Fig. 10.1
Hypoplastic left heart syndrome, as viewed from the mid esophageal four chamber view. There can be significant anatomic variation ranging from mitral stenosis and left ventricle hypoplasia (a) to virtual absence of these structures (b). The atrial septum can be adequately visualized and interrogated by color Doppler also in this plane (c). The mid esophageal long axis view (d) allows for visualization of the aortic valve and diminutive proximal ascending aorta (arrow). LA left atrium, LV left ventricle, RA right atrium, RV right ventricle
Hypoplastic Right Heart Syndrome
Hypoplastic right heart syndrome encompasses a spectrum of cardiac lesions characterized by pulmonary atresia (either a formed but imperforate pulmonary valve, also known as membranous atresia, or long-segment muscular atresia), varying degrees of RV hypoplasia, and an intact ventricular septum. This class of cardiac defects is often referred to as pulmonary atresia/intact ventricular septum. The tricuspid valve is patent but hypoplastic, with the degree of tricuspid hypoplasia generally correlating with the extent of RV hypoplasia. In moderate hypoplasia, only two major portions of the RV (inflow and outflow) are present; the apical (trabecular) portion is either truncated or absent. This is termed a “bipartite” RV. In the most severe cases, only a tiny RV (usually the inflow portion) is present and this is termed a “unipartite” RV. Such patients have an obligate right to left shunt across the atrial septum, and a good-sized LV and aortic valve. Given the absence of antegrade flow from RV to pulmonary artery, patients require an alternate source of pulmonary blood flow, usually from a patent ductus arteriosus (PDA). As RV hypoplasia becomes more severe, there is an increasing likelihood of persistent embryonic sinusoidal connections (also known as ventriculo-coronary arterial communications) between the RV and the epicardial coronary arteries [8]. Some of these sinusoidal connections can contain stenoses that result in portions of the myocardium being perfused primarily or exclusively by the hypertensive RV; this situation is termed RV dependent coronary artery perfusion. In such cases, a two ventricle repair cannot even be considered, as the RV cannot be decompressed [9].
In the absence of RV-dependent coronary circulation, a determination must be made whether a hypoplastic right heart syndrome patient is a candidate for a two ventricle repair, or whether the patient should undergo single ventricle palliation. This determination is largely based upon an assessment of the degree of RV hypoplasia, which in turn is based primarily upon an evaluation of tricuspid valve annulus and RV size. The minimum tricuspid and RV dimensions that dictate the choice of one vs. two ventricle strategy vary among different institutions, and the published criteria continue to be evolve with time and increasing experience. Some centers use a tricuspid valve annulus z score anywhere between <−3 to −10 as a cutoff [10–13], some use the RV morphology (unipartite vs. bi/tripartite) [14], some use an estimation of RV diastolic volume [15], and some use a combination of these criteria. Whatever the method, if the tricuspid valve and RV are deemed too hypoplastic for a two ventricle circulation, then initial surgery is undertaken with the intention of single ventricle palliation—specifically, planned future bidirectional Glenn and Fontan procedures (discussed below). In this case, a systemic-pulmonary artery shunt (Blalock-Taussig shunt) is initially performed, often in conjunction with an atrial septectomy to assure unobstructed mixing of right and left atrial blood. In patients with milder forms of tricuspid valve and RV hypoplasia, a two ventricle (vs. “one and a half”) approach can be considered. In these instances antegrade flow across the RV outflow tract is established in the newborn period, with the expectation that the increased blood flow through the RV will stimulate growth of this chamber. This can be accomplished surgically by reconstruction of the RV outflow tract, usually performed in combination with a systemic-pulmonary shunt (such as a Blalock-Taussig shunt) [16]. An alternative strategy utilizes interventional catheterization techniques to establish antegrade flow across the RV outflow tract, including catheter perforation of the pulmonary valve (using balloon, laser, or radiofrequency methods), along with transcatheter stenting of the ductus arteriosus [14, 17]. With either surgical or transcatheter approach, some patients experience enough growth of their RV that they can function effectively with two ventricle physiology [14, 18]. Eventually, these patients can undergo closure of the systemic-pulmonary shunt and, if an atrial defect is still present, it can also be closed. In other patients, the RV continues to be moderately hypoplastic and a “one and a half repair” is subsequently undertaken. In this type of operation, a bidirectional Glenn procedure (discussed below) is performed to allow SVC blood to drain directly to the pulmonary arteries, while leaving inferior vena cava (IVC) blood to return to the RV and then be ejected directly into the main and branch pulmonary arteries. At the time of the Glenn operation, the atrial septal defect, if present, is often closed [19].
The TEE evaluation of the patient with hypoplastic right heart syndrome focuses on the adequacy of an interatrial communication, as well as the degree of tricuspid valve and RV hypoplasia. The atrial septum can be assessed from the ME 4 Ch and mid esophageal bicaval (ME Bicaval) views. The tricuspid valve and hypoplastic RV are best seen using a combination of MV 4 Ch (Fig. 10.2a, Video 10.2) and mid esophageal right ventricle inflow-outflow (ME RV In-Out) views (Fig. 10.2b, c, Video 10.2). Tricuspid and pulmonary valve annular diameters can also be measured using these views; as mentioned above, the tricuspid valve annulus diameter plays a very important role in determining whether intervention should be undertaken for a one or two ventricle strategy [15, 19, 20]. Coronary artery sinusoidal flow can sometimes be seen with color flow Doppler, but coronary artery stenoses cannot be diagnosed by echocardiography and therefore must be evaluated by angiography. Left ventricular and mitral valve function should be evaluated carefully, also from the midesophageal views, specifically the ME 4 Ch, mid esophageal two chamber (ME 2 Ch), mitral commissural (ME Mitral), and long axis (ME LAX) views. Additional information regarding LV and mitral valve function can be provided by the transgastric views: the transgastric basal and mid short axis (TG Basal SAX, TG Mid SAX) and transgastric two-chamber (TG 2 Ch) and transgastric long axis (TG LAX) views. Some patients can have significant LV outflow tract obstruction due to bowing of the ventricular septum that produces mid-cavitary obstruction, and this should also be evaluated carefully with a combination of mid esophageal (ME 4 Ch, ME LAX), transgastric (TG LAX), and deep transgastric long axis (DTG LAX) and deep transgastric sagittal (DTG Sagittal) views. The pulmonary valve, if present, can be evaluated and its annulus measured in the ME RV In-Out view, using a multiplane angle of 60–90° (Fig. 10.2b). The branch pulmonary arteries and ductus arteriosus are not always well seen, but ductal left to right flow can often be detected by color flow Doppler in the UE PA LAX and UE Ao Arch SAX views, using leftward probe rotation (when there is a left aortic arch).
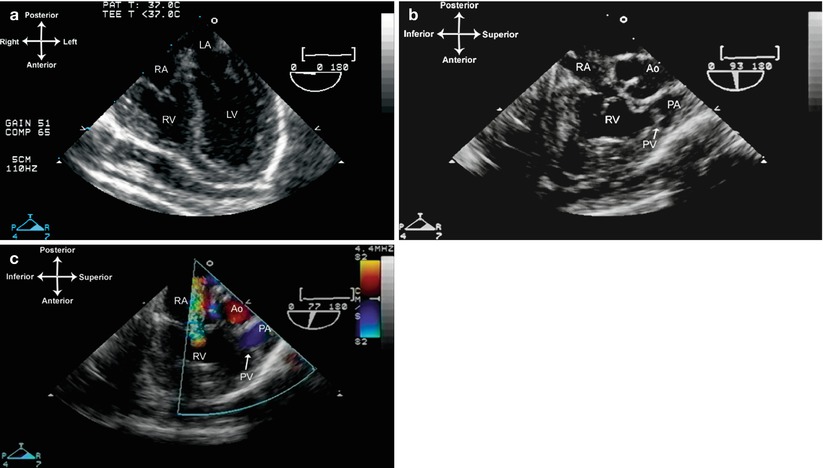
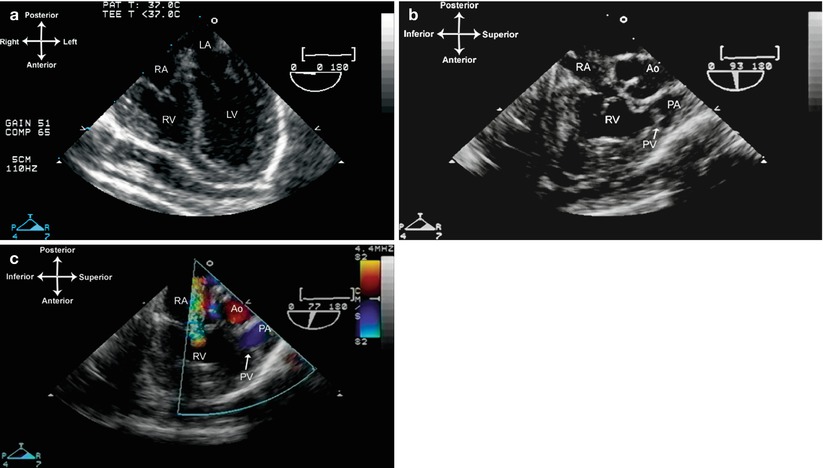
Fig. 10.2
Pulmonary atresia/intact ventricular septum, also known as hypoplastic right heart. The right ventricular cavity is small, muscular, and non-apex forming as displayed in the mid esophageal four chamber view (a). The trabecular portion of the right ventricle is typically underdeveloped, as shown in the mid esophageal right ventricular inflow-outflow views (b, c). Note the presence of an imperforate pulmonary valve without antegrade flow. There is significant tricuspid insufficiency. Ao ascending aorta, LA left atrium, LV left ventricle, PA main pulmonary artery, PV pulmonary valve, RA right atrium, RV right ventricle
Tricuspid Atresia
Patients with tricuspid atresia have no functional tricuspid valve. Often, there is complete absence of the tricuspid valve, with only a muscular shelf seen in the tricuspid position. In some instances the tricuspid valve is formed and present but imperforate (so-called “membranous” tricuspid atresia). In either case, there is obligate right to left shunting across the atrial septum, and complete mixing of deoxygenated and oxygenated blood at atrial level. There is generally a small RV cavity, with marked hypoplasia of the inflow portion but variable trabecular and infundibular portions, and usually (but not always) a communication between the LV and the hypoplastic RV. This communication has variously been termed a ventricular septal defect (VSD), or alternatively a bulboventricular foramen (BVF) [21, 22]—it generally is located between the LV and RV inflow portion, but can also be seen closer to the apex or infundibulum of the small RV. In the majority of cases, this defect will be the site of obstruction to LV to RV shunting, though obstruction can occur at the outlet valve itself (the valve arising from the RV) or in the subvalvar infundibulum [21]. The ventriculo-arterial connection can vary, and one of the most recognized classification systems for tricuspid atresia is based upon the relationship of the great arteries [23]. A summary of this classification system is given in Table 10.1. In general, this system’s nomenclature is not widely used when clinicians describe and discuss tricuspid atresia, but it still serves to underline the most important anatomic features relevant to this anomaly, namely (a) great artery origin/relationship, (b) VSD/BVF size, and (c) whether aortic or pulmonary outflow obstruction is present. In Type I, seen in 70–80 % of patients with tricuspid atresia [24], the aorta arises in normal position from the LV, there is ventriculo-arterial concordance, and the aortic valve is of normal size and function. The pulmonary artery arises from the small RV chamber, and the amount of pulmonary blood flow depends upon the size of the VSD/BVF and the degree of subpulmonary stenosis (if present). Type I can be further classified into three subtypes, based upon the size of the VSD and degree of pulmonary outflow obstruction: Type Ia (no VSD, pulmonary atresia), Type Ib (small VSD, pulmonary stenosis); Type Ic (large VSD, no pulmonary stenosis). If the ventricular defect is large and there is no subpulmonary stenosis, the patient can have excessive pulmonary blood flow leading to pulmonary overcirculation and congestion. If there is obstruction at the ventricular defect or subpulmonary level, there can be reduced antegrade flow leading to systemic arterial hypoxemia (Fig. 10.3, Video 10.3). The patient is then dependent upon an alternative source of pulmonary blood flow such as a patent ductus arteriosus or surgically created shunt. With Type II, seen in 12–25 % of patients with tricuspid atresia [24], there are transposed great arteries (ventriculo-arterial discordance), and the aorta arises from the small RV outflow chamber, generally to the right of the pulmonary artery, or D-transposed. Type II is also classified into three subgroups, based on the degree of pulmonary outflow obstruction: Type IIa (pulmonary atresia), Type IIb (pulmonary stenosis), Type IIc (no pulmonary stenosis). In general, with Type II tricuspid atresia there is either subaortic stenosis or subpulmonary stenosis, depending on the size of the VSD/BVF (through which blood must pass from LV to RV to aorta) and position of the infundibular septum. Type IIc is the most common form: in such cases the pulmonary valve (which arises from the LV) is usually of normal size and function. The ventricular defect is often surrounded by a muscular rim, and if small enough, there can be marked subaortic stenosis, often in association with hypoplasia of the aorta and aortic coarctation or interruption (in many cases requiring a patent ductus arteriosus for systemic perfusion). Such patients invariably require surgery to alleviate or bypass the subaortic stenosis, as well as repair the aortic arch. Conversely, Types IIa and IIb (which are much less common) have pulmonary outflow obstruction, usually due to posterior malalignment of infundibular septum that can produce significant subpulmonary stenosis and decreased pulmonary blood flow [25]. Such patients might require an alternative source of pulmonary blood flow, such as a ductus arteriosus or surgically created shunt. In these instances the VSD/BVF and aorta are generally of good size, and there is no obstruction to systemic blood flow. Types I and II are by far the most common forms of tricuspid atresia. Type III is much more rare, seen in about 3–6 % of patients with tricuspid atresia, and it is a less specific category that includes a number of other defects such as L-transposition of the great arteries, truncus arteriosus, etc. [24].
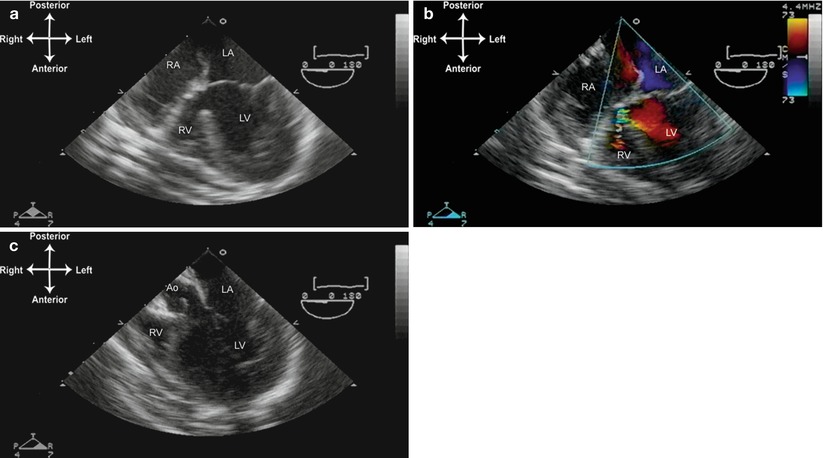
Table 10.1
Classification of tricuspid atresia
Type I | Normally related great arteries |
Ia | Intact ventricular septum and pulmonary atresia |
Ib | Small ventricular septal defect and pulmonary stenosis |
Ic | Large ventricular septal defect, no pulmonary stenosis |
Type II | Transposed great arteries |
IIa | Ventricular septal defect with pulmonary atresia |
IIb | Ventricular septal defect with pulmonary stenosis |
IIc | Ventricular septal defect, no pulmonary stenosis |
Type III | Transposition or malposition of the great arteries |
Associated complex lesions, including L-transpositionof the great arteries, double outlet right ventricle,double outlet left ventricle, truncus arteriosus |
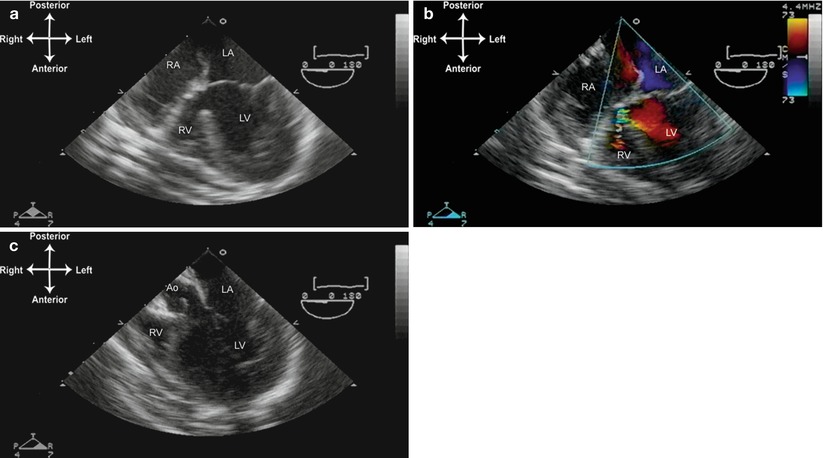
Fig. 10.3
Tricuspid atresia, normally related great arteries. (a) The mid esophageal four chamber view demonstrating lack of visible tricuspid valve; only a muscular shelf is seen, as well as a hypoplastic right ventricular chamber. (b) There is a small ventricular septal defect or bulboventricular foramen with turbulent left to right shunting. (c) Withdrawal and slight anteflexion of the TEE probe demonstrates the aortic valve (Ao) arising from the left ventricle. LA left atrium, LV left ventricle, RA right atrium, RV right ventricle.
The TEE evaluation of tricuspid atresia focuses upon atrial septal patency and shunting, mitral valve and LV function, the position of the great arteries and ventriculo-arterial relationship, and outflow tract patency including the size of the VSD/BVF. Much of this evaluation can be performed from the level of the mid esophagus, particularly the ME 4 Ch view for evaluation of atrial septum, mitral valve, LV and great arteries as well as VSD/BVF. Supplemental evaluation of these structures can also be provided by other mid esophageal views: ME Bicaval (systemic venous return, atrial septum), ME LAX (mitral valve, semilunar valves, VSD/BVF), ME RV In-Out (VSD/BVF). The deep transgastric views (DTG LAX, DTG Sagittal) can also provide good visualization of the mitral valve and VSD/BVF, as well as the aortic and pulmonary outflow tracts. The DTG views are also useful for spectral Doppler evaluation of the outflow tracts. In patients with transposed great arteries, the size of the ventricular defect, and potential for subaortic stenosis, should be evaluated carefully (Fig. 10.4, Video 10.4). If the VSD/BVF and/or the subaortic area are felt to be potentially obstructive, the pulmonary valve should be evaluated with the anticipation of a possible Damus-Kaye-Stansel (DKS) operation. The evaluation of the VSD/BVF and the DKS operation are discussed below. In tricuspid atresia patients with transposed great arteries, left juxtaposition of the atrial appendages can sometimes be encountered [26, 27]. Care should be taken to recognize this entity and not confuse it with a large atrial septal defect; in fact, the orientation of the true atrial septal defect is often different from what is normally encountered [28]. This entity is best evaluated from a modified ME AV SAX view first retroflexed, then slowly anteflexed to demonstrate the right atrial appendage just anterior to the left atrial appendage. An example of left juxtaposition of the atrial appendages is given in Chap. 12—Conotruncal Malformations.
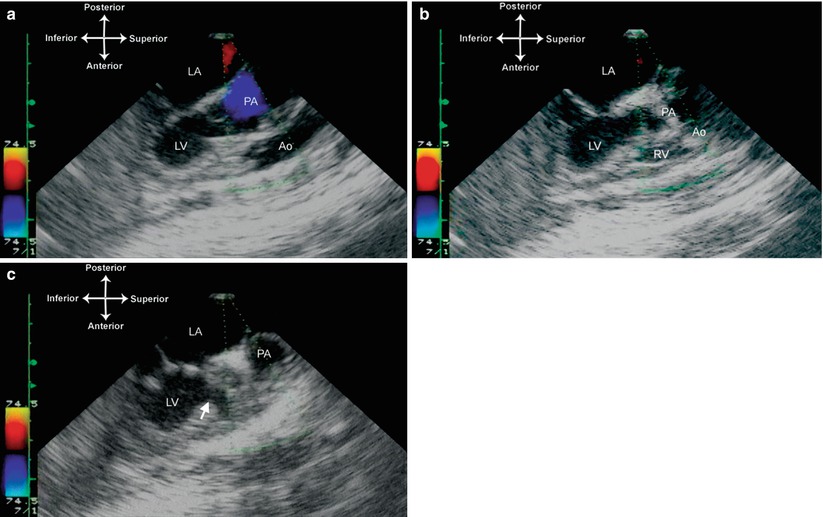
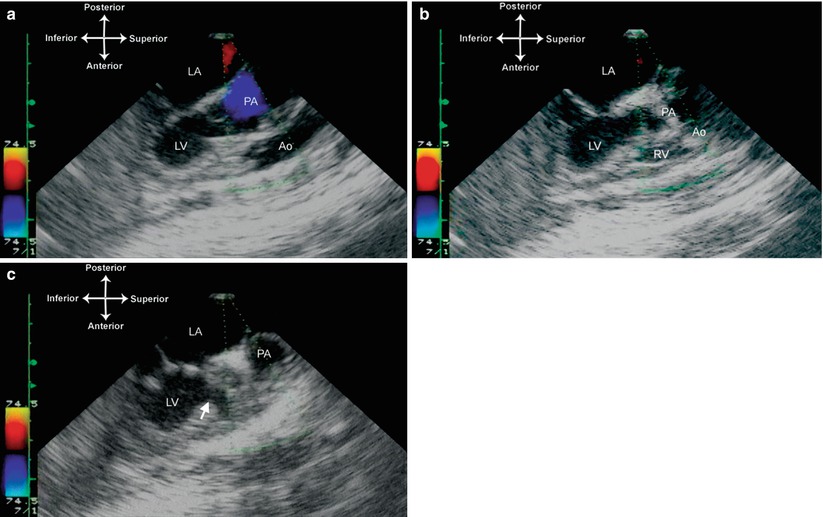
Fig. 10.4
Tricuspid atresia with D-transposed great arteries. Images taken from the mid esophageal aortic valve long axis view, multiplane angle 90°, showing the relationship of the great arteries with the pulmonary artery arising from the left ventricle, and the aorta arising from a small anterior right ventricular outflow chamber (a). The ventricular septal defect (bulboventricular foramen) is noted by the arrow (b). LA left atrium, LV left ventricle, PA pulmonary artery, Ao Aorta
In tricuspid atresia patients with normally related great arteries, the size of the interventricular communication and determination of the presence and degree of pulmonary outflow tract obstruction are essential. If pulmonary blood flow is unobstructed, limitation by means of pulmonary artery banding is usually undertaken; if blood flow is reduced, interventions to increase pulmonary blood flow will need to be considered. Some patients have just enough restriction to pulmonary blood flow that they are neither overcirculated nor excessively cyanotic—in other words, they are well balanced. Such patients might not require any intervention for the first few months of their life, although ultimately they will require the same surgeries used for all single ventricle patients—namely, the Glenn and Fontan procedures (discussed below).
Univentricular Atrioventricular (AV) Connection
This category of single ventricle includes those defects in which both atria empty completely (or almost completely) into one large ventricle. A second ventricle might or might not be present; if present, it is generally quite hypoplastic. This group of defects includes unbalanced AV septal defect, double inlet ventricle (DILV), as well as the rare double inlet right ventricle (DIRV) and true single (or solitary) ventricular chamber of indeterminate morphology.
Patients with unbalanced AV septal defects have similar characteristics to a large AV septal defect (Chap. 8—AV septal defects and AV valve anomalies), but the common AV valve—instead of being balanced equally over both ventricles—is oriented significantly toward one ventricle. Thus, both atria empty primarily or almost completely into that chamber, and there is usually some degree of hypoplasia of the other ventricle (Fig. 10.5). In some patients, the degree of AV valve unbalance is so pronounced, and/or the degree of ventricular hypoplasia so apparent, that it is clear that the defect cannot be septated — i.e. the patient must be treated as a functional single ventricle. In others, this assessment is more difficult, and various criteria are utilized to determine whether a biventricular repair is feasible [29, 30]. Unbalanced AV septal defect is a common accompanying feature of heterotaxy syndrome (discussed below). The TEE evaluation of such patients is very much the same as for any patient with an AV septal defect, with particular attention paid to the degree of common AV valve distribution or commitment to the underlying ventricular chambers, AV valve function, ventricular hypoplasia, and possible aortic or pulmonary outflow tract obstruction. A combination of mid esophageal views (ME 4 Ch, ME 2 Ch, ME LAX) are best for evaluating the common AV valve and ventricular chambers, and also the degree of AV valve regurgitation (if present). When available, the deep transgastric views (DTG LAX, DTG Sagittal) can be used to evaluate the AV valve en face and its balance over both ventricles, similar to the views obtained with a subcostal sagittal view [29, 31]. The TEE evaluation of AV septal defects is discussed extensively in Chap. 8.
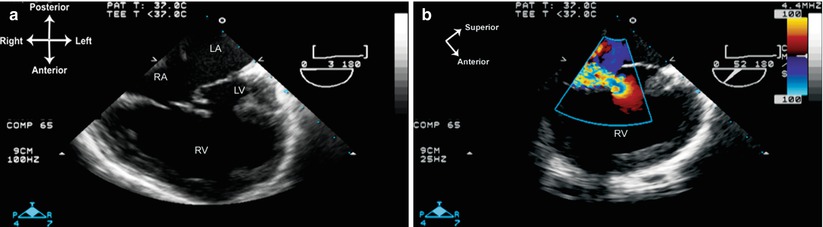
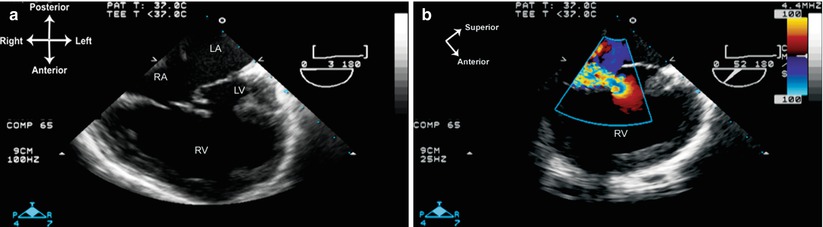
Fig. 10.5
Unbalanced atrioventricular (AV) septal defect. (a) Mid esophageal four chamber view demonstrates an extremely right dominant AV septal defect with a tiny left ventricle. The large common AV valve empties almost completely into the large right ventricle. (b) Significant central AV valve regurgitation as documented with a multiplane angle of 52°. LA left atrium, LV left ventricle, RA right atrium, RV right ventricle
Double inlet ventricles are characterized by both atria and AV valves emptying into a large ventricle of either left or right ventricle morphology. The vast majority of these are DILV: both AV valves empty into a large ventricle of LV morphology, and there is generally a smaller RV outflow chamber located anteriorly. In many cases of DILV, it is very difficult to determine which AV valve is tricuspid, and which is mitral. There are three general classes of DILV: (1) normally related great arteries; (2) D-transposed great arteries; (3) L-transposed great arteries. The patient with DILV and normally related great arteries, also known as a Holmes’ heart [32], has a normal aortic valve and aorta arising from the large LV, with the pulmonary artery arising from the RV outflow chamber with a variable degree of subpulmonary stenosis (Fig. 10.6, Video 10.5). The patient with DILV and D-transposed great arteries has the aorta arising from a rightward, RV outflow chamber; with L-transposed great arteries the aorta arises from a leftward RV outflow chamber. Of the three types of DILV, the L-transposed is the most common type (60–65 % of all DILV), followed by the D-transposed form (20–25 %), and lastly the normally related great arteries variation (15 %). In both forms of DILV with transposed great arteries, there is either significant subaortic narrowing (often with ascending aorta and aortic arch hypoplasia) or subpulmonary narrowing, depending on the size of the VSD/BVF and position of the infundibular septum [1, 33]. This is analogous to tricuspid atresia and transposed great arteries, as described above.
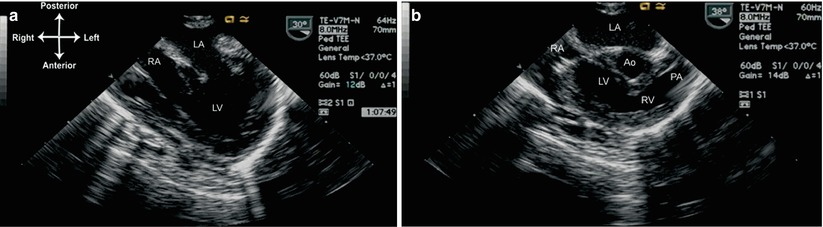
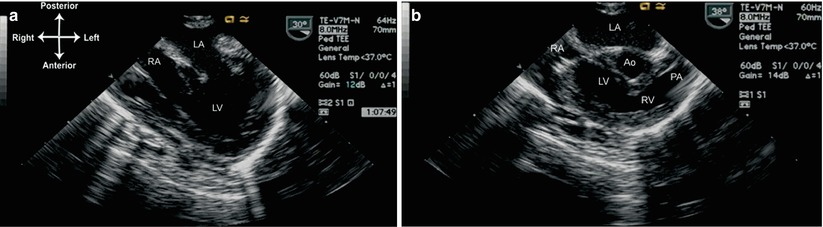
Fig. 10.6
Double inlet left ventricle, normally related great arteries (Holmes’ heart) as viewed from the mid esophageal four chamber view. (a) A multiplane angle of 30° is used to visualize both atrioventricular valves. (b) Rotation of multiplane angle to approximately 40° and probe tip anteflexion demonstrate the origin of the pulmonary artery from the hypoplastic right ventricular outflow chamber (RV). Ao aorta, LA left atrium, LV left ventricle, PA pulmonary artery, RA right atrium
DIRV is a rare defect in which both AV valves empty into a chamber of RV morphology [34]. In these instances, there are prominent muscle bundles in the large RV chamber; occasionally a large muscle bar is present that can be confused for a remnant of ventricular septum [35]. Usually, both great arteries also emerge from the same large RV, thus this lesion is often termed a double inlet, double outlet RV [35]. However unlike DILV, no anterior outflow chamber is visible. Instead, a rudimentary LV chamber may or may not be present; when present, it is located posteriorly, with no outflow to either great artery (Fig. 10.7, Video 10.6). Varying degrees of obstruction to one of the great arteries (generally the pulmonary trunk) can also be seen [36].
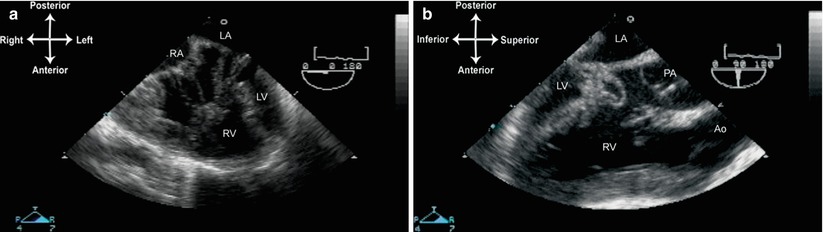
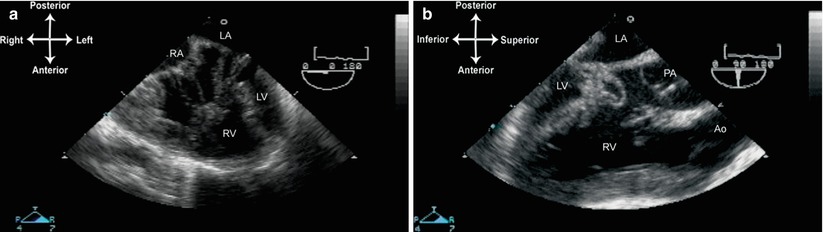
Fig. 10.7
Double inlet, double outlet right ventricle. (a) This image is obtained from the mid esophageal four chamber view. Both right and left atrioventricular valves drain into the large right ventricle (RV), inserting into a large, bizarre RV papillary muscle. There is a hypoplastic left sided left ventricle (LV). (b) Equivalent of the mid esophageal aortic valve long axis (90°) view. Both great arteries are seen to arise from the RV, with the aorta anterior to pulmonary artery. Ao aorta, LA left atrium, PA pulmonary artery, RA right atrium
In the true single ventricle, there is no visible second chamber, and the large solitary ventricle has a very primitive morphology that eludes definitive identification as either an RV or LV [4, 33, 37]. There can be either one or two AV valves emptying into the large chamber, or there can even be one large common AV valve (i.e. a large common AV canal). Often, the heart has a very primitive appearance, with bizarre and loose muscular trabeculations seen in the large ventricular cavity. The great arteries have a variable relationship, generally with either stenosis of the aortic or pulmonary artery outflow tracts. There is a higher prevalence of atrial isomerism with indeterminate ventricular morphology [38].
Evaluation of the univentricular heart by TEE is similar to the evaluation of tricuspid atresia. The views of the inflows are best obtained beginning with the lower esophageal situs short axis (LE Situs SAX), first using a multiplane angle of 0–20°, then withdrawing the probe to visualize the junction between IVC and right atrium. Further withdrawal of the probe to the ME 4 Ch view demonstrates the AV valve(s) by imaging. Both color flow and spectral Doppler are necessary to evaluate AV valve function. When two AV valves are present, if either AV valve is stenotic or atretic, patency of the atrial septum must be assessed both by imaging and color flow Doppler. The relationship of the great arteries must also be determined; if one of these arises from a smaller outflow chamber, the VSD/BVF size and patency also need to be evaluated, preferably from several multiplane angles between 0 and 90°. Any subpulmonary or subaortic stenosis can be well visualized from a ME RV In-Out, ME AV LAX, and ME LAX views, multiplane angles between 70° and 120°, optimized to yield the best longitudinal view of the outflow tract (Fig. 10.8, Video 10.7). When available, the deep transgastric windows (DTG LAX, DTG Sagittal) provide good visualization of a number of structures, including the VSD/BVF, as well as excellent angles of Doppler interrogation for gradients across either the aortic or pulmonary outflow tracts (Fig. 10.9).
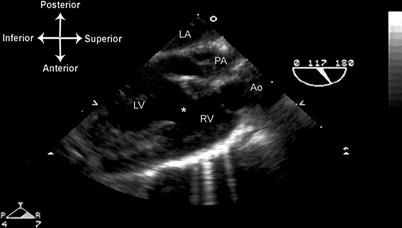
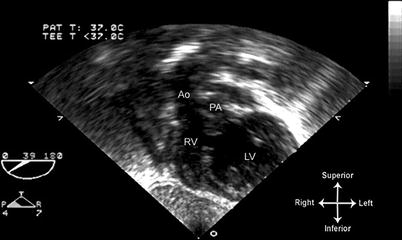
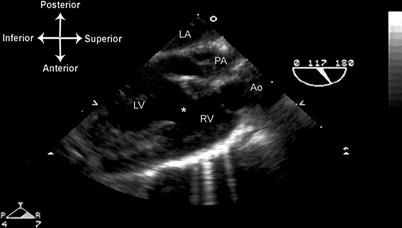
Fig. 10.8
Double inlet left ventricle, D-transposed great arteries, following pulmonary artery band placement. The mid esophageal aortic valve long axis view, multiplane angle of 117°, optimally demonstrates the long axis of both outflow tracts, and also shows the ventricular septal defect/bulboventricular foramen . This view also captures the left atrium and both ventricles in the same cross-sectional plane as the two outflow tracts. Ao aorta, LA left atrium, LV left ventricle, PA pulmonary artery, RV right ventricle
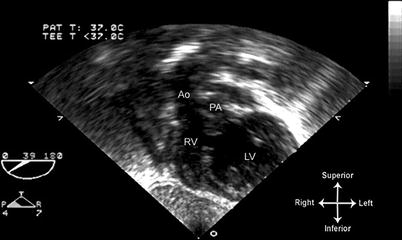
Fig. 10.9
Image from same patient as depicted in Fig. 10.8 obtained from a deep transgastric long axis view, multiplane angle of 39°. Both outflow tracts are displayed. This view offers an excellent angle for spectral Doppler evaluation of the outflow tracts. Ao aorta, LV left ventricle, PA pulmonary artery, RV right ventricle
As with tricuspid atresia, left juxtaposition of the atrial appendages is also associated with DILV and D-transposed great arteries, and care should be taken not to confuse this entity with a large atrial septal defect.
Heterotaxy
Despite an external appearance of right-left symmetry, much of the human body’s internal organ layout is in fact asymmetric, and this asymmetry leads to a definition of normal (and abnormal) sidedness. In situs solitus (which typifies most humans), the liver and IVC are on the right, the stomach and aorta on the left. The heart, being an asymmetric organ, also lateralizes to lie in the left chest. In situs inversus, the organs lateralize to the opposite side, producing a “mirror image” layout of the heart, lungs, and abdominal viscera. The term heterotaxy denotes an abnormality of laterality, or sidedness, in which there is (paradoxically) bilateral visceroatrial symmetry. Hence this condition is known as situs ambiguous or atrial isomerism and involves multiple organ systems in the body, including the heart. From a genetic standpoint, it is now known that heterotaxy belongs to a class of abnormalities known as “ciliopathies”, characterized by abnormalities or dysfunction of primary cilia. During cardiac embryogenesis, primary cilia on the embryonic node are required for generation of the left-right axis. Ciliary dysfunction abnormalities are responsible for disorders of left-right axis determination during early embryonic development. This results in a disorder of laterality, manifested by abnormalities of any internal organ that is asymmetrically position—including the heart. Significant phenotypic variation can result [39, 40].
Patients with heterotaxy generally have significant cardiac abnormalities, often affecting multiple segments of the heart [41–43]. However despite the myriad potential variations in cardiac anatomy, heterotaxy patients tend to fall into one of two categories: (1) right isomerism (bilateral right-sidedness), or asplenia; (2) left isomerism (bilateral left-sidedness), or polysplenia. The visceral and cardiac variants most commonly seen with each category are listed in Table 10.2. It is important to note, however, that this table lists only the most commonly associated features seen with each category, and a number of exceptions have been reported, as well as considerable overlap between the two categories.
Table 10.2
Right vs. left isomerism: common anatomic features
Right isomerism (asplenia) | Left isomerism (polysplenia) | |
---|---|---|
Liver | Midline, symmetric | Asymmetric or symmetric |
Lungs | Bilateral tri-lobe | Bilateral bi-lobe |
Eparterial bronchi | Hyparterial bronchi | |
Spleen | Usually absent, but rarely can be multiple | Usually multiple, but can be only one or absent |
Cardiac position | Dextrocardia (36–41 %) | Dextrocardia (33–42 %) |
Systemic venous drainage | Bilateral SVCs are common | Interrupted hepatic segment of IVC; azygous or hemiazygous continuation to right or left SVC |
Coronary sinus usually unroofed | One or both SVCs can be present; if two SVCs, one generally drains into a coronary sinus | |
IVC usually present; IVC and hepatic veinsreturn together to common atrium | Separate hepatic drainage to atrium | |
There can be separate drainage of hepatic veins | ||
Pulmonaryvenous drainage | Anomalous pulmonary venous drainage to systemic vein (62–88 %) | Usually to back of common atrium; abnormal position of atrial septum results in right veins to right side, left veins to left side |
Can return directly to heart, often via a common collector | ||
Atria and atrial septum | Bilatral right atrial appendages | Bilateral left atrial appendages |
Usually common atrium; small remainingatrial septal remnants | Usually common atrium; if atrial septum present, usually primum atrial septal defects | |
AV valve | Large common AV valve, often RV dominant | Two AV valves (common AV valve can also be seen) |
Ventricles | Usually large RV, hypoplastic LV | Can be two balanced ventricles or one dominant ventricle |
Ventricularoutflow tracts | Often subvalvar/valvar pulmonary stenosis or atresia (88–96 %) | Valvar/subvalvar pulmonary stenosis (about 40 %) and aortic obstruction/atresia (17–22 %) |
Rarely, aortic stenosis/atresia/coarctation | ||
Ventriculo-arterialrelationships | DORV (82 %)/TGA (9 %); often subvalvar/valvar pulmonary stenosis or atresia (88–96 %) | Normally related great arteries (50–84 %); DORV or ventriculo-arterial discordance (16–37 %); |
Rarely, aortic stenosis/atresia/coarctation |
For most heterotaxy patients, especially those with right isomerism (asplenia), the preponderance of cardiac abnormalities—particularly hypoplasia of one ventricle—dictates that a single ventricle pathway be chosen [44, 45]. However in a small percentage of patients (mainly those with left isomerism, or polysplenia), a two ventricle approach can be contemplated [46, 47]. Therefore when a TEE evaluation of either right or left isomerism is performed, a very careful and comprehensive evaluation is recommended in consideration of the implications regarding patient management. Because of potential for anatomic abnormalities at multiple levels, a complete, segmental examination (Chap. 4) should be performed, particularly in those patients with unknown or uncertain anatomy. Using the many available TEE views discussed in Chap. 4 as well as this chapter, careful attention should be paid to systemic and pulmonary venous drainage, number and function of the AV valves, ventricular size and function, great artery position, and possible outflow tract stenosis. In the intraoperative setting, the examination also needs to be tailored to the important surgical questions that must be answered for the proposed surgery. For example, if Fontan surgery is contemplated, evaluation of systemic and pulmonary venous drainage is paramount (Fig. 10.10, Video 10.8)
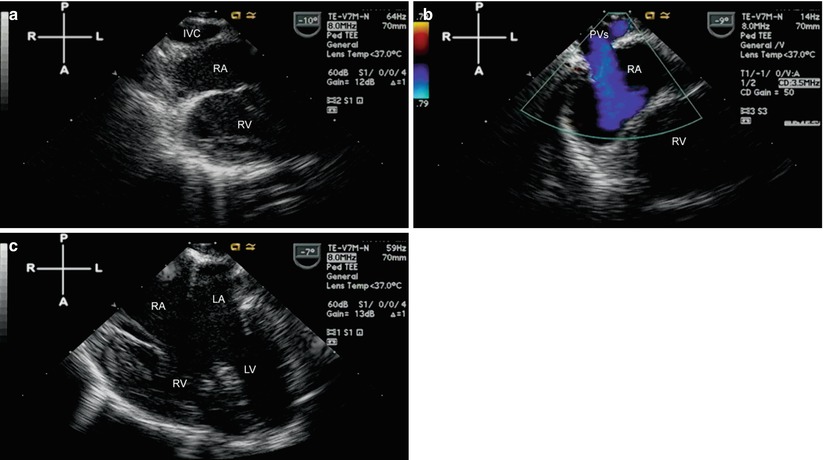
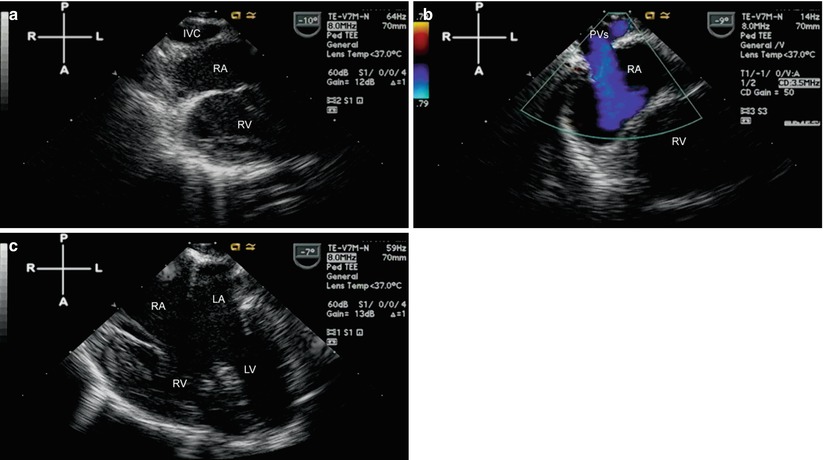
Fig. 10.10
Pre-Fontan study in a patient with right isomerism (asplenia) and double outlet right ventricle/pulmonary atresia. (a) Is obtained using multiplane angle 0° after withdrawal of the TEE probe from a lower to mid esophageal position. The inferior vena cava (IVC) receives the right hepatic veins and returns to the right atrium. In (b) the pulmonary veins are shown as they also return to the right sided atrium. (c) Demonstrates the presence of an unbalanced atrioventricular septal defect, right dominant. LA left atrium, LV left ventricle, PVs pulmonary veins, RA right atrium, RV right ventricle
Other Types of Single Ventricle
There are a number of other forms of complex congenital heart disease that—for one reason or another—fall under the “single ventricle” umbrella, even if they have two AV valves and two ventricles. In some patients, both ventricles are well-formed but other intracardiac features make a two ventricle repair unfeasible or undesirable, and therefore these patients are placed on a single ventricle pathway. Such complicating features include straddling/overriding AV valves, or double outlet RV with a VSD that is remote from either semilunar valve and makes two ventricle repair a high-risk proposition [48–50]. There are also some rare, complex cardiac defects that historically do not lend themselves well to a two ventricle repair. These include some forms of double outlet LV [51], superior-inferior ventricles with criss-cross AV relations [52, 53], etc. In many of these cases, there exist significant intracardiac abnormalities in addition to right or left ventricular hypoplasia.
When TEE is performed in patients with one of these complex cardiac lesions, a complete evaluation should be performed, with specific attention paid to AV valve size and orientation, ventricular chamber size and location of ventricular septal defect(s), and great artery relationships. In some cases, information from the TEE will be utilized to render an assessment as to whether a two ventricle repair is feasible. Currently, the bias for most clinicians caring for CHD patients is to perform a two ventricle repair whenever possible. However, the question as to whether a good one ventricle repair is better than a marginal two ventricle repair remains unanswered and is, in many instances, controversial [54–56].
Surgery for the Single Ventricle
With a single ventricle and single ventricle physiology, there is only one functioning pump that must serve both systemic and pulmonary circulations. Because of this, obligatory mixing of systemic and pulmonary blood flow occurs, resulting in systemic arterial hypoxemia. The eventual goal of surgical management of the single ventricle is to restore normal systemic arterial oxygenation by separating the circulations; this is performed by rerouting systemic venous (deoxygenated) blood directly into the pulmonary arteries, thereby bypassing the heart. Deoxygenated blood is expected to flow directly to the pulmonary arteries without the aid of a cardiac pumping chamber [57]. This is known as the Fontan procedure, which is named and based upon the original surgery described in 1971 by Fontan and Baudet for treatment of tricuspid atresia [58]. Over the past 40+ years the Fontan procedure has undergone a number of modifications and improvements, and has gained widespread acceptance as the preferred surgical management strategy for all forms of single ventricle [59]. It has been clearly established that, even without the aid of a right-sided pumping chamber, Fontan physiology is compatible with long-term survival and good functional status [60]. There are still a number of ongoing questions regarding long-term morbidity (arrhythmias, protein-losing enteropathy, liver dysfunction, ventricular dysfunction, plastic bronchitis, etc.) and survivability into late adulthood; these topics are beyond the scope of the current chapter but are fully discussed in many other references [59, 61–64].
While the ultimate goal for all single ventricle patients is the Fontan procedure and achievement of Fontan physiology, the complete Fontan operation cannot be performed in neonates or young infants because of a still immature pulmonary vascular bed, and still developing neonatal myocardium. The ideal timing for the Fontan procedure is generally felt to be after 2–3 years of age [65, 66]. Therefore, assuming single ventricle patients are correctly diagnosed in early infancy, almost all will require one or more staging surgeries prior to the Fontan procedure. The purpose of these staging surgeries is to ensure that patients are optimally prepared for their eventual Fontan procedure. For successful Fontan physiology, certain key anatomic and physiologic factors should ideally be present, including adequate size pulmonary arteries, low pulmonary arteriolar resistance, no more than mild AV valve regurgitation, and normal ventricular systolic and diastolic function [65, 66]. A full discussion of these important factors can be found in many references [59, 63, 67–70].
Different institutions utilize TEE to varying degrees for the various surgical procedures performed as part of single ventricle surgical pathway. Nonetheless, TEE can provide useful and important information. Preoperative evaluation of single ventricle patients by TEE is generally excellent due to its superior imaging capabilities. However, most preoperative single ventricle patients will have already undergone extensive imaging by several different modalities, hence TEE is used primarily as a complementary imaging technique, e.g. when assessment of AV valve function is desired in a larger patient with poor transthoracic imaging. For postoperative assessment, TEE can play a more prominent role. It is well known that postoperative single ventricle patients, particularly Fontan patients, are notoriously difficult to image by transthoracic echocardiography. Therefore TEE can provide a much greater contribution in the assessment of such patients, most notably in the immediate postoperative period. The following section discusses the important preoperative and postoperative features that can and should be assessed by TEE, whenever it is performed in single ventricle patients.
Preoperative Assessment
The preceding sections of this chapter detailed individual defects considered part of the single ventricle spectrum, including specific anatomic characteristics that should be evaluated. While considerable anatomic heterogeneity exists among the different classes of defects, what becomes evident is that certain common anatomic and functional characteristics must be explored whenever a TEE is performed for preoperative assessment. These characteristics are relevant not only for the patient’s current clinical condition, but also for any future palliative surgeries that might be contemplated. The list of potential surgeries for single ventricle palliation is limited (as will be discussed below), thus certain key anatomic features carry great weight—and therefore assume particular relevance—in the preoperative evaluation of these patients. Some of the features were mentioned previously for the individual cardiac lesions, but the following list summarizes the essential elements that should be addressed during preoperative assessment of all such patients:
Systemic venous return. The goal of single ventricle palliation is the return of all systemic venous blood directly to the pulmonary arteries. Thus, detailed knowledge of systemic venous return is essential. Although in most cases systemic venous drainage will have been adequately delineated prior to surgery, in many instances TEE can provide helpful confirmatory information. The presence/absence of the hepatic portion of IVC and the drainage of the hepatic veins should be verified. In some patients, particularly those with visceral heterotaxy, some hepatic veins can drain separately from the IVC and directly into the atrium (Fig. 10.11, Video 10.9). In others, particularly those patients with polysplenia (left isomerism), the hepatic portion of the IVC can be absent (interrupted IVC with azygos continuation) and there can be separate entrances of right and left hepatic veins into the atria. Advancing the TEE probe to the lower esophageal position (LE Situs SAX, multiplane angle 0–20°), with careful withdrawal and clockwise/counterclockwise turning of the TEE probe, can demonstrate the sites of IVC and hepatic vein drainage. Identification of the SVC drainage is also very important: both the number of SVCs (one vs. two), and their sites of drainage, should be determined. This can be performed by withdrawing the TEE probe to the mid/upper esophagus, including the mid esophageal ascending aortic short axis (ME Asc Ao SAX) and UE PA LAX views (both using a multiplane angle 0°), and rotating clockwise and counterclockwise. It is important to search directly for a separate right and left SVC and not rely upon the presence/absence of a dilated coronary sinus, because the coronary sinus could be unroofed or absent, allowing left SVC return directly to the atrium. Sagittal views of both SVCs can then be obtained in the mid to upper esophageal position, multiplane angle at approximately 80–90°, using a combination of views including ME Bicaval, mid esophageal ascending aortic long axis (ME Asc Ao LAX) with rightward rotation to visualize a right SVC, and ME 2 Ch, ME Asc Ao LAX, and UE Ao Arch (all with leftward rotation) to visualize a left SVC. With these sagittal views, azygos or hemiazygos drainage to the SVC can sometimes be seen coursing posteriorly and over the corresponding branch pulmonary artery.
Fig. 10.11
Image from patient in Fig. 10.10. The left sided hepatic veins return separately to the left sided atrium (a). The entrance of these veins into the left atrium is marked by an asterisk (b). HVs left hepatic veins, LA left atrium, LV left ventricle, RA right atrium, RV right ventricle
Pulmonary venous return. In general, pulmonary venous drainage will have been established with imaging studies performed prior to the TEE. Nonetheless, in some single ventricle patients the drainage of the pulmonary veins might not be completely clear. The role of the preoperative TEE is to confirm the drainage of all pulmonary veins, and establish that this drainage is unobstructed. Because of the close proximity of the esophagus to the atria, TEE generally provides excellent visualization of pulmonary venous return, both for imaging and color flow/spectral Doppler evaluation (Chaps. 4 and 6). Any obstruction to pulmonary venous flow must be noted, because it will have negative consequences for further single ventricle palliation [71, 72]. The relationship of the pulmonary venous return to the systemic veins should also be noted, as the placement of a Fontan conduit could potentially interfere with pulmonary venous return, and surgical adjustments might be required [73].
Patency of the atrial septum. An unrestrictive atrial septal defect is essential in those patients in whom systemic or pulmonary venous return empties into an atrial chamber associated with a stenotic or absent AV valve, or a hypoplastic/nonfunctional ventricular chamber (e.g. tricuspid atresia or HLHS). In such cases, the only pathway for egress of blood from the chamber is through the atrial communication. Using TEE, this can be evaluated both by imaging as well as color flow/spectral Doppler interrogation. Midesophageal views with a multiplane angle of 0–90° (ME 4 Ch, ME Bicaval) can be used, combined with other probe maneuvers such as advancement/withdrawal and left/right rotation. There are two types of restrictive atrial communication: with left to right shunting, and with right to left shunting. A restrictive left to right atrial communication is found in those defects in which there is left sided obstruction, such as HLHS and DILV with left AV valve stenosis/atresia. In these cases, atrial restriction is characterized by a number of findings, including: (a) small size (diameter <3 mm); (b) color flow Doppler aliasing of the atrial shunt and a mean gradient >5 mmHg [74]; (c) dilated pulmonary veins, along with prominent flow reversal in these structures; (e) dilated left atrium, with an atrial septum bowing toward the right (although a large left atrium does not always indicate a restrictive atrial communication); (f) in HLHS patients, a small left atrium with thick atrial septum [75]. The incidence of atrial septal restriction in HLHS patients is estimated between 6 and 20 % [75–78]. When the atrial septal communication is felt to be restrictive, it often requires enlargement, either by interventional cardiology procedures such as balloon dilation/stenting of the septum, or an atrial septectomy at the time of surgery. In HLHS patients with a restrictive atrial septal defect, emergent atrial septostomy is sometimes necessary, though mortality is much higher in this subset of patients [76, 78, 79]. This has led to development of in utero atrial septoplasty in HLHS patients prenatally diagnosed as having a restrictive atrial septal defect [80].
A restrictive right to left atrial communication is found in those defects in which there is obstruction to right AV valve inflow, such as tricuspid atresia, hypoplastic right heart syndrome, or DILV with right AV valve stenosis/atresia. In these cases the restriction can be more challenging to determine; unlike HLHS and other left sided obstruction, the atrial septum is often redundant and it can be difficult to define accurately the margins of the atrial defect. Moreover, in some patients restriction might not be apparent immediately after birth, but can increase with time [81]. This type of atrial septal restriction occurs in 5–15 % of tricuspid atresia patients [82, 83]. Overall, less data is available in this group of patients. One study has shown that an atrial septal aneurysm (defined as bulging >6 mm beyond the plane of the atrial septum) and atrial septal defect diameter <5 mm (measured on the 2D echocardiographic image) places tricuspid atresia patients at higher risk for atrial septal restriction [83].
Atrioventricular valve (AV) function. The AV valve(s) should be examined structurally in terms of the leaflets, support apparatus, and annular size. The function of the valves should be assessed as well. This is accomplished by two-dimensional imaging as well as color flow mapping and spectral Doppler. In single ventricle patients, the presence and degree of AV valve regurgitation can have significant bearing on clinical outcomes [84]. In patients with two AV valves (e.g. double inlet LV), the function of both valves should be examined. If one valve is excessively regurgitant, and the other valve competent and of adequate size, the regurgitant valve can be repaired or even oversewn. If one AV valve is significantly stenotic or atretic, a large atrial defect is mandatory to allow atrial blood to pass unobstructed to the other AV valve (as noted above). For the TEE evaluation of the AV valves, the mid esophageal views (ME 4 Ch, ME 2 Ch, ME Mitral, ME LAX) are useful to evaluate valve morphology and function. Additional information regarding chordal and papillary muscle architecture can be provided by the transgastric views: the “short axis” transgastric views (TG Basal SAX, TG Mid SAX) and “long axis” transgastric views (TG 2 Ch, TG LAX, and transgastric RV inflow or TG RV In) views. Given the unusual orientation of the AV valve(s) in many forms of single ventricles, variation in multiplane angle between 0° and 120° in many of these views might be necessary to facilitate optimal visualization. In some instances the deep transgastric views (DTG LAX, DTG Sagittal) can be useful for en face evaluation of the AV valves.Stay updated, free articles. Join our Telegram channel
Full access? Get Clinical Tree
