Fig. 10.1
The evolutionarily conserved building plan of the heart. (a) All vertebrate embryos form a heart tube with slow propagating peristaltic contractions reflected in a sinusoid ECG. (b) Atrial and ventricular compartments with a distinct molecular phenotype balloon out (blue) and due to faster propagation in these compartments the electrocardiogram (ECG) acquires an adult-like profile. Full ventricular septation in mammals and birds coincides with a primary ring that expresses the Bmp2/Tbx3 pathway. (c) The configuration of b is essentially the scaffold of the adult fish heart, except there is no primary ring, and even resembles the systemic side of the formed human heart. (d) The atrioventricular conduction axis is established in the embryo with a slow propagating atrioventricular canal (red) and a fast propagating trabeculated ventricle (blue) with a thin compact wall (light grey). This design is maintained in ectothermic vertebrates and modified in birds and mammals by the presence of the ventricular septum, thickened ventricular walls and an insulating plane (ip) (Modified from Jensen et al. [5])
The division of the embryonic heart into fast and slow propagating chambers and junctions, respectively, can give rise to an almost adult-like ECG with distinct P, QRS and T waves [6]. Similar regions are present in the adult hearts of fishes and amphibians, except the most basal vertebrates (hagfishes) where a myocardial outflow tract is absent and most teleost bony fishes, e.g. the zebrafish, where the myocardial outflow tract is feebly developed and the cardiac inflow is almost devoid of myocardium [9].
Differentiation of the working myocardium relies on broadly expressed transcription factors, like GATA binding protein 4 (Gata4) and NK2 homeobox 5 transcription factor (Nkx2-5), and T-box transcription factors Tbx5 and Tbx20 that are expressed in gradients from inflow to outflow and outflow to inflow, respectively [10]. The remaining heart tube myocardium escapes differentiation into working myocardium due to transcriptional repression. The repressive action of the pathway of bone morphogenetic protein 2/4 (Bmp2/4)-Tbx2/3 appears very important [11] and is regulated upstream by the signalling pathways of Notch [12] and Wnt/β-catenin [13] and activated by co-localization with Gata4 [14] (Fig. 10.1b). This model of transcriptional regulation of chamber formation has largely been unravelled in mechanistic studies in mice, but we and others have shown a similar pattern of expression of transcription factors in all vertebrate classes investigated, ranging from lampreys, bony fishes, amphibians, reptiles, birds to mammals [15, 16]. During further development of mammals and birds, the non-chamber regions may acquire the phenotypes of the cardiac conduction system, and Tbx3 is expressed in the sinus node, the atrioventricular node and the His bundle and its bundle branches [17] and functions in a very dosage-sensitive manner [18]. The Purkinje fibres, the terminal branches of the ventricular conduction system, share the molecular phenotype of the trabeculations of the embryonic ventricle and the fully formed ventricle of the ectotherms (Fig. 10.1d) [16]. The cardiac conduction system, thus, is built on the old scaffold of the vertebrate heart, and this may explain the great similarity in the ECGs from very different vertebrates and developmental stages (see Chap. 8).
10.3 Evolution of Cardiac Septation
In mammals and birds, the early atrial and ventricular septa have a mesenchymal cap that expresses the Bmp2/Tbx3 pathway [17]. This mesenchyme is continuous with the mesenchymal cushions of the atrioventricular canal and the so-called dorsal mesenchymal protrusion (or spina vestibuli of His the elder) that harbours the orifice of the pulmonary vein and contributes to the completion of atrial septation (Fig. 10.2b) [19, 20] (see Part VI). It is remarkable that a very similar mesenchymal configuration can be found in the heart of lungfishes (Fig. 10.2c–e). Benninghoff [21] called this mesenchyme a ‘Leitbahn’, or guide, for septation. Lungfishes are the extant vertebrates that resemble the tetrapod ancestors the most. They are also the only fishes where the heart displays partial septation of the atrium, ventricle and outflow. Studies on lungfish cardiogenesis, however, are experiencing a century-long hiatus and have to be extended with modern approaches [22–24]. A prominent ventricular septum of trabeculated myocardium develops in the lungfishes of Africa and South America (Fig. 10.2e), but not in the heart of the Australian lungfish, which nonetheless has a pronounced mesenchymal guide in development (Fig. 10.2d). A complete ventricular septum, including a membranous septum, is found only in mammals, crocodilians and birds (Fig. 10.2a) [25]. Comparing these groups, it appears that ventricular septum formation relies on firstly ballooning and secondly condensation of trabeculations (see Part V). In placental mammals complete atrial septation necessitates the fusion of the first and second atrial septum (see Part IV), whereas reptiles and birds simply develop a single perforated atrial septum that closes around hatching [25].
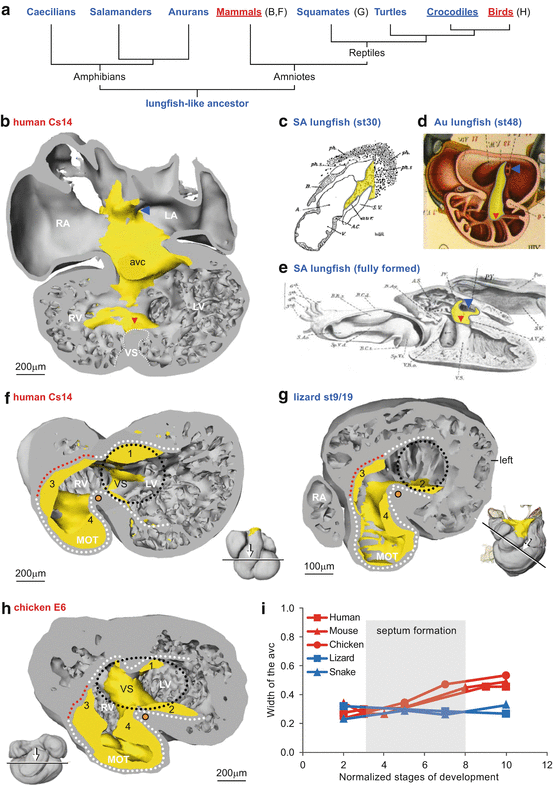
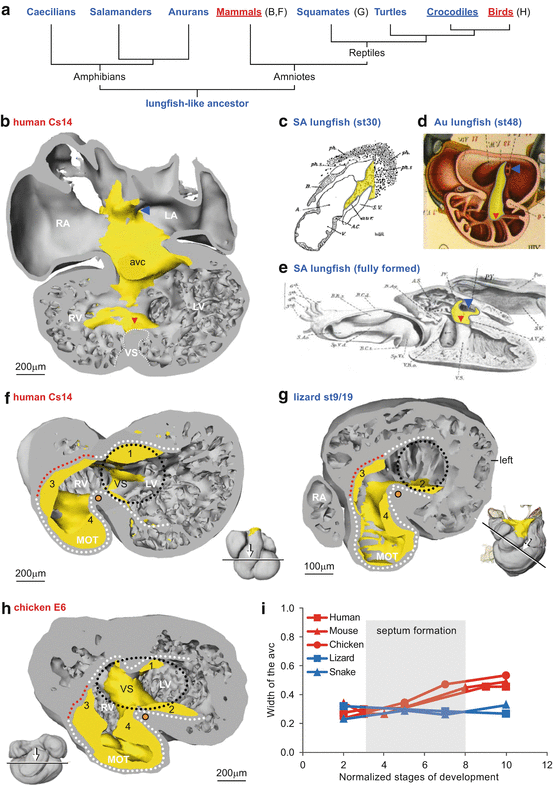
Fig. 10.2
Mesenchyme reflects the embryonic cardiac building plan. (a) Phylogeny of tetrapods with ectotherms in blue and endotherms in red, and underlined animals have complete ventricular septation. (b) The dorsal half of the embryonic human heart with mesenchyme (yellow) associated with the orifice of the pulmonary vein (blue arrowhead), atrial septum, cushions of the atrioventricular canal (avc) and the crest of the ventricular septum (red arrowhead). (c) In the embryonic South American lungfish, a dorsal mesenchymal protrusion harbouring the pulmonary vein precursors enters the atrium. (d) The cardiac mesenchyme in the embryo of the basal Australian lungfish associates with the orifice of the pulmonary vein, atrial septum, cushion of the atrioventricular canal and ventricular trabeculations (red arrowhead). (e) The right half of the fully formed heart of the South American lungfish showing the guiding mesenchyme associated with the orifice of the pulmonary vein, the perforated atrial septum (AS) and the crest of the ventricular septum (VS). (f–g) In early embryos of all amniote vertebrates, the atrioventricular canal (outlined by black dots) is to the left of the bulboventricular fold (orange dot) as exemplified by the human (f) and lizard (g). (h) In later embryonic stages of species with complete ventricular septation, exemplified by the chicken, the atrioventricular canal will expand to the right of the bulboventricular fold. (i) Quantification of the width of the atrioventricular canal. Each point is one measurement from a ‘four-chamber’ section of the maximal width (diameter) of the atrioventricular canal and ventricle. Stage 2 is at the onset of ventricular trabeculation; stages 3–8 encompass initiation and completion of ventricular septation in the endotherms (c, e are modified from Robertson [22], and d is modified from Greil [23])
When ventricular septum formation commences in mammals and birds, the atrioventricular canal is positioned on the left of the body midline as in reptiles (Fig. 10.2f–g). Soon after, the atrioventricular canal expands and shifts rightwards so that the right atrium remains in communication with the right ventricle (Fig. 10.2h). This process does not occur in ectothermic vertebrates (crocodilians excluded) [25]. Accordingly, the width of the atrioventricular canal almost doubles in mammals and birds, but not in the incompletely septated reptile hearts (Fig. 10.2i). Congenital malformations of the human heart like double-inlet left ventricle and tricuspid dysplasia and atresia may be considered as an incomplete rightward shift and expansion (see also Part XIV). Indeed, tricuspid atresia is not confined to man and has been reported in multiple mammalian species [26]. It is therefore crucial to know the mechanisms and forces that underlie the rightward shift and expansion of the atrioventricular canal in animals with complete ventricular septation. In formed crocodiles and birds, the mural leaflet of the right atrioventricular junction is largely myocardial and in mammals it is only late in gestation that the mural leaflet loses its myocardium and is exclusively composed of connective tissue. In Ebstein’s anomaly, which has also been reported for multiple mammalian species [26], the mural leaflet has persisting myocardium [27] (see Part XV).
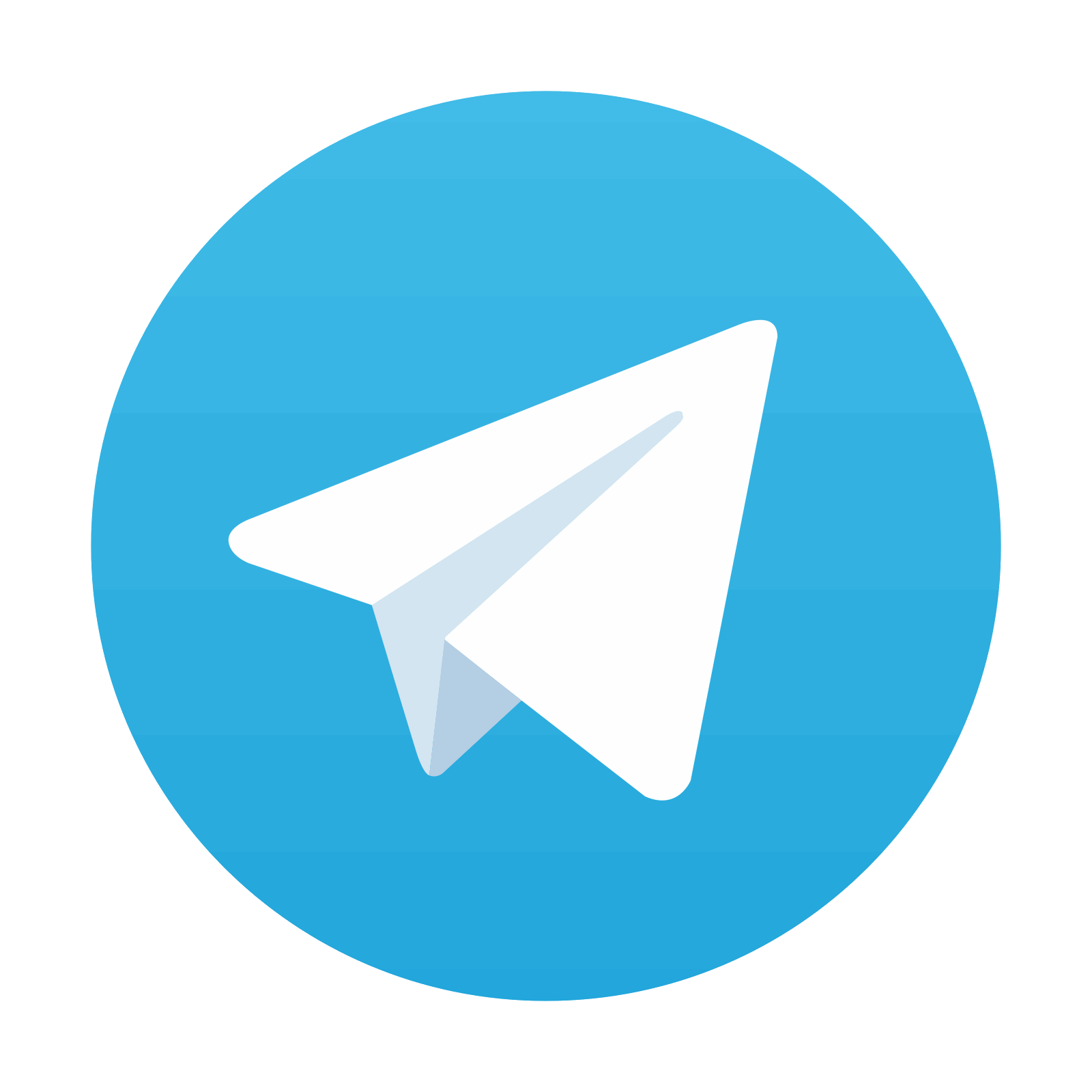
Stay updated, free articles. Join our Telegram channel

Full access? Get Clinical Tree
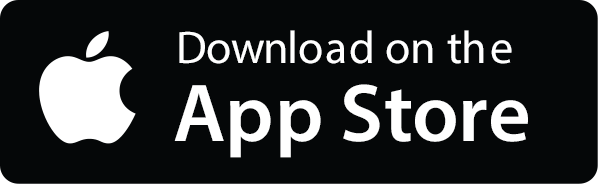
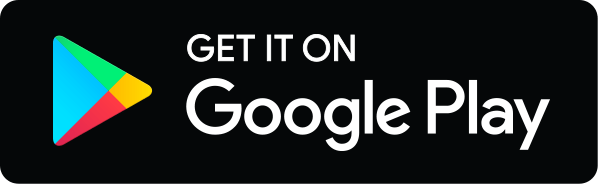