kidney transplantation.6–11
The resurgence of EVLP as a potential tool in lung transplantation started with the work of Steen et al.12 This group described EVLP as a method to reassess lungs after uncontrolled donation from cardiac death donors (DCD),13 since these organs cannot be evaluated in vivo. A lung perfusion specific solution (Steen® solution) with optimal osmolarity and high dextran content was developed by this group.12,14 After Steen’s publications, other groups demonstrated the feasibility of blood-based short-term EVLP (30–90 minutes) to evaluate lung function in animal models of donation after cardiac death and experimentally using injured human lungs deemed unsuitable for transplantation.15–21 However, even in this most current era of EVLP, attempts to perfuse ex vivo lungs for greater than 2 hours have largely failed, as extended perfusion times lead to the development of pulmonary edema and an increase in the pulmonary vascular resistance meaning that the system, itself, inflicts some degree of injury.22
With the vision that ex vivo treatment and repair of injured organs would, in general, require an ex vivo period lasting longer than 2 hours, we developed a protective EVLP system and strategy with the goal of maintaining lungs in the EVLP system for at least 12 hours without causing additional injury to the lungs (currently known as the Toronto technique of EVLP, see details below). Several important modifications were made to achieve 12 hours of perfusion stability. These included (1) an acellular perfusate, (2) a closed circuit with protective low perfusion pressure (pulmonary artery pressure [PAP] 10–13 mm Hg) and stable positive left atrial (LA) pressure (5 mm Hg), and (3) a protective mode of mechanical ventilation (tidal volume of 7 mL/kg, rate of 7 breaths per minute [bpm], with a positive end-expiratory airway pressure [PEEP] of 5 cmH2O). In our early work with normal pig lungs, lung function was stable during 12 hours of normothermic EVLP.23 This stability during prolonged EVLP translated into excellent posttransplant lung function, absence of edema formation, and preserved lung histology after transplantation. The acellular perfusion assessment of lung function accurately correlated with posttransplant graft function, and the addition of red blood cells did not provide additional functional information compared to acellular perfusate alone.23 This study provided the proof of concept that EVLP is able to maintain normal donor lungs for a prolonged period of time without damaging the organ. Further examination was then performed to determine the impact of prolonged EVLP using injured ischemic donor lungs.24 Pig donor lungs were cold preserved for 12 hours and subsequently divided into two groups: cold static preservation (CSP) or normothermic EVLP for a further 12 hours (total 24 hours of preservation). EVLP preservation resulted in significantly better lung oxygenation and lower edema formation rates after transplantation when compared to CSP. Alveolar epithelial cell tight junction integrity, evaluated by zona occludens-1 protein staining, was disrupted in the cell membranes after prolonged CSP but not after EVLP.
The components and set up of the circuit are demonstrated in Fig. 172-1. The EVLP perfusion and ventilation strategy is shown in Table 172-1. The preparation of the donor lungs occurs on the back table with the lungs immersed in a CSP solution. Specifically designed funnel-shaped cannulas with built in pressure sensors (XVIVO cannulas, Vitrolife) are attached to the LA and pulmonary artery (PA). The lungs are then transferred from the back table to the XVIVO chamber. The PA cannula is connected to the circuit and anterograde flow (PA–LA) is initiated at 150 mL/min with the perfusate at room temperature. The temperature of the perfusate is then gradually increased to 37°C over the next 30 minutes. Before increasing flow beyond this level, a careful check of the system is made. The PA and LA pressure readings are double-checked. When a temperature of 32 to 34°C is reached (usually over 20 minutes), ventilation is started and the perfusate flow rate is gradually increased to the target flow (40% of estimated donor cardiac output) within 60 minutes. Once ventilation is started, the flow of gas (86%N2, 6%O2, 8%CO2, Praxair) that will deoxygenate and provide carbon dioxide to the inflow perfusate via the gas exchange membrane is initiated (started at 1 L/min) and titrated to maintain inflow perfusate pCO2 between 35 and 45 mm Hg. Steroids, antibiotics, and heparin are added to the perfusate prior to EVLP initiation. Recruitment lung maneuvers are performed every hour to an airway peak pressure of 25 cmH2O. Ex vivo bronchoscopic examination and x-rays of the lungs are performed every 2 hours. Every 2 hours, 250 cc of perfusion solution is exchanged for a fresh perfusate.
Figure 172-1
Components of ex vivo lung perfusion circuit. The perfusate is circulated by a centrifugal pump passing through a membrane gas exchanger and a leukocyte-depletion filter before entering the lung block through the pulmonary artery. A filtered gas line for the gas-exchange membrane is connected to an H-size tank with a specialty gas mixture of oxygen (6%), carbon dioxide (8%), and nitrogen (86%). A heat exchanger is connected to the membrane gas exchanger to maintain the perfusate at temperature. Pulmonary artery flow is controlled by the centrifugal pump and measured using an electromagnetic flow meter. The outflow oxygenated perfusate returns through the left atrial cannula to a hard-shell reservoir. Lungs are ventilated with a standard ICU-type ventilator. The lungs are contained in a specifically designed lung enclosure (XVIVO, Vitrolife).

Ventilation | |
Tidal volume | 7 mL/kg |
PEEP | 5 cm H2O |
Frequency | 7 breaths per minute |
I/E ratio | 1/2 |
Recruitment | 1 every hour to PawP 20 cm H2O |
Perfusion | |
Pump flow | 40% estimated donor CO |
Pulmonary artery pressure | 7–13 mm Hg |
Left atrial pressure | 3–5 mm Hg |
Perfusate exchange | 250 cc every hour |
Perfusate composition | Steen solution, heparin, antibiotics, solumedrol |
Perfusate pH | 6.8–7.4 |
Perfusate pCO2 | 35–45 mm Hg |
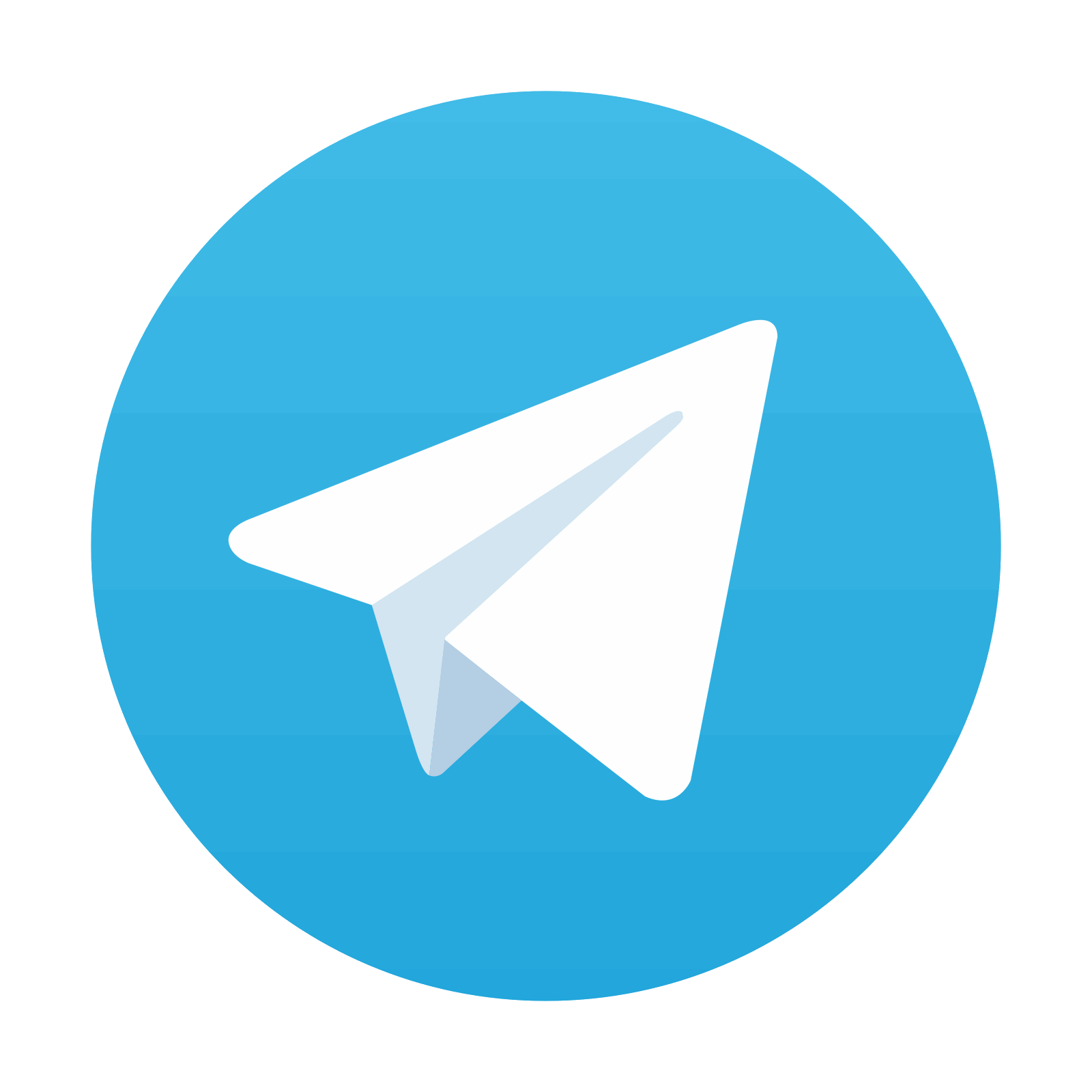
Stay updated, free articles. Join our Telegram channel

Full access? Get Clinical Tree
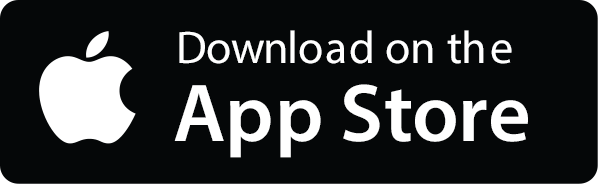
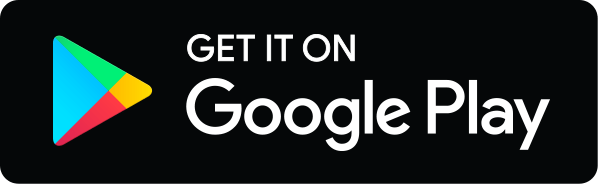
